Abstract
GABAergic control over dopamine (DA) neurons in the substantia nigra is crucial for determining firing rates and patterns. Although GABA activates both GABAA and GABAB receptors distributed throughout the somatodendritic tree, it is currently unclear how regional GABA receptors in the soma and dendritic compartments regulate spontaneous firing. Therefore, the objective of this study was to determine actions of regional GABA receptors on spontaneous firing in acutely dissociated DA neurons from the rat using patch-clamp and local GABA-uncaging techniques. Agonists and antagonists experiments showed that activation of either GABAA receptors or GABAB receptors in DA neurons is enough to completely abolish spontaneous firing. Local GABA-uncaging along the somatodendritic tree revealed that activation of regional GABA receptors limited within the soma, proximal, or distal dendritic region, can completely suppress spontaneous firing. However, activation of either GABAA or GABAB receptor equally suppressed spontaneous firing in the soma, whereas GABAB receptor inhibited spontaneous firing more strongly than GABAA receptor in the proximal and distal dendrites. These regional differences of GABA signals between the soma and dendritic compartments could contribute to our understanding of many diverse and complex actions of GABA in midbrain DA neurons.
Dopamine (DA) neurons in the substantia nigra pars compacta (SNc) as a pacemaker neuron generate action potential regularly. However, various synaptic and neuromodulatory events can affect them, generating diverse firing patterns including firing pauses and burst discharges [12]. Firing rate and patterns are critical in various DA actions related to many brain functions, including motor control, reward processing, reinforcement learning, and drug addiction [34]. Many excitatory and inhibitory neurotransmitters can influence firing activities of DA neurons. Gamma-aminobutyric acid (GABA), the most dominant inhibitory neurotransmitter, often suppresses spontaneous firing of DA neurons completely [567]. It is well known that striatum, globus pallidus, and substantia nigra pars reticulata project GABAergic afferents to SNc, and that almost 70% of these afferents to the SNc DA neurons are GABAergic [89]. Thus, GABAergic synapses might dominantly govern DA neuron activities. Disinhibition by removing background GABAA conductance can evoke burst firing in certain conditions [10] and in a DArgic model neuron [11]. On the other hand, it has been also reported that GABAB receptors are important for generating burst firings in DA neurons in vivo [12].
GABA activates two different types of receptors: GABAA and GABAB receptors. GABAA receptors are fast-acting ligand-gated chloride (Cl−) channels [1314], whereas GABAB receptors are slow-acting G-protein coupled receptors [1516]. Activation of ionotropic GABAA receptors hyperpolarizes membrane potential and inhibits firing activities of DA neurons [171819]. Metabotropic GABAB receptors can activate many downstream targets, including inwardly rectifying K+ channels, voltage-sensitive Ca2+ channels, and adenylyl cyclase [20]. Activation of K+ channels can generate slow inhibitory postsynaptic potentials (IPSPs) and reduce membrane excitability [21]. They can suppress postsynaptic responses [22] and limit action potential backpropagation [232425]. Local blockade of GABAB receptors in SNc DA neurons in vivo has shown ambiguous effects on firing rate, although it can increase firing regularity [726]. However, exact roles of GABA in nigral DA neurons are far from clear. There are many contradictory reports. For example, inhibitory responses of nigral DA neurons by in vivo stimulation of afferents from the striatum, globus pallidus, and substantia nigra pars reticulata are predominantly mediated by GABAA receptors, but nigral DA neurons in vitro express both functional GABAA and GABAB receptors [71226272829]. Therefore, it has been speculated that postsynaptic GABA induced by neural activity changes is dominantly mediated by GABAA receptors, while slow inhibition via GABAB receptor at presynaptic or perisynaptic sites might be involved when synaptic GABA overflows [71516303132]. However, in highly polarized DA neurons, little is known about where and how GABA receptors exactly regulate spontaneous firing in DA neurons.
Acutely isolated SNc DA neurons can be divided into the soma, proximal dendrite, and distal dendrite. They exhibit regular spontaneous firings [233]. By taking this advantage together with employment of local GABA-uncaging, we here report that GABA signals in the soma and dendrites can differently regulate spontaneous firing in SNc DA neurons of the rat. In the soma, GABA suppresses spontaneous firing equally through GABAA and GABAB receptors. However, in the proximal and distal dendrites, GABA suppresses firing in a GABAB receptor-dominant way.
