Abstract
This study aimed to analyze the impact of sleep deprivation (SD) on cardiac, hemodynamic, and endothelial parameters and to determine whether these are sustained with increased periods of SD. The study included 60 healthy men (mean: age 31.2±6.3 years; body mass index 24.6±2.6 kg/m2). Hemodynamic parameters, parameters of myocardial contractility, spectral analysis of heart rate (HR) and blood pressure (BP) variability, and the sensitivity of arterial baroreflex function were evaluated. Biochemical tests were performed to assess L-arginine (L-Arg) and asymmetric dimethylarginine (ADMA) levels in reflection of endothelial nitric oxide synthase ability. Measurements of cardiovascular system parameters were obtained at 9 a.m. (baseline) on the first day of the study and 9 a.m. (24-h SD), 1 p.m. (28-h SD), and 5 p.m. (32-h SD) on the second day. Blood samples for evaluating biochemical parameters were obtained at baseline and after 24-h SD. ANOVA Friedman's test revealed a significant effect for time in relation to HR (χ2=26.04, df=5, p=0.000), systolic BP (χ2=35.98, df=5, p=0.000), diastolic BP (χ2=18.01, df=5, p=0.003), and mean BP (χ2=28.32, df=5, p=0.000). L-Arg and ADMA levels changed from 78.2±12.9 and 0.3±0.1 at baseline to 68.8±10.2 and 0.4±0.1 after 24-hr SD, respectively (p=0.001, p=0.004). SD in healthy men is associated with increases in BP, which appear to occur after 24 hours of SD and are maintained over increasing periods of SD. The observed hemodynamic changes may have resulted due to disordered vascular endothelial function, as reflected in alterations in L-Arg and ADMA levels.
Sleep loss, sleep deprivation (SD), and circadian misalignment have been studied for the past century and are known to have negative effects on metabolism, inflammatory system, and cardiovascular regulation. SD has been found to elicit several biological consequences, such as neural autonomic control changes, increased oxidative stress, or altered inflammatory responses. Additionally, epidemiological studies have confirmed that SD is associated with increases in blood pressure (BP)123 and adverse cardiovascular events. Moreover, short sleep duration has been found to be associated with increased coronary heart disease mortality and morbidity. However, the underlying pathophysiological mechanisms that underpin these associations have not been clearly demonstrated.
The autonomic nervous system plays an important role in the regulation of cardiovascular function, and impairment in this system is associated with increased cardiovascular mortality. It has been suggested that SD blunts cardiovascular autonomic responses, potentially explaining the excess mortality seen with SD.45 However, it is not clear what periods of SD are needed to influence cardiovascular regulation. Also, there is no commonly accepted definition of SD, including minimum sleep length: most studies define SD as a lack of for 24 to 60 hours. The main objective of this study was to analyze the impact of SD upon cardiac, hemodynamic, and endothelial factors and to determine whether these are sustained over increased periods of SD.
This study was approved by the Ethics Committee of Ludwik Rydygier Collegium Medicum in Bydgoszcz, Nicolaus Copernicus University in Torun, Poland (Approval number: 330/2013). We included 60 volunteers, all of whom were healthy men (mean: age 31.2±6.3 years; height 1.80±6.5 m, weight 80.5±10.9 kg; body mass index 24.6±2.6 kg/m2). All gave informed consent to participate in the study. The main enrollment criteria included male sex, no co-morbidity, no reported sleep disorders (Pittsburgh Sleep Quality Index <5), and no extreme chronotype (ratings between 14 and 21 points on the morning-evening M/E questionnaire). The exclusion criteria were shift work, addiction to alcohol, drug use, athlete at a competitive level, consumption of alcohol within 12 hours before study, taking any medicines or supplements during the study, and any cardiovascular disorders observed during the study. Clinical assessment of subjects comprised basic neurological, clinical examination, and evaluation of the autonomic nervous system using the Autonomic Symptom Profile. After physical examination, elevated BP was found in 20 subjects [systolic BP (sBP) ≥140 mm Hg, diastolic BP (dBP) ≥90 mm Hg; according to guidelines set by the European Society of Hypertension and European Society of Cardiology].
During the experiment, subjects stayed in the chronobiology laboratory (temperature 22℃, humidity 60%, light <10 lx) employing a constant routine. Subjects reported to the laboratory in the evening (9 p.m.) for an 8-h sleep adaptation episode. Additionally, the ActiGraph GT3X device (ActiGraph Corp, Pensacola, FL, USA) was used during the experiment to monitor the subjects' sleep night (total sleep time=417.7±24.9 min, sleep efficiency=95.5±3.0 min, and wake after sleep onset=19.3±13.1 min). Subjects ate the same meals at the same time of the day (8:00, 12:00, 15:00, and 19:30). Water (100 mL) was administered at hourly intervals. Subjects were cared for by trained personnel and remained awake for 24 hours.
Parameters of cardiovascular function were measured at four points during the study: at 9 a.m. (baseline) on the first day of the study and at 9 a.m. (24-h SD), 1 p.m. (28-h SD), and 5 p.m. (32-h SD) on the second day. Blood sampling for biochemical parameters took place twice: 9 a.m. (baseline) on the first day of the study and also at 9 a.m. after 24 hours of SD (Fig. 1).
Functional assessment of the autonomic nervous system was carried out in a non-invasive manner using the Task Force Monitor system (model 3040i by CNSystems Medizintechnik, Graz, Austria). The Task Force Monitor system provides an automated and computerized beat-to-beat analysis of impedance cardiography, electrocardiogram, and oscillometric and non-invasive continuous BP measurement. The system was used to assess hemodynamic parameters, parameters of myocardial contractility, parameters of spectral analysis of heart rate (HR) and BP variability, and parameters of the sensitivity of arterial baroreflex function. All functions of the Task Force Monitor have been validated prior to the study, and the instrument has been used successfully in numerous advanced clinical and scientific projects.67
