Abstract
Purpose
Exposure to indoor radon is associated with lung cancer. This study aimed to estimate the number of lung cancer deaths attributable to indoor radon exposure, its burden of disease, and the effects of radon mitigation in Korea in 2010.
Materials and Methods
Lung cancer deaths due to indoor radon exposure were estimated using exposure-response relations reported in previous studies. Years of life lost (YLLs) were calculated to quantify disease burden in relation to premature deaths. Mitigation effects were examined under scenarios in which all homes with indoor radon concentrations above a specified level were remediated below the level.
Results
The estimated number of lung cancer deaths attributable to indoor radon exposure ranged from 1946 to 3863, accounting for 12.5–24.7% of 15623 total lung cancer deaths in 2010. YLLs due to premature deaths were estimated at 43140–101855 years (90–212 years per 100000 population). If all homes with radon levels above 148 Bq/m3 are effectively remediated, 502–732 lung cancer deaths and 10972–18479 YLLs could be prevented.
Radon is a colorless, odorless, and tasteless radioactive gas that is produced by the decay of natural uranium and radium in rocks and soils throughout the earth's crust. Inhalation of radon can damage DNA and enhance the risk of lung cancer by depositing alpha particles emitted during the radioactive decay of radon in lung epithelium.1 In 1988, the International Agency for Research on Cancer, a cancer research institute of the World Health Organization (WHO), declared radon to be carcinogenic for humans and classified it as a proven human carcinogen.2
Radon is the most important natural source of ionizing radiation for the general population. Most individuals are exposed to radon indoors, since radon can accumulate in confined spaces such as residential homes, while radon is quickly diluted outdoors by atmospheric mixing.3 This suggests that indoor radon is a great threat to public health. Indeed, among the risk factors of lung cancer, indoor radon is the second leading cause after smoking in many countries.4 Epidemiological studies conducted in Europe, North America, and China have confirmed exposure to indoor radon as increasing the risk of lung cancer among the general population.56789 The proportion of lung cancer deaths attributable to radon exposure is estimated to be between 3% and 20% worldwide.10 The global burden of lung cancer due to residential radon is also considerable, accounting for 2.0 million disability-adjusted life years (DALY) in 2013.11
Indoor radon is a preventable risk factor that can be mitigated by installing effective measures for reducing radon concentrations in buildings. Accordingly, action levels for indoor radon concentrations have been proposed in many countries. However, the majority of radon-induced lung cancer deaths are caused by exposure to radon at concentrations lower than recommended action levels, since most of general population are exposed to low and moderate level of indoor radon.4 This implies that more appropriate action levels and preventive measures to reduce exposure to indoor radon may benefit public health. For this, comprehensive analyses are needed to ascertain the population risk and disease burden of lung cancer deaths attributable to indoor radon exposure, as well as the expected benefits of radon mitigation. Therefore, we aimed to estimate the number of lung cancer deaths due to indoor radon exposure and the burden thereof on premature mortalities in Korea. Furthermore, preventive effects of radon mitigation on lung cancer deaths were investigated under scenarios in which all homes above a specified radon concentration were remediated below the level.
A nationwide survey of indoor radon concentration levels has been performed by the National Institute of Environmental Research in Korea since 2008. Radon concentrations in homes were periodically investigated from 2010 to 2014, including approximately 21000 households. Sample sizes within each administrative district were determined using census data. Households were selected using random sampling methods according to building type. Passive nuclear alpha track detectors (Raduet Model RSV-8, Radosys Ltd., Budapest, Hungary) measured indoor radon concentrations for 3 months during the winter season.
We used radon data that were collected in 2011/2012 and 2013/2014. A total of 15507 households participated in the survey during these periods. Since indoor radon concentrations are known to be the highest in winter and the lowest in summer, correction for seasonal variations was required to estimate annual average concentrations. Lee, et al.12 developed a model to correct for seasonal variations in indoor radon concentrations in Korea. The authors calculated the seasonal correction factors by applying a model previously developed by Pinel, et al.13 to a Korean database of indoor radon measurements. Using the Korean model, we estimated annual averages of indoor radon concentrations. Distribution of the estimated average indoor radon concentrations appeared to be log normal, ranging from 7.0 Bq/m3 to 2258.5 Bq/m3. Arithmetic mean (±SD) was 92.0±104.2 Bq/m3, and median value was 62.5 Bq/m3. Geometric mean (±SD) was 67.9±2.1 Bq/m3. Indoor radon concentrations varied across 16 administrative districts (Fig. 1).
