Abstract
Objective
The purposes of this study were to evaluate size-specific dose estimate (SSDE) of low-dose CT (LDCT) in the Korean Lung Cancer Screening (K-LUCAS) project and to determine whether CT protocols from Western countries are appropriate for lung cancer screening in Korea.
Materials and Methods
For participants (n = 256, four institutions) of K-LUCAS pilot study, volume CT dose index (CTDIvol) using a 32-cm diameter reference phantom was compared with SSDE, which was recalculated from CTDIvol using size-dependent conversion factor (f-size) based on the body size, as described in the American Association of Physicists in Medicine Report 204. This comparison was subsequently assessed by body mass index (BMI) levels (underweight/normal vs. overweight/obese), and automatic exposure control (AEC) adaptation (yes/no).
Results
Size-specific dose estimate was higher than CTDIvol (2.22 ± 0.75 mGy vs. 1.67 ± 0.60 mGy, p < 0.001), since the f-size was larger than 1.0 for all participants. The ratio of SSDE to CTDIvol was higher in lower BMI groups; 1.26, 1.37, 1.43, and 1.53 in the obese (n = 103), overweight (n = 70), normal (n = 75), and underweight (n = 4), respectively. The ratio of SSDE to CTDIvol was greater in standard-sized participants than in large-sized participants independent of AEC adaptation; with AEC, SSDE/CTDIvol in large- vs. standard-sized participants: 1.30 ± 0.08 vs. 1.44 ± 0.08 (p < 0.001) and without AEC, 1.32 ± 0.08 vs. 1.42 ± 0.06 (p < 0.001).
Low-dose CT (LDCT) screening reduces lung cancer mortality in high-risk individuals. Specifically, in the National Lung Screening Trial, in current or ex-smokers aged 55–74 years who had a smoking history of at least 30 pack-years and had quit within the last 15 years, lung cancer specific mortality was 20.0% lower in the LDCT screening arm than in the chest X-ray screening arm (1). On the basis of this result, the Korean Lung Cancer Screening (K-LUCAS) project had been launched and the pilot study for K-LUCAS was performed to evaluate the feasibility on the implementation of national screening with LDCT for high-risk subject of lung cancer (2).
Although LDCT uses a low level of radiation dose, the individual will nevertheless be exposed to radiation from screening CT. Moreover, LDCT examinations would be recommended more than one time per year according to the study results. To accurately evaluate the potential harm from radiation exposure associated with LDCT, the quantification of radiation dose is mandatory. Currently, CT manufacturers are required to display scanning protocol information (mAs, kVp, and pitch), as well as selected dosimetry metrics (volume CT dose index [CTDIvol] and dose-length product) (3). In particular, CTDIvol was developed as a standardized method to compare radiation output levels between different CT scanners using a reference phantom: a polymethyl methacrylate cylinder either 16 cm or 32 cm in diameter that is often called the “head” or “body” CTDI phantom, respectively. However, CTDIvol estimates based on reference phantoms have potential errors, because they do not take body size into account when calculating CT radiation dose. Furthermore, it is unclear whether LDCT lung cancer screening protocols for Western people is also appropriate for Asian populations who have smaller body size. Therefore, the purposes of our study were to evaluate the body size-specific radiation dose of LDCT examination in the K-LUCAS project and to determine whether CT protocols from Western countries are appropriate for lung cancer screening in Korean people.
The K-LUCAS pilot study aimed to evaluate the feasibility of CT screening in individuals at high-risk of lung cancer in South Korea. The study was carried out between November 2016 and March 2017. The National Cancer Center and three regional cancer centers in South Korea participated in the study. To qualify for inclusion in the K-LUCAS pilot study, the individuals were required to be asymptomatic, be current or ex-smokers, be aged 55–74 years, and have a smoking history of at least 30 pack-years, with tobacco use within the last 15 years. Subjects were not included in the study if they had poor performance status (Eastern Cooperative Oncology Group performance status ≥ 2) or a history of lung cancer. Subjects were also excluded if they were undergoing treatment for pulmonary disease such as tuberculosis, pneumonia, or interstitial lung disease, had been diagnosed as having any cancer other than thyroid and skin cancers within the previous 5 years, or had undergone chest CT within the 6 months prior to study initiation.