Biochemical tests related to endothelial nitric oxide synthase ability were performed to investigate L-arginine (L-Arg) and asymmetric dimethylarginine (ADMA) levels in healthy subjects (immunoenzyme method, ELISA).
Normality of variable distribution was evaluated with the Shapiro-Wilk test, and the statistical characteristics thereof are presented as arithmetic means and standard deviations, as well as calculated minimum and maximum values. Depending on the distribution characteristics of analyzed variables, a paired-samples Student's t-test or Wilcoxon signed-rank test was used to evaluate the significance of differences between measured values obtained after normal night sleep and after SD. The effects of time on sBP, dBP, and mean BP (mBP) were evaluated by Friedman repeated measures ANOVA test due to non-normal distribution. All calculations were performed with Statistica 10 software (StatSoft, Inc., Tulsa, OK, USA), with an assumed level of statistical significance of α<0.05.
Although we initially recruited 60 healthy men volunteers for the study, 20 subjects were excluded after clinical evaluation because of elevated BP: measurement of BP was conducted twice at two different visits. We used beat-to-beat analysis of impedance cardiography, electrocardiogram, and non-invasive oscillometric and continuous BP measurement to observe autonomic regulation of the cardiovascular system (Fig. 2). Table 1 shows the mean results of cardiovascular and autonomic parameters at four points during the study. Friedman ANOVA test revealed a significant effect for time on HR (χ2=26.04, df=5, p=0.000), sBP (χ2= 35.98, df=5, p=0.000), dBP (χ2=18.01, df=5, p=0.003), and mBP (χ2=28.32, df=5, p=0.000) (Fig. 3). Mean values of BP were significantly higher after 24 hours of SD and were maintained over increasing periods of SD. There were no significant changes in cardiac function or spectral analysis of HR and BP variability over increased periods of SD. After 24-h SD, plasma levels of ADMA were significantly increased, while L-Arg levels were significantly decreased in comparison to those after normal night sleep (Table 2).
Overall, we noted that, during increased time periods of experimental SD, healthy men experience changes in HR and BP, without significant changes in the activity of the autonomic nervous system. The differences in hemodynamic parameters detected in this study are in keeping with previous reports that confirmed that the secondary effects of SD are increases in HR and in systolic, diastolic, and mean arterial BP. Numerous studies have shown that shorter sleep and experimental SD lasting 24, 36, or 40 hours is associated with an increase in BP in healthy subjects.8910111213 Interestingly, the changes in BP measures in this study appeared to occur after 24 hours of SD and were maintained over increasing periods of SD. However, HR reduced after 24 hours of SD, increasing after longer periods. This implies that the risks posed by SD are associated with the first 24 hours of SD and that additional periods of SD are not associated with increases in arterial pressure and hypertensive risk or with adverse effects on cardiovascular regulation.
The current literature on changes in cardiovascular and autonomic functioning during SD is not conclusive. Holmes, et al.14 reported that, during the 30 hours of wakefulness, a reduction in HR was mediated by a decline in cardiac sympathetic activity and the maintenance of cardiac parasympathetic activity. Zhong, et al.15 claimed that 36 hours of SD increases activity of the sympathetic autonomic nervous system, with a reduction in parasympathetic function and a decrease in baroreflex sensitivity. Other studies have shown that one night SD in the absence of significant additional stress does not lead to increased arterial pressure values or to changes in autonomic profile, merely the expected increase in tiredness.16
In the present study, observed hemodynamic changes may have resulted due to disordered vascular endothelial function, which was suggested by changes in biochemical parameters. Vascular endothelial disturbances resulting from an increased secretion of inflammatory mediators are another mechanism described in the literature to account for the changes in arterial BP. The endogenous vasodilating factor that plays a major role in maintaining the normal structure and function of vascular relaxation is nitric oxide (NO). For its continuous synthetization, NO is involved with endothelial NO synthase to convert L-Arg to NO and citrulline. Meanwhile, ADMA inhibits the formation of NO, thereby increasing the production of free oxygen radicals. The observed decreases in L-Arg and increases in ADMA levels suggest that the inhibition of the basal release of NO leads to the predominance of vasomotor factors, increased vascular resistance, and consequently an increase in BP.17181920 Recent studies have shown that acute SD induces a significant increase in circulating levels of endothelial cell activation markers and pro- and anti-inflammatory markers.2122 Moreover, it has been reported that a high level of interleukin-6 or E-selectin could be linked to the development of endothelial dysfunction and cardiovascular disease. Sauvet, et al.10 demonstrated that experimental SD without behavioral and environmental stress is not a sufficient factor to stimulate the autonomic nervous system and that the first changes to occur are in plasma concentrations of markers of endothelial cell activation and an increase in E-selectin and interleukin-6.
One limitation of this study was lack of an objective sleep measure, such polysomnographic recording before the experiment. Future research applying standardized conditions on a larger scale is needed to clarify the detrimental effects of chronic SD in a real life model.
In conclusion, SD in healthy men is associated with increases in BP occurring after 24 hours of SD and maintained over increasing periods of SD. This implies that the risks of SD are associated with 24 hours of SD and that additional periods of SD are not associated with subsequent increases in arterial pressure and hypertensive risk or with negative effects on autonomic BP regulation.
References
1. Gangwisch JE. A review of evidence for the link between sleep duration and hypertension. Am J Hypertens. 2014; 27:1235–1242. PMID: 24778107.


