Abstract
Dipeptidyl peptidase4 (DPP4) inhibitors such as gemigliptin are anti-diabetic drugs elevating plasma concentration of incretins such as GLP-1. In addition to the DPP4 inhibition, gemigliptin might directly improve the functions of vessels under pathological conditions. To test this hypothesis, we investigated whether the acetylcholine-induced endothelium dependent relaxation (ACh-EDR) of mesenteric arteries (MA) are altered by gemigliptin pretreatment in Spontaneous Hypertensive Rats (SHR) and in Wistar-Kyoto rats (WKY) under hyperglycemia-like conditions (HG; 2 hr incubation with 50 mM glucose). ACh-EDR of WKY was reduced by the HG condition, which was significantly recovered by 1 µM gemigliptin while not by saxagliptin and sitagliptin up to 10 µM. The ACh-EDR of SHR MA was also improved by 1 µM gemigliptin while similar recovery was observed with higher concentration (10 µM) of saxagliptin and sitagliptin. The facilitation of ACh-EDR by gemigliptin in SHR was not observed under pretreatment with NOS inhibitor, L-NAME. In the endotheliumdenuded MA of SHR, sodium nitroprusside induced dose-dependent relaxation was not affected by gemigliptin. The ACh-EDR in WKY was decreased by treatment with 30 µM pyrogallol, a superoxide generator, which was not prevented by gemigliptin. Exendin-4, a GLP-1 analogue, could not enhance the ACh-EDR in SHR MA. The present results of ex vivo study suggest that gemigliptin enhances the NOS-mediated EDR of the HG-treated MA as well as the MA from SHR via GLP-1 receptor independent mechanism.
Type 2 diabetes mellitus (T2DM) is a well-established risk factor for cardiovascular disease (CVD). Diabetic patients have 2-to 4-fold greater risk of developing coronary artery disease (CAD) compared to non-diabetic patients [1]. Furthermore, diabetes is responsible for the rise of stroke incidence and the increased risk of heart failure [2]. Therefore, glucose lowering agent with CVD preventing effect is expected to provide additional benefit for diabetic patients.
Dipeptidyl peptidase-4 (DPP4) is known as an intrinsic membrane glycoprotein and a ubiquitous serine peptidase that cleaves X-proline dipeptides from the N-terminus of polypeptides. DPP4 degrades peptide hormone incretins such as GLP-1 and gastric inhibitory polypeptide (GIP). DPP4 inhibitors would increase the concentration of GLP-1 by preventing the degradation in vivo [3]. GLP-1 promotes insulin secretion from the pancreatic β cells resulting decrease of blood glucose in diabetic patients. On this background, DPP4 inhibitors, also called gliptins, are prescribed as a class of oral antidiabetic drugs.
It is well confirmed that DPP4 inhibitors reduce glycated hemoglobin (HbA1c) levels with clinically equivalent efficacy compared to other oral diabetic drugs without significant adverse effects such as hypoglycemia, weight gain and gastrointestinal trouble [4]. Most DPP4 inhibitors are prescribed for the treatment of T2DM as monotherapy or in combination with other class agents, such as metformin. Along with the glucose lowering effect, DPP4 inhibitors have been suggested to exert beneficial effects on the cardiovascular risk factors, including dyslipidemia and hypertension [45]. In various cardiovascular disease animal models, DPP4 inhibitors show beneficial effects [6789].
Diabetes is associated with endothelial dysfunction resulting in reduced NO release and excessive generation of reactive oxygen species (ROS) [10]. Thus, the beneficial effects of DPP4 inhibitors on cardiovascular diseases could be due to the anti-hyperglycemic effects. On the other hand, however, one cannot exclude the direct effect of DPP4 inhibitors on vascular functions, such as promoting the endothelium-dependent vasorelaxation. Such additional beneficial effect would be an interesting property of DPP4 inhibitors as therapeutic agents. The impaired function of endothelium could be measured as the reduced endothelium-dependent relaxation (EDR). Experimentally, the harmful effect of hyperglycemia on EDR can be demonstrated from the increased EC50 of ACh for the EDR of maximally contracted arteries.