The participants' height and weight were recorded, and body mass index (BMI) was calculated as weight divided by the square of their height (kg/m2). BMIs were categorized as underweight (< 18.5 kg/m2), normal weight (18.5–22.9 kg/m2), overweight (23.0–24.9 kg/m2), or obese (≥ 25 kg/m2) (4). Standard-sized participant include normal/underweight participants and large-sized participant include overweight and obesity.
The pilot study was approved by the Institutional Review Board of each screening center, and each participant provided informed consent.
The screening LDCT scan was performed from the lung apex to the lung base, without contrast administration, using the following K-LUCAS specifications to minimize radiation exposure: use of multidetector CT with a minimum of 16 channels, completion of scan within one breath hold, gantry rotation time of < 0.5 seconds) radiation dose ≤ 3.0 mGy in standard-sized subjects (height of about 170 cm, body weight of about 70 kg, or BMI of about 24 kg/m2), image reconstruction using both thin (1.0–1.25 mm) and thick slices (2.5–3.0 mm). These specifications were based on the previous LDCT technical specifications detailed by the American College of Radiology (ACR) and the Society of Thoracic Radiology (STR) (56); they were modified by the Korean Society of Thoracic Radiology. Additional coronal reconstruction images were recommended. Furthermore, advanced techniques such as automatic exposure control (AEC), and iterative reconstruction methods are also recommended for smoothening of the images and reducing the quantum noise. AEC systems modulate tube current in the longitudinal and angular directions to adjust scanner output according to the attenuation for each patient at different tube positions. Effective radiation doses were estimated by multiplying the dose-length product by a conversion factor of 0.014 mSv/mGy·cm (7). All technicians were trained in these protocols, and the CT scanning parameters and radiation dose output were recorded and reviewed. Considering the heterogeneity of CT equipment between institutions, all scanning parameters other than the essential requirements were allowed to be modified according to the institutions' situations and preferences. Furthermore, there were no specific regulations for scanning parameters such as tube current, tube voltage, and radiation dose in large-sized participants in this pilot study.
A list of CT scanning parameters, CTDIvol values, and phantom diameters used (32-cm diameter reference phantom) was obtained from the Digital Imaging and Communications in Medicine dose report. Although CTDIvol is dependent on the scan parameters (tube voltage, tube current, gantry rotation time, and pitch), it is independent of patient size.
To estimate subject-specific radiation dose from LDCT, size-specific dose estimates (SSDEs) were recalculated from the CTDIvol, as recommended by the American Association of Physicists in Medicine (AAPM) Report 204 (8); body size (antero-posterior [AP] and lateral [LAT] dimensions) were measured at mid-liver level on the LDCT images (Fig. 1). The tabulated body size-dependent conversion factor (f-size), combined with measurement of the participant's body size (AP plus LAT dimensions), enables conversion from CTDIvol to SSDE according to the following equation: SSDE = f-size × CTDIvol (8).
Descriptive statistics are reported as proportions or means with standard deviations.
Using the methods described, we compared the CTDIvol and SSDE using the paired t test. In addition, the ratio of SSDE to CTDIvol (SSDE/CTDIvol) was subsequently compared in terms of BMI level (standard-sized vs. large-sized participants) and use of AEC adaptation (yes vs. no). Linear regression analysis was employed to assess the relationship between two continuous variables.
Two-sided p values of < 0.05 were considered statistically significant. Analysis was performed using SPSS for Windows ver. 19.0 (IBM Corp., Armonk, NY, USA).
For this pilot study, a total of 256 participants were enrolled from four institutions across South Korea. The baseline characteristics of the population are summarized in Table 1. Mean age was 63.2 ± 5.4 years and 98.8% (253/256) were men. A total of 145 subjects (56.6%) were current smokers, and 111 subjects (43.4%) were ex-smokers. Their mean BMI was 24.4 ± 3.0 kg/m2, and the proportions of participants in each BMI category were as follows: underweight (1.6%), normal weight (29.6%), overweight (28.1%), and obese (40.7%).
Table 2 summarizes the LDCT scanning and reconstruction protocols in this pilot study. The K-LUCAS specifications and recommendations are also included for comparison. All participants were scanned using either a 64-channel CT scanners (n = 234; 91.4%) or a dual-source 128-channel CT scanner (n = 22; 8.6%). A tube voltage of 120 kVp was used in 218 participants (85.2%); 100 kVp was used in the remaining participants. Rotation time was less or equal to 0.5 seconds in all participants (range: 0.28–0.50 seconds). The effective tube current-time product ranged from 16.0 mAs to 64.0 mAs, with an average value of 28.6 mAs. All scans adhered to the thick and thin slice reconstruction requirements, and two institutions added coronal reconstruction images.