2. Javaheri S, Storfer-Isser A, Rosen CL, Redline S. Sleep quality and elevated blood pressure in adolescents. Circulation. 2008; 118:1034–1040. PMID: 18711015.


3. Verdecchia P, Angeli F, Borgioni C, Gattobigio R, Reboldi G. Ambulatory blood pressure and cardiovascular outcome in relation to perceived sleep deprivation. Hypertension. 2007; 49:777–783. PMID: 17261645.


4. Cincin A, Sari I, Oğuz M, Sert S, Bozbay M, Atas¸ H, et al. Effect of acute sleep deprivation on heart rate recovery in healthy young adults. Sleep Breath. 2015; 19:631–636. PMID: 25319876.


5. Mullington JM, Haack M, Toth M, Serrador JM, Meier-Ewert HK. Cardiovascular, inflammatory, and metabolic consequences of sleep deprivation. Prog Cardiovasc Dis. 2009; 51:294–302. PMID: 19110131.


6. Fortin J, Klinger T, Wagner Ch, Sterner H, Madritsch Ch, Grullenberger R. The task force monitor–a non-invasive beat-to-beat monitor for hemodynamic and autonomic function of the human body. In : Proceedings of the 20th Annual International Conference of the IEEE Engineering in Medicine and Biology Society; 29 October–1 November, 1998; Hong Kong.
7. Fortin J, Marte W, Grüllenberger R, Hacker A, Habenbacher W, Heller A, et al. Continuous non-invasive blood pressure monitoring using concentrically interlocking control loops. Comput Biol Med. 2006; 36:941–957. PMID: 16483562.


8. Franzen PL, Gianaros PJ, Marsland AL, Hall MH, Siegle GJ, Dahl RE, et al. Cardiovascular reactivity to acute psychological stress following sleep deprivation. Psychosom Med. 2011; 73:679–682. PMID: 21949422.


9. Robillard R, Lanfranchi PA, Prince F, Filipini D, Carrier J. Sleep deprivation increases blood pressure in healthy normotensive elderly and attenuates the blood pressure response to orthostatic challenge. Sleep. 2011; 34:335–339. PMID: 21358850.


10. Sauvet F, Leftheriotis G, Gomez-Merino D, Langrume C, Drogou C, Van Beers P, et al. Effect of acute sleep deprivation on vascular function in healthy subjects. J Appl Physiol (1985). 2010; 108:68–75. PMID: 19910332.