Sprague-Dawley rats at 9 to 12 days old were subjected to decapitation. Their brains were quickly excised and placed in ‘high glucose solution’ containing 135 mM NaCl, 5 mM KCl, 10 mM HERPES, 1 mM CaCl2, 1 mM MgCl2 and 25 mM D-glucose. The pH was adjusted to 7.3 with NaOH. Midbrain coronal slices of 400 µm in thickness, containing SNc, were obtained using a vibratome (Series 100, St. Louis, MO, USA). Subsequently, SNc regions of slices demarcated by dark color were dissected out with a scalpel blade and placed in fully oxygenated HEPES-buffered saline containing papain (8 U/ml, Worthington) and incubated at 34-37℃ for 20-30 min. Next, tissue segments were rinsed with enzyme-free saline and then gently triturated with a graded series of fire polished micro-Pasteur pipette. Gentle agitation using various sizes of Pasteur pipettes produced typical single DA neurons. These isolated cells were then plated onto poly-D-lysinecoated small glass cover slips that were already fitted for a recording chamber.
Acutely isolated cells on glass coverslips were rinsed twice with phosphate-buffered saline and fixed with 4% paraformaldehyde for 40 min at room temperature. After fixation, these cells were washed with phosphate-buffered saline and then incubated in phosphate-buffered saline containing 2% normal goat serum and 0.1% Triton X-100 for 60 min at room temperature. Cells were then incubated for 2 hours in phosphate-buffered saline containing tyrosine hydroxylase antibodies (diluted 1 : 1000), 2% normal goat serum, and 0.1% Triton X-100. After rinsing three times with phosphate-buffered saline, they were incubated with fluorescence isothiocvanate (FITC)-conjugated goat anti-mouse IgG (Molecular probes) diluted 1 : 1000 in phosphate-buffered saline at room temperature for 1 h. Excess fluorescent antibodies were removed by washing three times with phosphate-buffered saline. Fluorescence images were then obtained using a Zeiss 510 confocal laserscanning microscope (excitation at 488 nm; emission at 505-545 nm). Detailed descriptions of morphologies and staining results have been previously reported [33].
A patch clamp recording system (EPC-9, HEKA Electronik, Lambreacht, Germany) was used to measure spontaneous firing activities. Patch pipettes were made from 1.5-mm brocilicate glass (WPI, Sarasota, FL, USA) with a Sutter puller (Model P-97, Sutter Instrument, Novato, CA, USA). The resistance of these patch pipettes was between 2 and 3 MΩ. We employed whole-cell and cell-attached configurations in current-clamp mode. In cell-attached and whole-cell mode patch-clamp experiments, electrical signals were continuously sampled at 2 kHz (1 kHz filter). In cellattached mode recording, patch pipettes were filled with normal bath solution. Frequency calculations of spontaneous firing were performed with Igor version 4. (Igor Wavemetrics, Lake Oswego, OR, USA). Interspike interval (ISI) was calculated by counting spike numbers during 1 min. Some data were analyzed using Origin version 6.0 (Microcal Software, Inc., Northampton, MA, USA). When we measured GABA-mediated currents in wholecell configuration, patch pipettes were filled with an KCl-rich internal solution that consisted of 123 mM KCl, 6 mM NaCl, 1.3 mM CaCl2, 1 mM MgCl2, 10 mM EGTA-2K, 10 mM HEPES, 2 mM Mg-ATP, 10 glucose, adjusted to pH 7.3 with KOH.
To increase local GABA within a small area of a single DA neuron, the local uncaging function of the Zeiss 510 confocal microscope was used. We used UV laser (lines 351 and 364 nm) and a 40× oil immersion objective lens (NA=1.3) (Carl Zeiss AG, Germany) in this experiment. Bath solution contained 20 µM (O)-(CNB-caged)-GABA caged-compounds.
Stock solutions of chemicals were prepared with dimethylsulfoxide or triple-distilled water. They were applied in a normal bath solution at known concentrations immediately before using them. The normal bath solution contained 140 mM NaCl, 5 mM KCl, 10 mM HEPES, 10 mM glucose, 1 mM CaCl2, 1 mM MgCl2. The pH and osmolarity were adjusted with NaOH at room temperature (20-24℃) to 7.35 and about 300 mOsm, respectively. Among chemicals related to ionotropic/metabotropic GABA receptors, 6-amino-3-(4-methoxyphenyl)-1(6H)-pyridazin ebutanoic acid hydrobromide (SR95531 hydrobromide, a GABAA receptor antagonist), (2S)-3-[[(1S)-1-(3,4-dichlorophenyl) ethyl]amino-2-hydroxypropyl] (phenylmethyl) phosphinic acid hydrochloride (CGP55845 hydrochloride, GABAB antagonist), 1,2,3,6-tetrahydro-4-pyridinecarboxylic acid hydrochloride (isoguvacine hydrochloride, a specific GABAA receptor agonist), and (R)-4-amino-3-(4-chlorophenyl)butanoic acid (a selective GABAB receptor agonist) were obtained from Tocris Bioscience. UV photolysis of γ-aminobutyric acid, α-carboxy-2-nitrobenzyl ester, trifluoroacetic acid salt (O-(CNB-Caged) GABA) were obtained from Invitrogen.