Gemigliptin is an oral anti-hyperglycemic agent (anti-diabetic drug) of the new DPP4 inhibitor class of drugs approved in global markets [1112]. However, it has not been investigated whether gemigliptin directly affect EDR in disease models as well as in normal control. The purpose of this study was to identify the effect of gemigliptin and other DPP4 inhibitors (sitagliptin and saxagliptin) on the EDR of isolated arteries exposed to hyperglycemic conditions and to chronic hypertensive states.
Male Wistar-Kyoto rats (WKY, n=16) and male spontaneously hypertensive rats (SHR, n=23) were used in this study. We purchased 12 weeks old WKY/Izm and SHR/Izm from Japan SLC Inc (Hamamatsu, Japan). This investigation was in accordance with the Guide for the Care and Use of Laboratory Animals published by US National Institutes of Health (8th Edition, revised 2011) and also conformed to the Institutional Animal Care and Use Committee (IACUC) of Seoul National University (IACUC approval NO. SNU-170221-4). The rats were sacrificed by heart excision after full anesthesia using ketamine and xylazine (ketamine 90 mg/kg and xylazine 10 mg/kg mixture i.p. injection).
Blood pressures and heart rates were measured twice before sacrificed by using CODA non-invasive blood pressure system, tail-cuff method (Kent Scientific corporation, CT, USA). Rats were warmed at 32–34℃ for 20 min before the measurement. Each value used in the analysis was the means of ten times recordings. The tail-cuff blood pressure monitoring showed significantly higher blood pressure in the SHR than WKY (Fig. 1A). Also, the SHR used in this study weighed less than WKY (Fig. 1B).
After sacrificing the rats, the intestine was rapidly removed with omentum and submerged in cold Normal Tyrode (NT) solution. The NT solution for dissection contained (in mmol/l): 140 NaCl, 5.4 KCl, 0.33 NaH2PO4, 10 HEPES, 10 glucose, 1.8 CaCl2 and 1 MgCl2, pH 7.4 titrated with NaOH. Mesenteric arteries (MA) were carefully dissected as 3 mm length of segments using micro-scissors under surgical stereomicroscope view.
The segments of MA were mounted on 25 µm wires in a dual-wire myograph system (620 M; DMT, Aarhus, Denmark) and stabilized in physiological salt solution (PSS) equilibrated with 21% O2, 5% CO2 and N2 balanced at 37℃. The PSS to measure arterial tension contained (in mmol/l): 118 NaCl, 4 KCl, 0.44 NaH2PO4, 24 NaHCO3, 1.8 CaCl2, 1 MgSO4 and 5.6 glucose. To confirm the viability of arteries, the response to 80 mM KCl-PSS (80K) was measured initially. In each vessel, EDR was evaluated by applying 10 µM ACh in the presence of 10 µM Phenylephrine (PhE). To exposure high glucose (HG) condition, the glucose concentration was elevated to 50 mM in PSS with gas bubbling during 2 h.
Gemigliptin were obtained from LG Chem, Ltd. Sitagliptin was purchased from AdooQ BioScience (Irvine, CA, USA) and saxagliptin was purchased from Combi-Blocks Inc. (San Diego, CA, USA). All other drugs and chemicals used in this study were purchased from Sigma-Aldrich (St.Louis, MO, USA). Gemigliptin and sitagliptin were dissolved in DMSO and a total amount of DMSO was set below 0.5%. Others were dissolved in distilled water.
Data are presented as the mean values±SEM with number of tested arteries indicated as n. The relaxation to ACh or SNP was presented as a percentage of the 5 µM PhE precontraction (% Relaxation). Unpaired Student's t-test and one-way ANOVA were used for statistical analysis. To calculate the EC50, the concentration-response curves were fitted to Logistic function by using OriginPro8 (OriginLab Corporation, Northampton, MA, USA). A value of p<0.05 was considered to be statistically significant.