Automatic exposure control were applied to 193 subjects in two institutions (Care Dose 4D for the Somatom Sensation 64, Dose Right ACS and D-DOM for the Brilliance 64). The Care Dose 4D (Siemens Healthineers, Forchheim, Germany) is a reference current-based AEC system; the user chooses the reference current value to be used for a standard-sized patient. The quality reference effective mAs was set to 25 mAs, and the AEC strength was set to average among weak, average, and strong options. The Dose Right ACS and D-DOM (Philips Healthcare, Eindhoven, the Netherlands) are reference image-based AEC systems; in these systems, adjustments are made to match the noise on a reference image of appropriate quality for the current clinical requirements. The remaining two institutions acquired CT images without AEC application, using fixed kVp and mAs values independent of BMI level.
The CTDIvol based on a 32-cm diameter reference phantom, as well as the calculated SSDE, are plotted in Figure 2. The mean CTDIvol was 1.67 ± 0.60 mGy (dose length product, 68.63 ± 21.68 mGy·cm) and the effective radiation dose was 0.95 ± 0.36 mSv. In nine participants (3.5%), the CTDIvol exceeded 3 mGy; five of these cases were caused by obesity (mean BMI, 30.7 ± 5.3 kg/m2; range, 25.9–39.0 kg/m2) combined with AEC adaptation, and the remaining four cases (with BMI values of 20.8, 24.7, 25.7, and 26.2 kg/m2, respectively) were caused by erroneous scanning using 50 mAs at one institution (other participants at this institution were scanned using 25 mAs).
Based on body size (mean AP plus LAT dimensions, 56.3 ± 4.1 cm; range, 46.0–72.0 cm), the corresponding f-size was 1.35 ± 0.10 (range, 1.00–1.62). Using these f-size values, the mean SSDE was 2.22 ± 0.75 mGy, which was greater than the CTDIvol in all participants (Fig. 2); the mean difference between SSDE and CTDIvol was 0.56 ± 0.22 mGy (range, 0–1.54 mGy). The SSDE/CTDIvol was higher in the lower BMI groups: 1.26 in obese, 1.37 in overweight, 1.43 in normal weight, and 1.53 in underweight subjects. Although the CTDIvol of large-sized participants was significantly higher than that of standard-sized participants (1.76 ± 0.65 mGy vs. 1.46 ± 0.43 mGy, p < 0.001), the SSDE was not different between these two BMI groups (2.27 ± 0.79 mGy vs. 2.10 ± 0.62 mGy, p = 0.055).
The CTDIvol and SSDE were compared between LDCT with and without AEC. The SSDE/CTDIvol was not different between LDCT with and without AEC (SSDE/CTDIvol: 1.34 ± 0.10 with AEC and 1.35 ± 0.09 without AEC, p = 0.623).
In LDCT with AEC, both CTDIvol and SSDE were greater in large-sized participants than in standard-sized participants (large- vs. standard-sized participants: CTDIvol = 1.86 ± 0.61 mGy vs. 1.47 ± 0.37 mGy, p < 0.001; SSDE = 2.40 ± 0.73 mGy vs. 2.10 ± 0.53 mGy, p = 0.002). There was linear correlation between CTDIvol and BMI (R2 = 0.212, p < 0.001) and between SSDE and BMI (R2 = 0.063, p < 0.001).
In LDCT without AEC, neither CTDIvol nor SSDE were significantly different between the two BMI groups (large- vs. standard-sized participants: CTDIvol = 1.44 ± 0.66 mGy vs. 1.46 ± 0.61 mGy, p = 0.953; SSDE = 1.90 ± 0.85 mGy vs. 2.06 ± 0.88 mGy, p = 0.503). However, the SSDE/CTDIvol was greater in standard-sized participants than in large-sized participants independent of AEC adaptation; with AEC, SSDE/CTDIvol in large- vs. standard-sized participants: 1.30 ± 0.08 vs. 1.44 ± 0.08 (p < 0.001) and without AEC, 1.32 ± 0.08 vs. 1.42 ± 0.06 (p < 0.001) (Fig. 3).