Data on lung cancer deaths in 2010 were obtained from the Statistics Korea. Deaths due to lung cancer were defined as cases coded as C33–C34 by the International Classification of Diseases, 10th Revision. For 2010, 15623 people died of lung cancer in Korea (11416 men and 4207 women). Population data were obtained through the 2010 census conducted by the Statistics Korea. Information on the proportion of ever-smokers among lung cancer cases was obtained from a large prospective Korean cohort study.14 Out of all lung cancer cases, 81% of men and 14% of women were either past or current smokers.
Data analysis was performed in three stages. First, we estimated the number of lung cancer deaths attributable to indoor radon exposure in Korea. Second, years of life lost (YLLs) were calculated to determine the burden of premature mortality due to indoor radon-induced lung cancer. In the third stage, we estimated the number of lung cancer deaths and YLLs that could be prevented from reducing indoor radon concentrations.
The number of lung cancer deaths attributable to indoor radon exposure was estimated using the following formula:
where NAR is the number of lung cancer deaths attributable to indoor radon exposure; ERR is the excess relative risk of lung cancer death due to indoor radon; and Ntotal is the total number of lung cancer deaths. Calculations were carried out by age, sex, smoking status (ever- and never-smokers), and administrative districts. In the formula, ERR was estimated based on the exposure-response relations determined in two epidemiological studies performed by the sixth Biological Effects on Ionizing Radiations (BEIR VI) Committee1 and Darby, et al.5 They are briefly described below.
In 1999, BEIR VI Committee proposed two models for lung cancer risk due to radon exposure by reanalyzing a combined analysis of 11 cohorts of miners in 1994.15 ERR estimated by the models represents multiplicative increment in excess lung cancer risk due to radon above background levels. ERR is a linear function of cumulative exposure, and it varies according to attained age, time since exposure, and either the duration of exposure [Exposure-Age-Duration (EAD) model] or the level of concentration [Exposure-Age-Concentration (EAC) model]. Both models are as follows:
where β is the slope parameter of exposure-response relation; w5–14, w15–24, and w25+ are the exposures incurred at 5–14, 15–24, and 25 years or more before the current age, respectively; and θ15–24 and θ25+ are the relative contributions to risk from exposures at 15–24 and 25 years or more before the attained age. The parameters Φage and γz represent the effect modification factors of multiple categories of attained age, and either exposure rate in EAC model or exposure duration in EAD model. Since tobacco is the leading cause of lung cancer, BEIR Committee investigated the interaction between smoking and indoor radon exposure on lung cancer risk. The interaction appeared to be submultiplicative, and the committee proposed using a factor of 2 for ERR calculation among nonsmokers, and a factor of 0.9 among smokers. We used these interaction factors to estimate ERRs separately for ever- and never-smokers. As for age and sex, BEIR Committee assumed that ERR per unit exposure did not vary with age at exposure and sex, and we followed these assumptions.
Darby, et al.5 conducted a joint analysis of individual data from 13 European case-control studies to determine the risk of lung cancer associated with residential radon exposure.5 The collaboration included 7148 cases of lung cancer and 14208 controls. The authors estimated ERR of lung cancer due to indoor radon exposure using the following model:
where β is the slope parameter of exposure-response relation, and X is the mean radon concentration in homes occupied over the 5–34 years before study enrollment. This model is adjusted for possible confounders such as study, age, sex, region of residence, and smoking status. In terms of effect modification, they found no significant differences in ERR according to age, sex and smoking status. Based on results of the collaborative study, we used the same value of β for strata separated according to those factors.