In each tested MA of WKY, near-maximum contraction was induced by 5 µM PhE. Then, increasing concentrations of ACh (0.1 nM–10 µM) were applied to induce endothelium dependent relaxations (EDR). With normal level of glucose (5.6 mM), the half relaxation concentration (EC50) of ACh was 37.1±3 nM (Fig. 2A, black line). When exposed to the HG condition (50 mM glucose, 2 h), EC50 of ACh-induced EDR (ACh-EDR) was increased, and the maximum relaxation level was also reduced in the MAs of WKY (Fig. 2A). When treated with 1 µM or 10 µM gemigliptin for 2 h along with HG, both the maximum relaxation and EC50 were recovered while not perfect (Fig. 2B). The 10 µM gemigliptin alone did not significantly change the ACh-EDR in WKY MA (Fig. 2B, gray line). Different from gemigliptin, treatment of 1 µM sitagliptin or saxagliptin had no effect on the ACh-EDR in WKY MA under HG condition (Fig. 2C). Even the treatment with 10 µM sitagliptin or saxagliptin could not recover the ACh-EDR impaired by the HG condition (Fig. 2D). Table 1 showed summary of EC50 values in WKY MA exposed to HG with or without DPP4 inhibitors.
The ACh-EDR in MA from SHR were impaired when compared with the responses of WKY. The EC50 of ACh in SHR was 79.8±12 nM (Fig. 3A, red line). Interestingly, the incubation with 1 µM or 10 µM gemigliptin for 2 h increased the sensitivity to ACh in SHR (Fig. 3B). However, the pretreatment with saxagliptin and sitagliptin at 1 µM did not improve the ACh-EDR in SHR (Fig. 3C). Nevertheless, 2 h incubation with 10 µM saxagliptin and sitagliptin could partly improve the ACh-EDR in SHR (Fig. 3D). Table 2 showed summary of EC50 values in SHR MA with or without pretreatment with DPP4 inhibitors.
To evaluate the contribution of NO-dependent mechanism in the improvement of EDR by gemigliptin, MAs from SHRs were also pretreated with NOS inhibitor, 100 µM L-NAME for 2 h. Both ACh sensitivity and maximum relaxation were significantly reduced by the L-NAME treatment (Fig. 4A, brown line). The L-NAME-resistant component of ACh-EDR was thought to be mediated by endothelium-dependent hyperpolarization (EDH) mechanisms. In the presence of L-NAME, however, 1 µM gemigliptin could not change the EC50 of the ACh-EDR in SHR (Fig. 4A, green line).
To confirm whether gemigliptin affects mesenteric smooth muscles directly, endothelium removed MAs from SHRs by rubbing were used as checking EDR response to ACh 10 µM with PhE precontraction. The dose response of SNP (0.1 nM–10 µM), a NO donor, was performed in endothelium denuded MAs from SHRs. The concentration-dependent relaxation by SNP were not affected by gemigliptin 1 µM, which suggest that gemigliptin did not directly affect the MA smooth muscle (Fig. 4B).
Excessive ROS generated under various pathological conditions could impair the effect of NO [1314]. We tested whether gemigliptin 1 µM could play as an ROS scavenger. WKY MAs were pretreated a superoxide generator, pyrogallol (30 µM, 2 h) that induced EDR impairment. A combined treatment with gemigliptin did not show recovery of the sensitivity and maximum relaxation to ACh (Fig. 4C). These data showed that gemigliptin did not play a role as a ROS scavenger.
In this study, we analyzed the effects of DPP4 inhibitors (gemigliptin, sitagliptin and saxagliptin) on the ACh-EDR of SHR MA and of HG-treated WKY MA. Among the three DPP4 inhibitors tested in the present study, gemigliptin was more effective in improving the ACh-sensitivity of EDR (Fig. 2 and Fig. 3). The pharmacological tests suggest that the improvement of EDR by gemigliptin would be mediated by eNOS-dependent pathway (Fig. 4). Since the SNP-sensitivity of endothelium-denuded MA was not altered by gemigliptin, endothelium but not the smooth muscle seems to be the target site. Interestingly, the treatment with gemigliptin alone did not enhance the ACh-EDR of the normal WKY MA (Fig. 2A). Thus, it is suggested that the beneficial effect of gemigliptin appears to be specific to the pathological conditions, such as hyperglycemia and hypertension.