Acutely isolated DA neurons from the SNc show large sized soma attached with 3-6 multiple long dendrites. They exhibit spontaneous firing in normal bath solution [233]. In such large cells, most neurons were immunopositive to tyrosine hydroxylase (TH) (Fig. 1A) and characteristic electrical and morphological features of DA neurons were observed as shown in Fig. 1B. In both on-cell and whole-cell current-clamp modes, regular spontaneous firing activities were recorded without any external stimuli (Figs. 1B-b,c). Step current injections evoked typical ‘sag’ potentials which quickly returned to resting membrane potential (Figs. 1B-d) due to activation of Ih current [34].
To understand how GABA regulates spontaneous firing in this spontaneously firing DA neuron,, we first applied GABA in various concentrations (Figs. 1C and D). Spontaneously firing activities were measured using cell-attached current-clamp condition. GABA at 0.3 µm did not inhibit spontaneous firing. However, at concentration between 0.5 and 1 µM, it suppressed spontaneous firing without a complete blockade of spontaneous firing. At concentration of more than 5 µM, GABA completely blocked spontaneous firing, although there were differences in initial delays depending on GABA concentration applied (Fig. 1C). By measuring numbers of firing during 1 min in several cells, we were able to draw a dose-response curve of GABA-induced firing inhibition. Its IC50 was 0.87 µM (Fig. 1D).
Next, we examined GABA-induced currents after activation of either GABAA or GABAB receptors in acutely isolated DA neurons (Fig. 2). Whole-cell voltage-clamp experiments were carried out using isolated DA neurons. GABAA or GABAB receptor-mediated currents were measured by applying specific GABAA or GABAB receptor agonists to these acutely isolated DA neurons at −50 mV with a KCl rich pipette solution (Fig. 2). As shown in Fig. 2A, GABA evoked an inward current in all cases (−131.6±17.0, n=7). Isoguvacine (20 µM), a specific GABAA receptor agonist, also induced inward currents in all cells tested (−42.4±5.9 pA, n=4). However, (R)-baclofen (100 µM), a specific GABAB receptor agonist, evoked outward currents (14.8±2.8 pA, n=6, Fig. 2A), suggesting the presence of functioning GABAA and GABAB receptors in DA neurons. These results are compatible with previous reports showing that GABAA receptor is a Cl− channel and that GABAB receptors can activate K+ channels in DA neurons [31]. Next, we used specific antagonists for GABA receptor subtypes. When GABA was applied in the presence of either 2 µM CGP55845 (a GABAB receptor antagonist), or 5 µM SR95531 (a GABAA receptor antagonist), we were able to record inward currents after blocking GABAB receptors (Fig. 2C, upper panel) and outward currents by blocking GABAA receptors (Fig. 2C, lower panel), very similar to results of agonist experiments (Fig. 2A). These results are summarized in Figs. 2B and D. Mean current amplitudes of GABA with a GABAB receptor antagonist and GABA with a GABAA receptor antagonist were −112.14±16.22 pA (n=5) and 12.06±2.50 pA (n=5), respectively (Fig. 2D). From these results, it could be concluded that the SNc DA neurons express both functional GABAA and GABAB receptors.
Next, we examined GABA-induced currents after activation of either GABAA or GABAB receptors in acutely isolated DA neurons (Fig. 2). Whole-cell voltage-clamp experiments were carried out using isolated DA neurons. GABAA or GABAB receptormediated currents were measured by applying specific GABAA or GABAB receptor agonists to these acutely isolated DA neurons at −50 mV with a KCl rich pipette solution (Fig. 2). As shown in Fig. 2A, GABA evoked an inward current in all cases (−131.6±17.0, n=7). Isoguvacine (20 µM), a specific GABAA receptor agonist, also induced inward currents in all cells tested (−42.4±5.9 pA, n=4). However, (R)-baclofen (100 µM), a specific GABAB receptor agonist, evoked outward currents (14.8±2.8 pA, n=6, Fig. 2A), suggesting the presence of functioning GABAA and GABAB receptors in DA neurons. These results are compatible with previous reports showing that GABAA receptor is a Cl− channel and that GABAB receptors can activate K+ channels in DA neurons [31]. Next, we used specific antagonists for GABA receptor subtypes. When GABA was applied in the presence of either 2 µM CGP55845 (a GABAB receptor antagonist), or 5 µM SR95531 (a GABAA receptor antagonist), we were able to record inward currents after blocking GABAB receptors (Fig. 2C, upper panel) and outward currents by blocking GABAA receptors (Fig. 2C, lower panel), very similar to results of agonist experiments (Fig. 2A). These results are summarized in Figs. 2B and D. Mean current amplitudes of GABA with a GABAB receptor antagonist and GABA with a GABAA receptor antagonist were −112.14±16.22 pA (n=5) and 12.06±2.50 pA (n=5), respectively (Fig. 2D). From these results, it could be concluded that the SNc DA neurons express both functional GABAA and GABAB receptors.