11. Ohira T, Tanigawa T, Iso H, Odagiri Y, Takamiya T, Shimomitsu T, et al. Effects of shift work on 24-hour ambulatory blood pressure and its variability among Japanese workers. Scand J Work Environ Health. 2000; 26:421–426. PMID: 11103841.


12. Gottlieb DJ, Redline S, Nieto FJ, Baldwin CM, Newman AB, Resnick HE, et al. Association of usual sleep duration with hypertension: the Sleep Heart Health Study. Sleep. 2006; 29:1009–1014. PMID: 16944668.


13. Yamasaki F, Schwartz JE, Gerber LM, Warren K, Pickering TG. Impact of shift work and race/ethnicity on the diurnal rhythm of blood pressure and catecholamines. Hypertension. 1998; 32:417–423. PMID: 9740605.


14. Holmes AL, Burgess HJ, Dawson D. Effects of sleep pressure on endogenous cardiac autonomic activity and body temperature. J Appl Physiol (1985). 2002; 92:2578–2584. PMID: 12015376.
15. Zhong X, Hilton HJ, Gates GJ, Jelic S, Stern Y, Bartels MN, et al. Increased sympathetic and decreased parasympathetic cardiovascular modulation in normal humans with acute sleep deprivation. J Appl Physiol (1985). 2005; 98:2024–2032. PMID: 15718408.


16. Pagani M, Pizzinelli P, Traon AP, Ferreri C, Beltrami S, Bareille MP, et al. Hemodynamic, autonomic and baroreflex changes after one night sleep deprivation in healthy volunteers. Auton Neurosci. 2009; 145:76–80. PMID: 19006684.


17. Calvin AD, Covassin N, Kremers WK, Adachi T, Macedo P, Albuquerque FN, et al. Experimental sleep restriction causes endothelial dysfunction in healthy humans. J Am Heart Assoc. 2014; 3:e001143. PMID: 25424573.


18. Frey DJ, Fleshner M, Wright KP Jr. The effects of 40 hours of total sleep deprivation on inflammatory markers in healthy young adults. Brain Behav Immun. 2007; 21:1050–1057. PMID: 17524614.


19. Shearer WT, Reuben JM, Mullington JM, Price NJ, Lee BN, Smith EO, et al. Soluble TNF-alpha receptor 1 and IL-6 plasma levels in humans subjected to the sleep deprivation model of spaceflight. J Allergy Clin Immunol. 2001; 107:165–170. PMID: 11150007.
20. Vaara J, Kyröläinen H, Koivu M, Tulppo M, Finni T. The effect of 60-h sleep deprivation on cardiovascular regulation and body temperature. Eur J Appl Physiol. 2009; 105:439–444. PMID: 19002705.


21. Sauvet F, Drogou C, Bougard C, Arnal PJ, Dispersyn G, Bourrilhon C, et al. Vascular response to 1 week of sleep restriction in healthy subjects. A metabolic response? Int J Cardiol. 2015; 190:246–255. PMID: 25932797.
22. Sauvet F, Florence G, Van Beers P, Drogou C, Lagrume C, Chaumes C, et al. Total sleep deprivation alters endothelial function in rats: a nonsympathetic mechanism. Sleep. 2014; 37:465–473. PMID: 24587568.