In the present study, participants in the K-LUCAS had relatively small body size compared with a 32-cm reference phantom. Their mean f-size was 1.35 ± 0.10, and the minimum f-size was 1.0 in the largest BMI participant (BMI of 39.0 kg/m2); f-size was > 1.0 in the remaining participants. These data indicate a maximum radiation dose of 3.0 mGy obtained using a 32-cm reference phantom may exceed the appropriate radiation exposure for standard-sized candidates in the Korean population.
Significant linear correlation was observed between f-size and BMI (R2 = 0.704, p < 0.001). Using the f-size distribution of BMI levels, we estimated the optimal radiation dose, defined as a 3 mGy/f-size, using the K-LUCAS participants (Table 3). A radiation dose of ≤ 2.34 mGy could be recommended to standard-sized candidates. For overweight candidates, a dose ≤ 2.48 mGy could be applied, while a dose of ≤ 3.00 mGy could be recommended to obese Korean candidates.
Since CTDIvol only provides information on scanner output, but does not address patient size, it cannot be used to estimate actual radiation exposure (9). This is especially concerning in the case of small-sized and pediatric patients, and the displayed CTDIvol can lead to underestimation of radiation doses.
In this pilot study, we evaluated the CTDIvol and SSDE of screening CT in Korean individuals aged between 55 and 74 years who were at high-risk of lung cancer. The results showed that SSDE was higher than CTDIvol in all participants. The minimum f-size value was 1.0, even for the largest BMI participant, which may reflect the relatively small body size of Korean people compared with a 32-cm diameter reference phantom used to evaluate radiation output. A maximum CTDIvol radiation dose of 3.0 mGy is recommended in standard-sized subjects, based on previous LDCT technical specifications proposed by the ACR and STR (56). However, this dose recommendation may exceed appropriate radiation exposure levels in Korean individuals, who have a relatively small body size. For instance, in one study that evaluated the SSDE of torso CT for adult patients from the United States, the AP plus LAT dimension-based f-size was lower than that of the Korean population. Specifically, the mean f-size was 1.20, ranging from 0.80–1.74 (10).
At the beginning of the present study, we gave no specific recommendations for large-sized participants in terms of scanning parameters such as kVp and mAs, or radiation dose. Such decisions depended on each institution's preference, since we wanted to evaluate radiation exposure on the basis of real clinical practice. With AEC, both CTDIvol and SSDE were higher in large-sized participants than in standard-sized participants. Two institutions acquired CT scans without AEC adaptation; they used the same tube current and voltage in large- and standard-sized participants. Consequently, neither CTDIvol nor SSDE differed significantly between the two body size groups. However, the SSDE/CTDIvol was significantly higher in the standard-sized participants than in the large-sized participants. These findings suggest that the SSDE/CTDIvol depends on body size, and that reference phantom-based CTDIvol values underestimate the radiation exposure associated with LDCT for lung cancer screening in relatively small-sized Korean candidates. The mean CTDIvol in the present study was 1.67 ± 0.60 mGy, which is much lower than the 3 mGy recommended by the ACR-AAPM. This reflects the lower radiation dose setting used in real practice for Korean population.
The risks associated with radiation exposure; including cancer resulting from cumulative exposure, vary depending on the subject's age of the screening, the number of scans received, and the individual's exposure to other sources of radiation. In the context of lung cancer screening CT, the subjects' ages range from 55 years to 74 years; they undergo LDCT examination every year and may receive additional CT scans depending on the LDCT results. This population is not radio-sensitive like children are, and various low-dose techniques are applied in screening CT. Nonetheless, dose reduction is important to minimize patient risk from cumulative radiation exposure in asymptomatic subjects. For this reason, many researchers are currently interested in further reducing the radiation dose of LDCT using advanced technologies such as AEC and iterative reconstruction. Using AEC, the CT tube current is automatically and dynamically adjusted in the x and y planes (angular modulation), in the z plane (z-axis modulation), or both, according to the size and attenuation of the body part being imaged. Thus, this process yields consistent image noise and quality throughout the examination, with lower radiation exposure (1112). Under the AEC system, a linear correlation between BMI and CTDIvol is expected. Indeed, in one previous study evaluating the relationship between radiation dose and body size under AEC adaptation, CTDIvol did increase linearly with patient body size (R2 = 0.480, p < 0.001), however, SSDE was independent of body size (R2 = 0.003, p = 0.300) (10). These findings are inconsistent with our own; there was correlation between SSDE and BMI as well as CTDIvol and BMI, although the correlation between SSDE and BMI was weak. These observations may have resulted from the AEC system settings used to adjust scanner output in patients of different sizes. For example, if the scanner output is markedly increased in larger patients, a positive correlation between SSDE and patient size would be observed.