To investigate how effectively GABAA and GABAB receptors could inhibit spontaneous firing activities in SNc DA neurons, specific GABA receptor agonists and antagonists were used, because it was previously confirmed that they could specifically block one type of GABA receptors completely in DA neurons. Bath application of either isoguvacine (20 µM) or (R)-Baclofen (100 µM) inhibited spontaneous firing of DA neurons (Fig. 3A). Their inhibitory effects on spontaneous firing activity were not significantly different (Fig. 3B). The firing rate in control was 2.15±0.68 Hz (n=9). Spontaneous firing rates in the presence of isoguvacine or (R)-baclofen were 0.47±0.18 Hz (n=6) or 0.32±0.17 Hz (n=3), respectively (Fig. 3B). Effects of antagonists for GABA receptor subtypes were also examined. In the presence of CGP55845 (2 µM) or SR95531 (5 µM), GABA was applied for 100 sec (Fig. 3C). Inhibition of one type of GABA receptors, either GABAA or GABAB receptors, did not interfere with GABA-induced inhibition of firing in DA neurons (Fig. 3C). After complete blocking either GABAA or GABAB receptors, GABA dramatically blocked or slowed spontaneous firing rate to 0.28±0.37 Hz (n=3) and 0.41±0.03 Hz (n=2), respectively (Fig. 3C). Results are summarized in Fig. 3D. These results indicate that either GABAA or GABAB receptor alone can inhibit spontaneous firing completely.
To investigate whether GABA receptors in the soma or dendrites could regulate spontaneous firing differently in polarized DA neurons, we employed local GABA-uncaging techniques in cell-attached recording condition in which intracellular condition was maintained without any violation via dialysis with pipette solution (Fig. 4A). As shown in Fig. 4A, a typical patch-attached DA neuron had long dendrites. To stimulate similar areas of membrane of the soma as much as those in areas of each dendritic region in proximal and distal dendrites, uncaging areas of GABA were adjusted with the same laser expose time (1.50 msec). For this experiment, isolated neurons having long dendrites of >140 µm were selected as shown in Fig. 4A. Bath application of inactivated (O)-CNB caged GABA (20 µM) did not significantly affect the frequency of spontaneous firing (n=3, data not shown). In this typical neuron, local GABA-uncaging sites are seen as red areas in Fig. 4A. Photolysis of caged-GABA on the soma (ISI=2.69 s, a), proximal dendrite (ISI=3.73 s, b), and distal dendrite (ISI=2.33 s, c) induced complete inhibition of spontaneous firing (Fig. 4B). Red triangles in Fig. 4B mean uncaging time while red colored areas indicate duration of firing inhibition. In six neurons, we found that GABA exposures to any area of a DA neuron inhibited spontaneous firing completely. There were no significant differences (p>0.05) in firing inhibition duration among the soma (3.00±0.22 s, n=6), proximal dendrite (3.72±0.70 s, n=6), and distal dendrite (3.10±0.38 s, n=6) (Fig. 4C). These results indicate that GABA can suppress spontaneous firing by GABA receptors not only in the soma, but also in the part of proximal and distal dendrite of the DA neuron.
To investigate how GABAA and GABAB receptors in the soma and dendritic tree might participate in the firing inhibition of DA neurons, local GABA uncaging using (O)-CNB caged GABA under the presence of specific blockers for GABAA or GABAB receptors was performed. Red areas in Fig. 5 indicate uncaging sites in the soma, proximal, and distal dendrites. After local GABA uncaging, we repeated the same experiment in the presence of either a specific GABAA receptor blocker or a specific GABAB receptor blocker. In the presence of each antagonist for GABAA and GABAB receptors, GABA uncaging at the soma inhibited spontaneous firing similarly (Fig. 5B, top panel). There was no significant difference in receptor-induced firing inhibitions between GABAA and GABAB (GABAA: 1.97±0.03 s, GABAB: 1.83±0.17 s, n=8, Fig. 5B, top panel), suggesting that GABAA or GABAB receptor alone could inhibit spontaneous firing equally in the soma. In contrast, GABA-induced firing inhibitions in dendritic compartment differed from that of the soma. In proximal dendrites, GABAB receptors (3.02±0.09 s, n=8) inhibited spontaneous firing more strongly than GABAA receptors (1.20±0.06 s, n=8, Fig. 5B, middle panel). Responses at distal dendrites were the same as inhibition of firing at proximal dendritic compartment. ISI was 1.32±0.07 s by GABAA receptor activation (n=8). It was 2.38±0.22 s by GABAB receptor mediated firing inhibition (n=8, Fig. 5B, bottom panel). The bar graph shows grade of firing inhibition (isi) at the soma, proximal dendrite, and distal dendrite (red bars=GABAA antagonist, blue bars=GABAB antagonist) (n=8). These data indicate that in the soma GABAA or GABAB receptors can equally suppress spontaneous firing. However, in the dendrites, GABAB receptors can dominantly suppress spontaneous firing.