Applying the models proposed by BEIR VI and Darby, et al., we assumed that all individuals in the same district were equally exposed to the average concentration of indoor radon, and that the population was exposed to the same concentration level for their entire life.
In the second stage, we determined YLLs due to lung cancer deaths attributable to indoor radon exposure. YLL is a fatal component of burden of disease measuring the years of life lost due to premature mortality. YLL calculations were based on the methods of the WHO for Global Burden of Disease (GBD) in 2013.16 YLLs are calculated as the sum of the number of age-specific deaths and the standard life expectancy at the age at which death occurs. In the present study, the number of deaths corresponded to estimates of lung cancer deaths due to indoor radon for 5-year age intervals stratified by sex, district, and smoking status, which were derived from analyses in the first stage. Standard life expectancy was referred to the WHO report for GBD 2013.16 YLL rates were calculated as YLLs divided by the number of population according to sex and smoking status, and expressed as YLLs per 100000 population.
Finally, we examined the preventive effects of mitigating indoor radon concentrations on lung cancer deaths. For this analysis, cut-offs of radon concentrations in homes were chosen as 74, 148, 200, and 300 Bq/m3, based on the Korean action level of 148 Bq/m3. Analysis was performed in three steps. First, since complete elimination of indoor radon exposure is not practical, radon concentrations in all homes at or above 74, 148, 200, and 300 Bq/m3 were assigned a random value, between 0 and cut-points, to represent potential mitigation of indoor radon concentrations. Second, with the newly created radon data, we estimated the number of indoor radon-attributable lung cancer deaths and YLLs from premature deaths. These calculations were conducted by applying the methods mentioned above in the first and second stages. Third, prevented number of lung cancer deaths and saved YLLs were calculated by subtracting the number of lung cancer deaths and YLLs derived under the mitigation scenario from those estimated in the first and second stages, respectively. For analysis of these three steps, 1000 iterations were performed to account for variability in the random assignment of mitigated indoor radon concentrations.
Table 1 shows the estimated number of lung cancer deaths attributable to indoor radon exposure by sex and smoking status in Korea in 2010. Depending on the dose-response relationship model used, our analyses indicated that 1946–3863 lung cancer deaths were attributable to indoor radon exposure, regardless of sex and smoking status. These estimates suggest that of the 15623 lung cancer deaths in 2010, 12.5–24.7% may have been caused by indoor radon exposure. As for smoking status, lung cancer deaths due to indoor radon exposure occurred more among ever-smokers than among never-smokers (1230–2014 and 716–1849, respectively). However, for women, the majority of radon-attributable lung cancer deaths occurred among never-smokers (445–1111 for never-smokers and 73–108 for ever-smokers), since the proportion of female smokers is very low in Korea. The attributable percentages by smoking status varied depending on the risk model used, due to different assumptions on the interaction between smoking and radon. Under BEIR VI models, the percentage was lower for ever-smokers than for never-smokers (15.4–20.5% and 26.0–32.0%), whereas, with Darby, et al.'s model, indoor radon exposure attributed to lung cancer deaths similarly among everand never-smokers (12.5% and 12.4%). Regardless of smoking status, the estimated numbers of lung cancer deaths associated with residential radon were 1428–2644 in men and 518–1219 in women. These calculations suggest that indoor radon accounts for 12.5–23.2% and 12.3–29.0% of total lung cancer deaths in men and women, respectively. After stratification by smoking status, there were no obvious differences in the attributable percentages between men and women.
Table 2 outlines YLLs due to premature deaths caused by lung cancers attributable to indoor radon exposure in Korea in 2010, based on three different risk models. Regardless of sex and smoking status, total YLLs ranged from 43140 to 101855 years, and YLL rates varied from 90 to 212 years per 100000 population. YLLs for ever- and never-smokers were 27711–53960 years (122–238 years per 100000 population) and 15429–47859 years (61–189 years per 100000 population), respectively. According to sex, YLLs were 32332–70293 years in men and 10808–31562 years in women, which corresponds to 136–295 YLLs and 45–131 YLLs per 100000 male and female populations, respectively.