Fig. 1
Study protocol. Measurements of cardiovascular system parameters were obtained at four points during the study: on the first day of the study (after adaptive night) and after 24, 28, and 32 hours of SD. Blood sampling for biochemical parameters took place twice: on the first day of the study and after 24 hours of SD. SD, sleep deprivation; BP, blood pressure.
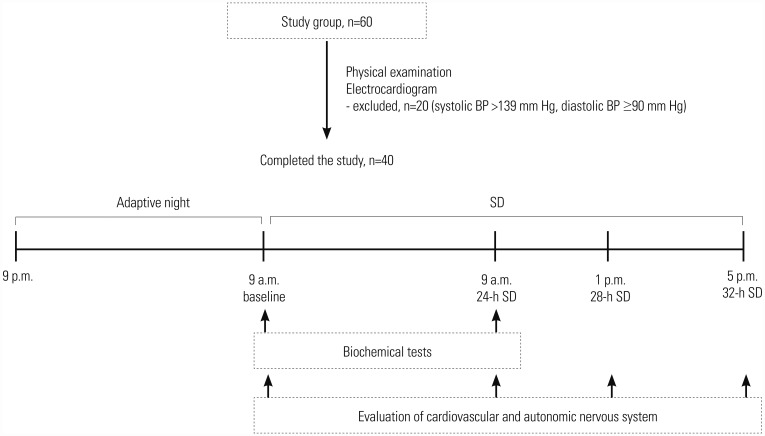
Fig. 2
Representative beat-to-beat analysis of impedance cardiography, electrocardiogram, and non-invasive continuous blood pressure measurement observed in one patient. HR, heart rate; SI, stroke index; CI, cardiac index; IC, index of contractility; TPRI, total peripheral resistance index; sBP, systolic blood pressure; dBP, diastolic blood pressure; mBP, mean blood pressure; LVWI, left ventricular work index; LVET, left ventricular ejection time; TFC, thoracic fluid content.
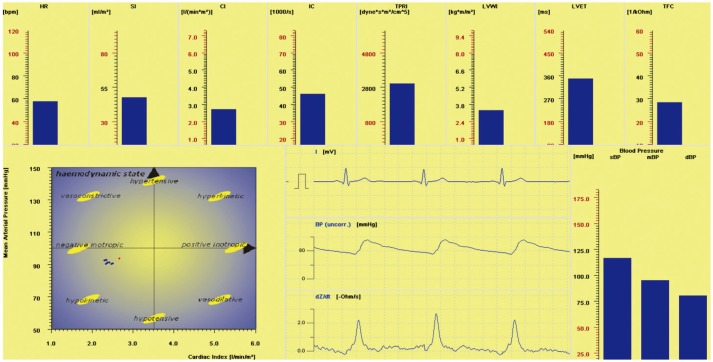
Fig. 3
Mean values of HR, sBP, dBP, and mBP after 24-h SD, 28-h SD, and 32-h SD. Statistical analysis showed a significant effect for time on these parameters. SD, sleep deprivation; HR, heart rate; sBP, systolic blood pressure; dBP, diastolic blood pressure; mBP, mean blood pressure.
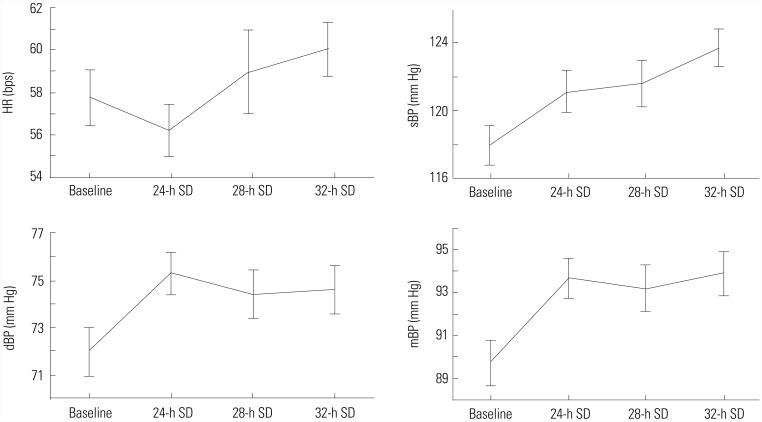
Table 1
Mean Values of Cardiovascular and Autonomic Parameters after 24, 28, and 32 Hours of SD
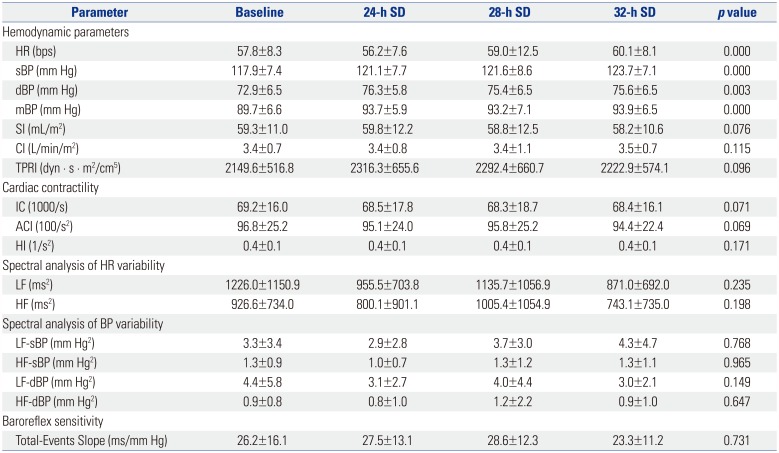
SD, sleep deprivation;HR, heart rate; sBP, systolic blood pressure; dBP, diastolic blood pressure; mBP, mean blood pressure; SI, stroke index; CI, cardiac index; TPRI, total peripheral resistance index; IC, index of contractility; ACI, acceleration index; HI, Heather index; HF, high-band frequency spectrum; LF, low-band frequency spectrum.