SNc DA neurons generate spontaneous firing in vivo, in vitro, and under dissociated conditions [235363738]. DA neurons in vivo fire spontaneously in three distinct modes; a tonic regular firing, a random pattern, and a burst firing pattern [35363738]. Because firing modes and/or modulation of regularly firing activity of DA neurons determine DA release, it is very important to understand how excitatory and inhibitory neurotransmitters modulate spontaneous firing activity. Although burst firing is well known to be evoked by glutamatergic afferents [10], it can be also enhanced or evoked by disinhibition of GABAergic afferents in a certain condition [2632]. GABA is a major inhibitory neurotransmitter in the brain. Activation of GABA receptors is known to inhibit neuronal cell activities, but it affects all of the above three firing modes in DA neurons [5639]. GABAergic inputs account for 70% of afferents to the SNc DA neurons [8]. They play a key role in switching of firing patterns in DA neurons [6]. Direct exposure to GABA containing solution inhibits spontaneous firing of DA neurons. This has been reported in brain slices and isolated neurons [1227]. It is also known that nigral DA neurons express both GABAA and GABAB receptors and that application of GABAA or GABAB receptors agonists can inhibit spontaneous firing in vitro [712272829]. However, inhibitory responses of SNc DA neurons by stimulation of afferents from striatum, globus pallidus, or substantia nigra pars reticulata in vivo have been shown to be mediated predominantly by GABAA receptors [32]. GABAergic neurons in the striatum, globus pallidus, and pars reticulata are fast pacemakers that continuously suppress nigral DA neurons. Input activity from the GABA neurons appear to be translated linearly to spike frequency and it is thus likely that this basal ganglia output is frequency coded [151920]. Since both GABAA and GABAB receptors can mediate the inhibitory action of GABA in most neurons including DA neurons [273241], regional distribution of GABAA and GABAB receptors on the soma and/or dendrites in DA neurons appears to be very important for the generation of specific firing patterns and integration of synaptic inputs. In this situation, it is very interesting to see that local electrical stimulation with high frequency trains in vitro evokes both GABAA and GABAB receptors, but that weak stimulation in vivo appears to be mediated exclusively by GABAA receptors [3241]. Therefore, the effect of GABA on spontaneous firing in DA neurons should be determined by strength of GABA afferents as well as location of receptor subtypes. Although GABAB receptors have been primarily studied at the cell body, they are abundant at spines and dendrites [4243] which are known to receive most inhibitory inputs. However, due to the lack of information about distribution of GABAergic buttons and receptors on the somatodendritic tree of DA neurons, it is difficult to exactly estimate how GABA affects firing activities in detail at the moment. In addition, apart from firing regulation, GABA-related mechanisms appear to be involved in neuronal regeneration and axon guidance in the brain [531] as well as in addictive drug actions and reward/aversion pathways [29]. However, GABAA and GABAB type receptor distribution has not been thoroughly tested in DA neurons at single neuron level. Therefore, using patch-clamp recording and local GABA uncaging techniques, we investigated regional roles of GABAA or GABAB receptors in SNc DA neurons.
As a result, in acutely dissociated DA neurons, we confirmed that GABA had inhibitory effect on spontaneous firing. At low concentrations (<5 µM), GABA suppressed tonic firing and reduced spontaneous firing rate. However, at higher concentrations (≥5 µM), it completely abolished spontaneous firing (Fig. 1). Activation of GABAA receptors evoked inward Cl− currents, whereas activation of GABAB receptors seemed to activate outward K+ currents (Fig. 2), similar to results reported in DA neurons [151920]. Although suppressive actions of GABAB receptors in vivo appears to be very weaker than those of GABAA receptors [122732], bath application of GABAA/GABAB receptor agonists and antagonists (in this case together with GABA) showed that spontaneous firing activity