Figs. 2 and 3 report the effects of indoor radon mitigation on lung cancer deaths. The total number of preventable lung cancer deaths estimated ranged from 502 [standard error (SE), 0.1] to 732 (0.1) if all homes in Korea at or above 148 Bq/m3 were effectively remediated below this level (Fig. 2). The estimate suggests that 3.2–4.7% of all lung cancer deaths may be prevented annually in Korea. The reduced number of lung cancer deaths could potentially save 10972 (SE, 1.8)–18479 (3.2) years from being lost (Fig. 3). According to smoking status, the number of and YLLs from radon-attributable lung cancer deaths could be reduced by 318–447 deaths and 7065–11394 years among ever-smokers and by 184–343 deaths and 3907–8075 years among never-smokers. After lowering the mitigation level to 74 Bq/m3, the overall estimated number of preventable lung cancer deaths nearly doubled (970–1491), accounting for 6.2–9.5% of total lung cancer deaths. Additional YLLs could also be saved by as many as 10349–19897 years.
The present study was conducted to quantify the public health hazard and disease burden from lung cancer deaths that are attributable to indoor radon exposure, and to underscore the necessity of a national strategy for mitigating indoor radon concentrations. For Korea, we estimated that 12.5–24.7% of all lung cancer deaths in 2010 were attributable to indoor radon exposure. Furthermore, we found that those premature deaths inflicted a considerable disease burden, with an estimated 43140–101855 YLLs during that year. Finally, our findings indicated that effective mitigation strategies aimed at lowering indoor radon concentrations could help reduce lung cancer deaths and disease burden. This holistic approach may be meaningful in establishing effective strategies for indoor radon control by providing comprehensive information to authorities.
There are considerable variations in radon levels between and within countries,4 as radon concentrations depend on several factors including the local geology, building type and materials, ventilation practices, season, and activities of occupants.171819 The variation in radon levels could lead to differences in the proportions of lung cancer deaths due to indoor radon exposure among countries, with a positive correlation between both.10 In the present study, the average indoor radon concentration in Korea was 92.0 Bq/m3, which is higher than average levels in the U.S.1 and European countries.172021 Accordingly, compared to these countries, our estimates of the attributable percentage of indoor radon for lung cancer deaths were relatively high.
Estimates of attributable risk are dependent on the exposure-response models used. The attributable risk based on EAD model is generally estimated to be about 30% lower compared to that based on EAC model, possibly due to inherent differences between parameters used in both models.1 In an epidemiological study in the U.S., the proportions of lung cancer deaths attributable to indoor radon exposure were estimated at 13.9% in EAC model and 9.8% in EAD model.1 The same trend was observed in other studies conducted in France,17 Portugal,19 and Korea.18 When applying the model proposed by Darby, et al.,5 we observed the fewest deaths from radon-induced lung cancer, similar to other studies.17,18 This discrepancy may have resulted as BEIR VI models tend to overestimate the effects of indoor radon on lung cancer, due to overestimation of relevant exposure in older age groups and the lack of correction for uncertainties in radon distributions.21
The interaction between tobacco smoking and radon was accounted for differently in the models of BEIR VI and Darby, et al.5 in our analyses. BEIR VI Committee assumed a submultiplicative interaction of smoking and radon based on their analyses using miner data with smoking history.1 Meanwhile, Darby, et al.5 found that there was no effect modification by smoking on the association between radon and lung cancer in their analyses. This discrepancy leads to different pattern of attributable percentage according to smoking status between the models of BEIR VI and Darby, et al.5: estimated attributable percentages of lung cancer deaths were higher for never-smokers than for ever-smokers in BEIR VI models, whereas similar percentages were recorded between ever- and never-smokers in Darby, et al.5 model.