It is generally accepted that endothelial dysfunction is one of the hallmark of diabetes and hypertension [16]. Even for the normal WKY MA, exposure to HG for 2 h significantly attenuated the ACh-EDR, which was recovered by 1 µM gemigliptin cotreatment (Fig. 2B) while not by sitagliptin or saxagliptin up to 10 µM (Figs. 2C and D). Similar to the previous study [16], ACh-EDR was impaired in the SHR MA (Fig. 3A). Interestingly the improving effect on gemigliptin on the ACh-EDR in the SHR MA was more potent than those of sitagliptin and saxagliptin (Figs. 3B–D).
To investigate the EDR unrelated with the NO production (e.g. endothelium-dependent hyperpolarization factor) mediated relaxation, the ACh-EDR of SRH MA were examined in the presence of NOS inhibitor, L-NAME. The sensitivity to ACh was reduced by treatment of L-NAME, and the remained EDR of SHR could not be recovered by gemigliptin. These results indicated that gemigliptin might have facilitated the NOS or NO-dependent signaling pathway in the SHR or HG-treated MA. To confirm whether gemigliptin directly affects the arterial smooth muscle cells, we examined the effect of gemigliptin in endothelium denuded MA from SHRs. Dose-response of NO donor, SNP, showed similar sensitivity between with and without gemigliptin. The results suggest that gemigliptin had no concern with smooth muscles.
Endothelial dysfunction in hyperglycemia or hypertension is often associated with pathological increase in ROS. In fact, the treatment with pyrogallol, a superoxide generator, significantly decreased the ACh-EDR in WKY MA. However, gemigliptin treatment did not prevent the EDR impairment by pyrogallol (Fig. 4C). Although previous studies with other DPP4 inhibitors (ex. linagliptin) suggested putative antioxidant effect [17], our present study indicate that gemigliptin might not be an effective antioxidant or ROS scavenger.
Some previous studies reported that the cardiovascular beneficial effects by DPP4 inhibitors were mediated by GLP-1 dependent mechanism [171819]. GLP-1 itself may act on the vessels via their receptor (GLP-1R), producing cyclic AMP and activating PKA. In addition, GLP-1 dilates via release of nitric oxide (NO) from vascular endothelial cells [20]. In several previous studies, GLP-1 receptor agonist mimicked cardiovascular protective effect of DPP4 inhibitors showing that DPP4 inhibitors act on via GLP-1 pathway. However, our present study showed that the GLP-1R agonist, exendin-4, did not directly affect the ACh-EDR of SHR MA (Fig. 4D). Considering the ex vivo condition of present experiment, the results of gemigliptin would not be mediated by stimulating GLP-1R that are not expressed in the SHR MA myocytes or endothelium.
Despite the intriguing beneficial effects of gemigliptin on ACh-EDR, the present study has limitations: 1) since we applied DPP4 inhibitors only for 2 h, effects of more prolonged exposure on endothelial function is not known, 2) since the clinical route of application of DPP4 inhibitor is oral, the present ex vivo results might not be equivalent. Therefore, clinical implication need to consideration and further studies will be needed. Despite the limitations, our study indicates that gemigliptin shows more potent EDR enhancing effect than other DPP4 inhibitors in the arteries under hyperglycermic and hypertensive conditions.
ACKNOWLEDGEMENTS
This research was supported by grant No.800-20170043 from LG Chem Ltd and the Research Fund from Seoul National University College of Medicine (2017, Basic-Clinical Collaboration Project).
Notes
References
1. Newman AB, Siscovick DS, Manolio TA, Polak J, Fried LP, Borhani NO, Wolfson SK. Ankle-arm index as a marker of atherosclerosis in the Cardiovascular Health Study. Cardiovascular Heart Study (CHS) Collaborative Research Group. Circulation. 1993; 88:837–845. PMID: 8353913.