could be completely inhibited by activation of only one type of GABAA or GABAB receptor (Fig. 3). Finally, we showed that local application of GABA limited on the soma, proximal dendritic, and distal dendritic region using local caged-GABA uncaging technique, was enough to completely inhibit spontaneous firing (Fig. 4). In case of the soma, activation of GABAA or GABAB receptors equally suppressed spontaneous firing. However, in the proximal and distal dendritic regions, GABAB receptors inhibited spontaneous firing more strongly than GABAA receptors (Fig. 5). This regional difference in the inhibition of GABA receptors between soma and dendritic compartments can help to resolve many complex actions of GABA on spontaneous firing and firing patterns and to understand GABA signaling in DA neurons.
Figures and Tables
Fig. 1
Dose dependence of GABA-induced inhibition of spontaneous firing in SNc DA neurons.
(A and B) Identification of SNc DA neurons. Transmitted and immunofluorescence images of an acutely isolated DA neuron. Expression of tyrosine hydroxylase (TH) was confirmed by staining with THantibody. Spontaneous firing activity (b: cell-attached current-clamp mode, c: whole cell current-clamp mode) was recorded from acutely isolated DA neuron (B-a). DA neurons showed a large sag in voltage response to hyperpolarizing current steps (from - 800 pA with 200 pA increases for 500 ms). (C) Bath application of GABA (0.3 µM to 10 µM) inhibited spontaneous firing activity in a dose-dependent manner. (D) Dose-response curve of GABA-induced inhibition of firing rate (IC50=0.87 µM, n=18).
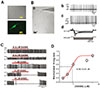
Fig. 2
GABA-induced currents by activation of GABAA and GABAB receptors in SNc DA neurons.
Activation of GABAA and GABAB receptors in DA neurons evoked inward and outward currents at −50 mV, respectively. (A) Typical current responses to isoguvacine hydrochloride (a specific GABAA agonist, 20 µM) and (R)-Baclofen (a specific GABAB agonist, 100 µM). (B) Comparison of GABA, isoguvacine, and baclofen mediated currents (n=7). (C) GABA (10 µM) mediated current in the presence of GABAB receptor antagonist (CGP55845, 2 µM) or GABAA receptor antagonist (SR95531, 5 µM). Inset: 10 µM GABA induced inward current at −50 mV. (D) GABA-induced currents in the presence of GABA receptor antagonists are summarized (n=5). *p<0.05; ***p<0.001 by t-test.
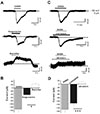
Fig. 3
Inhibition of spontaneous firing by activation of GABAA or GABAB receptors.
(A) Typical firing traces recorded from acutely dissociated DA neurons. Isoguvacine (20 µM) and (R)-Baclofen (100 µM) applications completely inhibited spontaneous firing activity of DA neurons. (B) Effects of GABAA agonist and GABAB agonist on spontaneous firing are summarized (n=9). (C) GABA induced firing inhibitions in the presence of CGP55845 (2 µM) or SR95531 (5 µM). (D) CGP55845 (2 µM) and SR95531 (5 µM) did not alter the effect of GABA (n=5). **p<0.01 by two sample t-test.
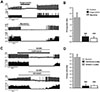
Fig. 4
Inhibition of spontaneous firing by caged-GABA uncaging on the soma and dendrites in DA neurons.
(A) Transmitted image of SNc DA neuron with uncaging sites of (O)-CNB caged-GABA (20 µM). (B) Inhibition of spontaneous firing by uncaging of caged-GABA on the soma, proximal dendrite, and distal dendrite, respectively. Red areas indicated the duration of firing inhibition by caged-GABA uncaging (red triangles) (C) Duration of spontaneous firing inhibition by local caged-GABA uncaging is summarized (n=5). n.s., nonsignificant.
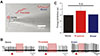
Fig. 5
Regionally different contribution of GABAA and GABAB receptors in the soma and dendrites to firing inhibition of SNc DA neurons.
(A) Transmitted image of DA neuron with location of uncaging sites. (B) Spontaneous firing inhibited by caged-GABA uncaging in the soma, proximal dendrite, and distal dendrite (control). CGP55845 (2 µM), a GABAB receptor specific antagonist, significantly blocked GABA effects in the proximal and distal dendrite. In the soma, GABAA and GABAB receptor antagonists elicited partial block of GABA-induced firing inhibition. Spontaneous firing inhibitions by activation of GABAA and GABAB receptors in three different regions are summarized. *p<0.05; **p<0.01; ***p<0.001 by t-test, n.s., nonsignificant.
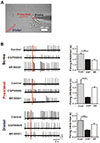
ACKNOWLEDGEMENTS
This study was supported by a grant of the Korean Health Technology R&D Project, Ministry of Health & Welfare, Republic of Korea (HI13C1793). The authors declare no competing financial interests.
Notes
References
1. Grace AA, Onn SP. Morphology and electrophysiological properties of immunocytochemically identified rat dopamine neurons recorded in vitro. J Neurosci. 1989; 9:3463–3481.