The present study has several limitations that require consideration. First, the sample size was relatively small. Second, the CT scanning parameters and use of AEC adaptation differed among the institutions. Third, we did not analyze image noise or quality in terms of the scan parameters; however, in each case, the image quality was adequate as to be interpretable. Finally, we did not estimate either cumulative effective dose or radiation hazards such as the lifetime attributable risk of cancer in screening CT; the study population was too small to evaluate these parameters, and effective dose should not be reported in an individual.
In conclusion, CTDIvol based on a 32-cm diameter phantom may not adequately represent the actual radiation exposure of lung cancer screening LDCT in Korean population. Since average Korean individuals have small body size compared with the reference phantom, CTDIvol underestimates the radiation exposure associated with LDCT. Based on the findings from this pilot study, the maximum radiation dose recommendation of 3.0 mGy, based on a 32-cm reference phantom, might not appropriate for standardsized Korean participants. Further studies are required to verify optimal radiation dose limit for lung cancer screening LDCT in Korean population.
References
1. Kramer BS, Berg CD, Aberle DR, Prorok PC. Lung cancer screening with low-dose helical CT: results from the National Lung Screening Trial (NLST). J Med Screen. 2011; 18:109–111. PMID: 22045816.


2. Lee JW, Kim HY, Goo JM, Kim EY, Lee SJ, Kim TJ, et al. Radiological report of pilot study for the Korean Lung Cancer Screening (K-LUCAS) project: feasibility of implementing lung imaging reporting and data system. Korean J Radiol. 2018; 19:803–808. PMID: 29962887.


3. McNitt-Gray MF. AAPM/RSNA physics tutorial for residents: topics in CT. Radiation dose in CT. Radiographics. 2002; 22:1541–1553. PMID: 12432127.
4. WHO Expert Consultation. Appropriate body-mass index for Asian populations and its implications for policy and intervention strategies. Lancet. 2004; 363:157–163. PMID: 14726171.
5. Larke FJ, Kruger RL, Cagnon CH, Flynn MJ, McNitt-Gray MM, Wu X, et al. Estimated radiation dose associated with low-dose chest CT of average-size participants in the National Lung Screening Trial. AJR Am J Roentgenol. 2011; 197:1165–1169. PMID: 22021510.


6. Aberle DR, Gamsu G, Henschke CI, Naidich DP, Swensen SJ. A consensus statement of the Society of Thoracic Radiology: screening for lung cancer with helical computed tomography. J Thorac Imaging. 2001; 16:65–68. PMID: 11149694.
7. AAPM Task Group 23 of the Diagnostic Imaging Council CT Committee. The measurement, reporting and management of radiation dose in CT (Report No. 96). College Park, MD: American Association of Physicists in Medicine;2008.
8. AAPM Task Group 204. Size-specific dose estimates (SSDE) in pediatric and adult body CT examinations. College Park, MD: American Association of Physicists in Medicine;2008.
9. McCollough CH, Leng S, Yu L, Cody DD, Boone JM, McNitt-Gray MF. CT dose index and patient dose: they are not the same thing. Radiology. 2011; 259:311–316. PMID: 21502387.
10. Christner JA, Braun NN, Jacobsen MC, Carter RE, Kofler JM, McCollough CH. Size-specific dose estimates for adult patients at CT of the torso. Radiology. 2012; 265:841–847. PMID: 23091173.


11. Israel GM, Cicchiello L, Brink J, Huda W. Patient size and radiation exposure in thoracic, pelvic, and abdominal CT examinations performed with automatic exposure control. AJR Am J Roentgenol. 2010; 195:1342–1346. PMID: 21098193.


12. Mulkens TH, Bellinck P, Baeyaert M, Ghysen D, Van Dijck X, Mussen E, et al. Use of an automatic exposure control mechanism for dose optimization in multi-detector row CT examinations: clinical evaluation. Radiology. 2005; 237:213–223. PMID: 16126917.


Fig. 1
Body size measurement using low-dose screening chest CT. AP diameter and lateral diameter are measured at mid-liver level.