2. Martín-Timón I, Sevillano-Collantes C, Segura-Galindo A, Del Cañizo-Gómez FJ. Type 2 diabetes and cardiovascular disease: have all risk factors the same strength? World J Diabetes. 2014; 5:444–470. PMID: 25126392.


3. Ahrén B. Dipeptidyl peptidase-4 inhibitors: clinical data and clinical implications. Diabetes Care. 2007; 30:1344–1350. PMID: 17337494.
4. Papagianni M, Tziomalos K. Cardiovascular effects of dipeptidyl peptidase-4 inhibitors. Hippokratia. 2015; 19:195–199. PMID: 27418775.
5. Fadini GP, Avogaro A. Cardiovascular effects of DPP-4 inhibition: beyond GLP-1. Vascul Pharmacol. 2011; 55:10–16. PMID: 21664294.


6. Sauvé M, Ban K, Momen MA, Zhou YQ, Henkelman RM, Husain M, Drucker DJ. Genetic deletion or pharmacological inhibition of dipeptidyl peptidase-4 improves cardiovascular outcomes after myocardial infarction in mice. Diabetes. 2010; 59:1063–1073. PMID: 20097729.


7. Esposito G, Cappetta D, Russo R, Rivellino A, Ciuffreda LP, Roviezzo F, Piegari E, Berrino L, Rossi F, De Angelis A, Urbanek K. Sitagliptin reduces inflammation, fibrosis and preserves diastolic function in a rat model of heart failure with preserved ejection fraction. Br J Pharmacol. 2017; 174:4070–4086. PMID: 27922176.


8. Koibuchi N, Hasegawa Y, Katayama T, Toyama K, Uekawa K, Sueta D, Kusaka H, Ma M, Nakagawa T, Lin B, Kim-Mitsuyama S. DPP-4 inhibitor linagliptin ameliorates cardiovascular injury in salt-sensitive hypertensive rats independently of blood glucose and blood pressure. Cardiovasc Diabetol. 2014; 13:157. PMID: 25471116.


9. Ihara M, Asanuma H, Yamazaki S, Kato H, Asano Y, Shinozaki Y, Mori H, Minamino T, Asakura M, Sugimachi M, Mochizuki N, Kitakaze M. An interaction between glucagon-like peptide-1 and adenosine contributes to cardioprotection of a dipeptidyl peptidase 4 inhibitor from myocardial ischemia-reperfusion injury. Am J Physiol Heart Circ Physiol. 2015; 308:H1287–H1297. PMID: 25747753.


10. Rodríguez-Mañas L, Angulo J, Vallejo S, Peiró C, Sánchez-Ferrer A, Cercas E, López-Dóriga P, Sánchez-Ferrer CF. Early and intermediate Amadori glycosylation adducts, oxidative stress, and endothelial dysfunction in the streptozotocin-induced diabetic rats vasculature. Diabetologia. 2003; 46:556–566. PMID: 12739028.


11. Gutch M, Joshi A, Kumar S, Agarwal A, Pahan RK, Razi SM. Gemigliptin: newer promising gliptin for type 2 diabetes mellitus. Indian J Endocrinol Metab. 2017; 21:898–902. PMID: 29285456.


12. Park SE, Lee BW, Kim JH, Lee WJ, Cho JH, Jung CH, Lee SH, Suh S, Hur GC, Kim SH, Jang YH, Park CY. Effect of gemigliptin on glycaemic variability in patients with type 2 diabetes (STABLE study). Diabetes Obes Metab. 2017; 19:892–896. PMID: 28058753.


13. Incalza MA, D'Oria R, Natalicchio A, Perrini S, Laviola L, Giorgino F. Oxidative stress and reactive oxygen species in endothelial dysfunction associated with cardiovascular and metabolic diseases. Vascul Pharmacol. 2018; 100:1–19. PMID: 28579545.


14. Craige SM, Kant S, Keaney JF Jr. Reactive oxygen species in endothelial function - from disease to adaptation. Circ J. 2015; 79:1145–1155. PMID: 25986771.
15. Dickson SL, Shirazi RH, Hansson C, Bergquist F, Nissbrandt H, Skibicka KP. The glucagon-like peptide 1 (GLP-1) analogue, exendin-4, decreases the rewarding value of food: a new role for mesolimbic GLP-1 receptors. J Neurosci. 2012; 32:4812–4820. PMID: 22492036.