2. Jang J, Um KB, Jang M, Kim SH, Cho H, Chung S, Kim HJ, Park MK. Balance between the proximal dendritic compartment and the soma determines spontaneous firing rate in midbrain dopamine neurons. J Physiol. 2014; 592:2829–2844.


4. Missale C, Nash SR, Robinson SW, Jaber M, Caron MG. Dopamine receptors: from structure to function. Physiol Rev. 1998; 78:189–225.


5. Sivilotti L, Nistri A. GABA receptor mechanisms in the central nervous system. Prog Neurobiol. 1991; 36:35–92.


6. Eder M, Rammes G, Zieglgänsberger W, Dodt HU. GABAA and GABAB receptors on neocortical neurons are differentially distributed. Eur J Neurosci. 2001; 13:1065–1069.
7. Tepper JM, Martin LP, Anderson DR. GABAA receptor-mediated inhibition of rat substantia nigra dopaminergic neurons by pars reticulata projection neurons. J Neurosci. 1995; 15:3092–3103.
8. Bolam JP, Smith Y. The GABA and substance P input to dopaminergic neurones in the substantia nigra of the rat. Brain Res. 1990; 529:57–78.


9. Henny P, Brown MT, Northrop A, Faunes M, Ungless MA, Magill PJ, Bolam JP. Structural correlates of heterogeneous in vivo activity of midbrain dopaminergic neurons. Nat Neurosci. 2012; 15:613–619.


10. Grace AA, Floresco SB, Goto Y, Lodge DJ. Regulation of firing of dopaminergic neurons and control of goal-directed behaviors. Trends Neurosci. 2007; 30:220–227.


11. Lobb CJ, Troyer TW, Wilson CJ, Paladini CA. Disinhibition bursting of dopaminergic neurons. Front Syst Neurosci. 2011; 5:25.


12. Erhardt S, Mathé JM, Chergui K, Engberg G, Svensson TH. GABAB receptor-mediated modulation of the firing pattern of ventral tegmental area dopamine neurons in vivo. Naunyn Schmiedebergs Arch Pharmacol. 2002; 365:173–180.
13. McKernan RM, Whiting PJ. Which GABAA-receptor subtypes really occur in the brain? Trends Neurosci. 1996; 19:139–143.
14. Sieghart W. Structure, pharmacology, and function of GABAA receptor subtypes. Adv Pharmacol. 2006; 54:231–263.
15. Lüscher C, Jan LY, Stoffel M, Malenka RC, Nicoll RA. G proteincoupled inwardly rectifying K+ channels (GIRKs) mediate postsynaptic but not presynaptic transmitter actions in hippocampal neurons. Neuron. 1997; 19:687–695.
16. Tamás G, Lorincz A, Simon A, Szabadics J. Identified sources and targets of slow inhibition in the neocortex. Science. 2003; 299:1902–1905.
17. Curtis DR, Hösli L, Johnston GA, Johnston IH. The hyperpolarization of spinal motoneurones by glycine and related amino acids. Exp Brain Res. 1968; 5:235–258.


18. Pandit S, Lee GS, Park JB. Developmental changes in GABAA tonic inhibition are compromised by multiple mechanisms in preadolescent dentate gyrus granule cells. Korean J Physiol Pharmacol. 2017; 21:695–702.
19. Deeb TZ, Lee HH, Walker JA, Davies PA, Moss SJ. Hyperpolarizing GABAergic transmission depends on KCC2 function and membrane potential. Channels (Austin). 2011; 5:475–481.