AP = antero-posterior
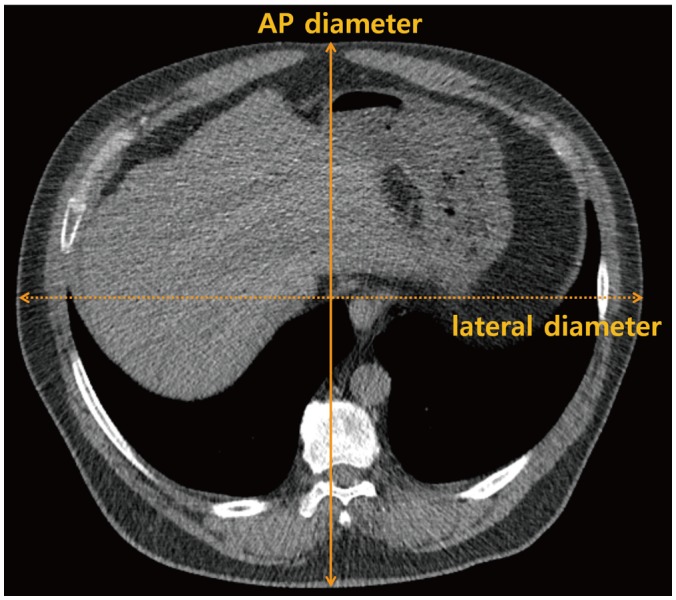
Fig. 2
Distribution of radiation dose estimates based on 32-cm diameter reference phantom (CTDIvol), SSDEs, and SSDE/CTDIvol according to BMI.
BMI = body mass index, CTDIvol = volume CT dose index, SSDE = size-specific dose estimate, SSDE/CTDIvol = ratio of SSDE to CTDIvol
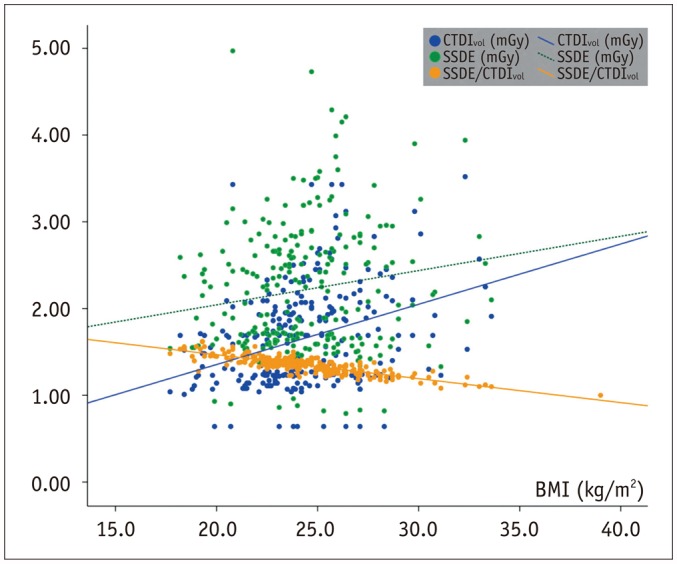
Fig. 3
Comparison of radiation dose estimates according to BMI and use of AEC.