16. Silva BR, Pernomian L, Bendhack LM. Contribution of oxidative stress to endothelial dysfunction in hypertension. Front Physiol. 2012; 3:441. PMID: 23227009.


17. Salheen SM, Panchapakesan U, Pollock CA, Woodman OL. The DPP-4 inhibitor linagliptin and the GLP-1 receptor agonist exendin-4 improve endothelium-dependent relaxation of rat mesenteric arteries in the presence of high glucose. Pharmacol Res. 2015; 94:26–33. PMID: 25697548.


18. Liu L, Liu J, Wong WT, Tian XY, Lau CW, Wang YX, Xu G, Pu Y, Zhu Z, Xu A, Lam KS, Chen ZY, Ng CF, Yao X, Huang Y. Dipeptidyl peptidase 4 inhibitor sitagliptin protects endothelial function in hypertension through a glucagon-like peptide 1-dependent mechanism. Hypertension. 2012; 60:833–841. PMID: 22868389.


19. Hausenloy DJ, Whittington HJ, Wynne AM, Begum SS, Theodorou L, Riksen N, Mocanu MM, Yellon DM. Dipeptidyl peptidase-4 inhibitors and GLP-1 reduce myocardial infarct size in a glucose-dependent manner. Cardiovasc Diabetol. 2013; 12:154. PMID: 24148218.


20. Poudyal H. Mechanisms for the cardiovascular effects of glucagon-like peptide-1. Acta Physiol (Oxf). 2016; 216:277–313. PMID: 26384481.