20. Misgeld U, Bijak M, Jarolimek W. A physiological role for GABAB receptors and the effects of baclofen in the mammalian central nervous system. Prog Neurobiol. 1995; 46:423–462.
21. Newberry NR, Nicoll RA. Direct hyperpolarizing action of baclofen on hippocampal pyramidal cells. Nature. 1984; 308:450–452.


22. Otmakhova NA, Lisman JE. Contribution of Ih and GABAB to synaptically induced afterhyperpolarizations in CA1: a brake on the NMDA response. J Neurophysiol. 2004; 92:2027–2039.
24. Tsubokawa H, Ross WN. IPSPs modulate spike backpropagation and associated [Ca2+]i changes in the dendrites of hippocampal CA1 pyramidal neurons. J Neurophysiol. 1996; 76:2896–2906.
25. Leung LS, Peloquin P. GABAB receptors inhibit backpropagating dendritic spikes in hippocampal CA1 pyramidal cells in vivo. Hippocampus. 2006; 16:388–407.
26. Paladini CA, Tepper JM. GABAA and GABAB antagonists differentially affect the firing pattern of substantia nigra dopaminergic neurons in vivo. Synapse. 1999; 32:165–176.
27. Engberg G, Kling-Petersen T, Nissbrandt H. GABAB-receptor activation alters the firing pattern of dopamine neurons in the rat substantia nigra. Synapse. 1993; 15:229–238.
28. Okada H, Matsushita N, Kobayashi K, Kobayashi K. Identification of GABAA receptor subunit variants in midbrain dopaminergic neurons. J Neurochem. 2004; 89:7–14.
29. Parker JG, Wanat MJ, Soden ME, Ahmad K, Zweifel LS, Bamford NS, Palmiter RD. Attenuating GABAA receptor signaling in dopamine neurons selectively enhances reward learning and alters risk preference in mice. J Neurosci. 2011; 31:17103–17112.
30. Bettler B, Kaupmann K, Mosbacher J, Gassmann M. Molecular structure and physiological functions of GABAB receptors. Physiol Rev. 2004; 84:835–867.
31. Mott DD, Lewis DV. The pharmacology and function of central GABAB receptors. Int Rev Neurobiol. 1994; 36:97–223.
32. Brazhnik E, Shah F, Tepper JM. GABAergic afferents activate both GABAA and GABAB receptors in mouse substantia nigra dopaminergic neurons in vivo. J Neurosci. 2008; 28:10386–10398.
33. Choi YM, Kim SH, Uhm DY, Park MK. Glutamate-mediated [Ca2+]c dynamics in spontaneously firing dopamine neurons of the rat substantia nigra pars compacta. J Cell Sci. 2003; 116:2665–2675.
34. Mercuri NB, Bonci A, Calabresi P, Stefani A, Bernardi G. Properties of the hyperpolarization-activated cation current Ih in rat midbrain dopaminergic neurons. Eur J Neurosci. 1995; 7:462–469.
35. Grace AA, Bunney BS. Intracellular and extracellular electrophysiology of nigral dopaminergic neurons--1. Identification and characterization. Neuroscience. 1983; 10:301–315.


36. Grace AA, Bunney BS. The control of firing pattern in nigral dopamine neurons: burst firing. J Neurosci. 1984; 4:2877–2890.


37. Cardozo DL. Midbrain dopaminergic neurons from postnatal rat in long-term primary culture. Neuroscience. 1993; 56:409–421.


38. Uchida S, Akaike N, Nabekura J. Dopamine activates inward rectifier K+ channel in acutely dissociated rat substantia nigra neurones. Neuropharmacology. 2000; 39:191–201.
39. Tepper JM, Lee CR. GABAergic control of substantia nigra dopaminergic neurons. Prog Brain Res. 2007; 160:189–208.


40. Richards CD, Shiroyama T, Kitai ST. Electrophysiological and immunocytochemical characterization of GABA and dopamine neurons in the substantia nigra of the rat. Neuroscience. 1997; 80:545–557.


41. Johnson SW, North RA. Two types of neurone in the rat ventral tegmental area and their synaptic inputs. J Physiol. 1992; 450:455–468.


42. Beaulieu C, Somogyi P. Targets and quantitative distribution of GABAergic synapses in the visual cortex of the cat. Eur J Neurosci. 1990; 2:296–303.


43. Kulik A, Vida I, Luján R, Haas CA, López-Bendito G, Shigemoto R, Frotscher M. Subcellular localization of metabotropic GABAB receptor subunits GABAB1a/b and GABAB2 in the rat hippocampus. J Neurosci. 2003; 23:11026–11035.