A. Radiation dose estimate based on 32-cm diameter reference phantom (CTDIvol). B. SSDEs. C. SSDE/CTDIvol according to BMI and AEC. AEC = automatic exposure control, 1 = underweight and normal, 2 = overweight and obese
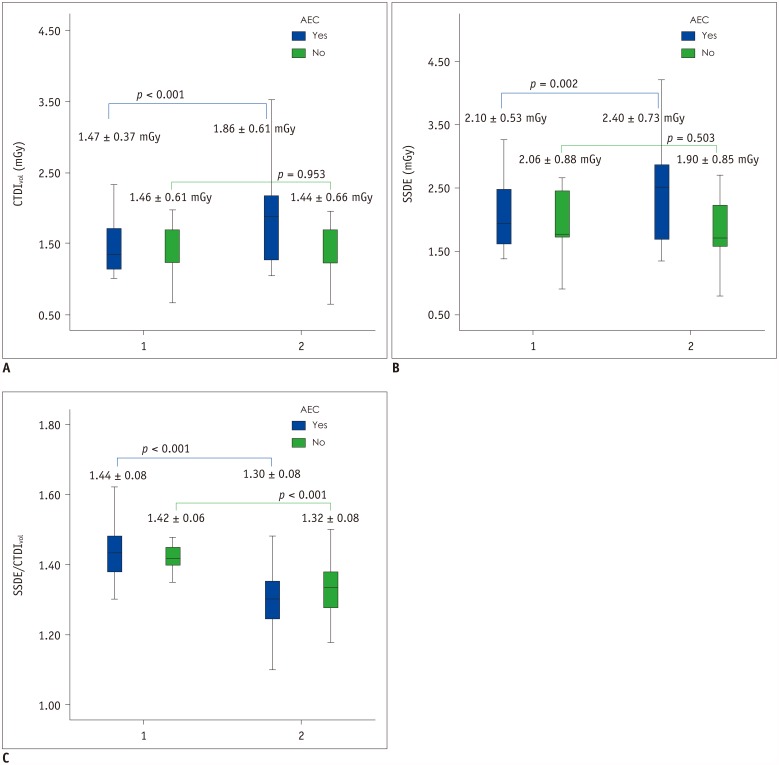
Table 1
Baseline Characteristics of Subjects Enrolled in K-LUCAS Project Pilot Study
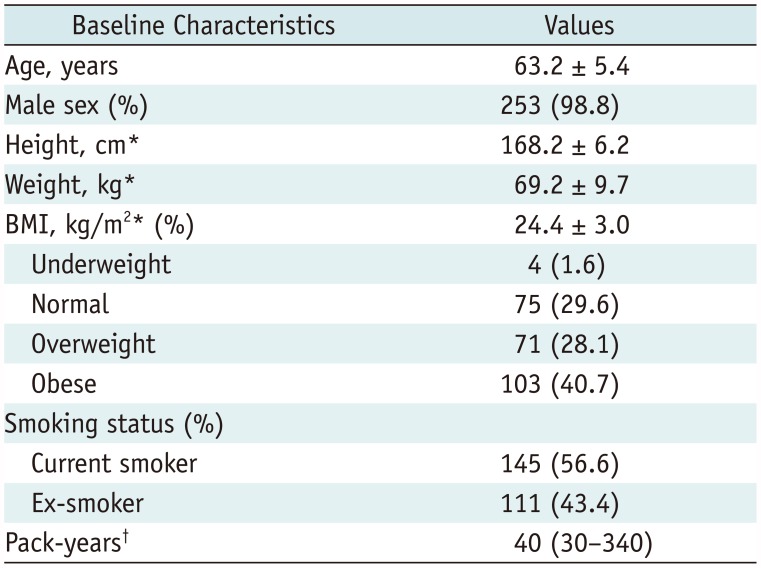
Table 2
Technical Parameters of CT Examinations in Pilot Study and Protocol Specifications in K-LUCAS Project
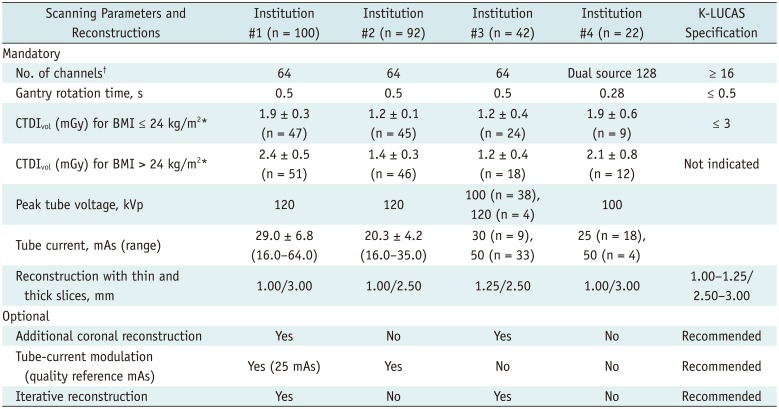
*BMI values were missing for three patients, †Scanners used: institution #1-Somatom Definition (n = 100), Siemens Healthineers, institution #2-Brilliance 64 (n = 92), Philips Healthcare; institution #3-GE Discovery (n = 32) and Revolution (n = 9), GE Healthcare and Somatom Definition (n = 1); institution #4-Somatom Definition Flash (n = 22), Siemens Healthineers. CTDIvol = volume CT dose index
Table 3
F-Sizes and Optimal Dose Distributions according to BMI