Fig. 1
Blood pressure and body weights of WKY (n=16) and SHR (n=23) rats.
(A) Systolic and diastolic blood pressures were measured by tail-cuff methods. The blood pressures were significantly higher in SHR than WKY rats (***p<0.001). (B) SHR rats weighed less than WKY significantly (***p<0.001).
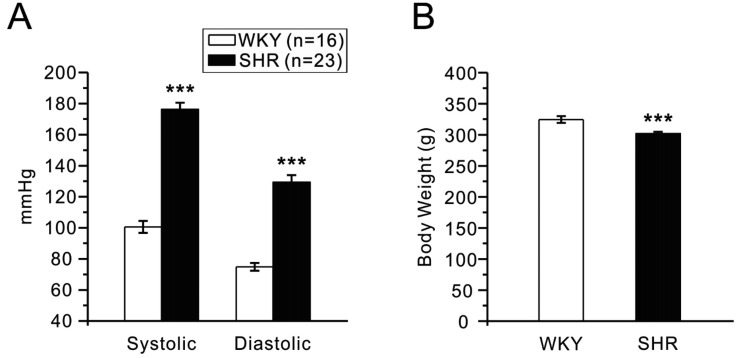
Fig. 2
Improvement of ACh-EDR by gemigliptin in WKY MA exposed to 50 mM glucose (HG).
Cumulative concentration-dependent relaxation of endothelium-intact MA were obtained in the presence of 5 µM PhE (ACh-EDR), and normalized to the steady-state contraction by PhE. HG condition (n=31) attenuated ACh-EDR of WKY MA (A), and the treatment with gemigliptin (1 µM; n=18, 10 µM; n=18) induced partial recovery of ACh-EDR (B). However, other DPP-4 inhibitors, sitagliptin (1 µM; n=12, 10 µM; n=12) and saxagliptin (1 µM; n=12, 10 µM; n=12) did not (C, D). n indicated the number of experiments. The concentration-response curves were plotted by using different colored symbols for each group. Statistical differences were analyzed between the tested groups at each concentration of ACh. The significance was marked by asterisks of the corresponding color (**p<0.01, ***p<0.001).
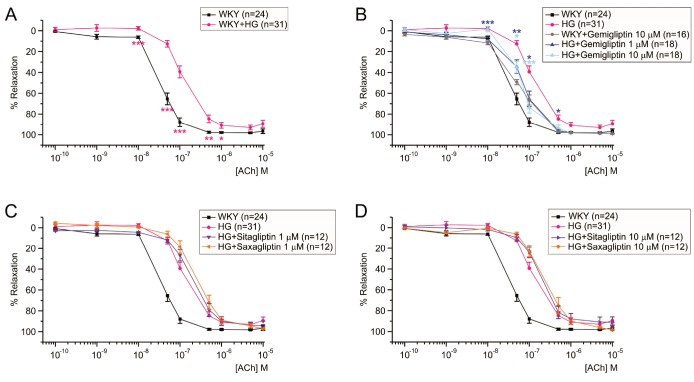
Fig. 3
More effective improvement of ACh-EDR by gemigliptin than sitagliptin and saxagliptin in SHR MA.
The normalized concentration-response curves to ACh were obtained. Statistical differences were analyzed between the tested groups at each concentration of ACh, and the significance was marked by asterisks of the color corresponding to each group symbol (*p<0.05, **p<0.01, ***p<0.001). The sensitivity to ACh was lower in SHR (n=23) than WKY (n=24) (A). Incubation with gemigliptin at both 1 (n=8) and 10 µM (n=8) similarly improved the sensitivity to ACh in SHR MA (B). However, pretreatment with 1 µM sitagliptin (n=8) or 1 µM saxagliptin (n=8) did not recover ACh-EDR in SHR MA (C). Treatment of 10 µM sitagliptin (n=8) improved ACh-EDR slightly in SHR MA, whereas the treatment of 10 µM saxagliptin (n=8) did not (D). n indicated the number of experiments.
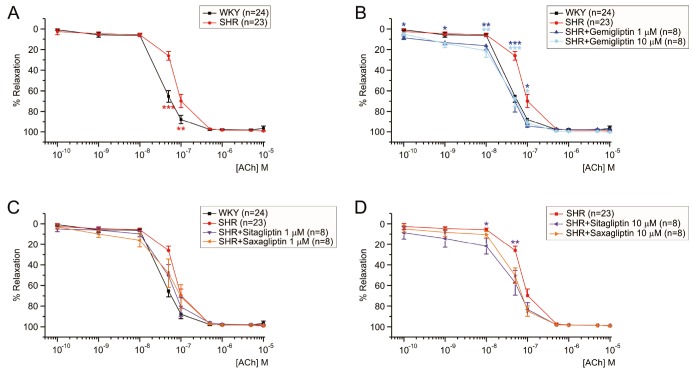
Fig. 4
Pharmacological investigation of the mechanism underlying the EDR improvement by gemigliptin.
Cumulative concentration-response curves to ACh (A, C, D) or to SNP (B) in MAs of WKY (C) and SHR (A, B, D). (A) No effect of gemigliptin on ACh-induced EDR in the presence of L-NAME (n=12). (B) No difference of SNP-induced relaxation in the absence of functional endothelium. (C) No improving effect of gemigliptin on EDR under the pretreatment with 30 µM pyrogallol (n=12). (D) No improvement of ACh-EDR by 1 µM exendin-4 pretreatment in SHR MA (n=10). In each group of experiments, tonic contraction of MA was initially induced by 5 µM PhE. Statistical significance was marked by asterisks of the color corresponding to each group symbol. n indicated the number of experiments.
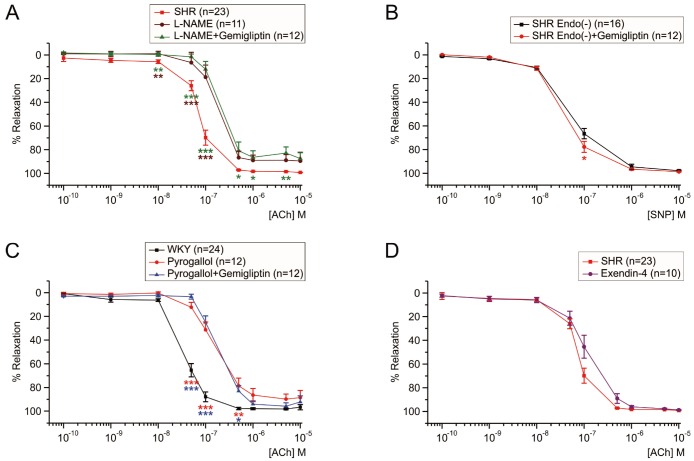