To our knowledge, the present study is the first attempt to measure the disease burden for radon-attributable lung cancer in Korea. In a Korean population-based study, the burden of disease was measured for environmental risks and associated diseases.22 The study reported that a total of 1798 DALYs per 100000 population were related to environmental diseases, and that YLLs accounted for about 30% of total DALYs. Considering our estimation that 90–212 years per 100000 population were lost due to indoor radon-related lung cancer deaths, lung cancer due to indoor radon exposure may occupy a large proportion of the environmental burden of disease in Korea. A study for six European countries reported that indoor radon is a leading risk factor associated with environmental burden of disease, and radon-related lung cancer showed high impacts on public health.23
In the U.S., mitigation level of indoor radon concentration is the same as that in Korea at 148 Bq/m3.24 A study in the U.S. estimated that remediating indoor radon concentrations in all homes below 148 Bq/m3 could reduce lung cancer mortality by 3.7%.1 The study further reported that if mitigation level was lowered to 74 Bq/m3, 6.5% of all lung cancer deaths could be prevented every year. These estimates are similar to ours with a 3.2–4.7% reduction in lung cancer deaths at 148 Bq/m3 and 6.2–9.5% at 74 Bq/m3. In Germany and Canada, reducing indoor radon concentrations to outdoor levels for homes above 200 Bq/m3 was estimated to prevent 15.9% and 28.4% of all lung cancer deaths attributable to indoor radon per year, respectively.3,21 The estimates were higher than ours, since their results were calculated under more intensive scenario of reducing indoor radon concentrations to the outdoor level, whereas our scenario remediated to random values below the cut-off level. In addition, the authors calculated the preventable percentage among total radon-related lung cancer deaths not all-cause lung cancer mortalities. If we calculated the percentage of preventable lung cancer deaths using their methods, our estimates would increase to 13.4–18.5%.
The present study used lung cancer mortality as an outcome in exposure-response models. For this reason, the estimation of disease burden was confined to YLLs, and it was impossible to calculate years lost due to disability for people living with lung cancer or its consequences. The vast majority of studies applying the models of BEIR VI Committee and Darby, et al. have also only used mortality data for estimating the attributable risk of indoor radon on lung cancer.1317181920212526 This might be due to relatively easier availability of mortality data rather than morbidity data. Moreover, since the risk models, especially BEIR VI models, are based on lung cancer death as an outcome, it remains uncertain whether it is possible to use incidence or prevalence data in the models. Intuitively, the coefficient values would likely be different between risk models based on morbidity and those based on mortality. This suggests that it is necessary to investigate whether the morbidity and mortality models are interchangeable.
Estimation of attributable risk is subject to a number of uncertainties. The uncertainties mainly come from radon exposure misclassification and the inherent weaknesses of risk models used.10 Radon concentrations within homes vary by seasons. Therefore, correction for season is needed to obtain the annual average concentration levels. Annual average radon concentrations are also subject to substantial random year-to-year variations related to numerous factors, including weather patterns.4 Indoor radon concentrations can vary between houses, and even from room to room in the same house. These spatial variations depend on several factors such as the soil, building materials, house type, and ventilation practices.527 Measurement errors also introduce uncertainties in estimating indoor radon concentrations. Some detectors (e.g., open detectors) are unable to distinguish between radon and thoron, thereby providing misleading information concerning radon concentrations. Raduet detector used in the national radon survey in Korea detects radon and thoron activity via two chambers; main chamber is selective for radon activity. Localization of detectors within a home is one of the main sources of measurement error. To address this methodological issue, the Korean survey of indoor radon concentrations followed a standard protocol for installment of detectors. The risk models proposed by BEIR VI Committee were derived from data of miner cohorts to extrapolate the models to general populations. However, there are appreciable differences between underground miners and general populations. In aspects of the environment, radon concentrations are much higher in mines than in homes, and not only radon but also other carcinogens, such as arsenic, can exist in the underground mines. In addition, there are differences in the characteristics of subjects of two populations, including sex and age distributions as well as smoking habits.1 Since these uncertainties are difficult to be characterized,25 many studies have had to assume that the lung cancer risk due to radon exposure in homes is close to that observed among miners.10
In the present study, the proportion of ever-smokers among lung cancer cases was based on findings from a previous epidemiological study, in which 81% of men and 14% of women were ever-smokers.14 The individuals who participated in the study were government employees and teachers, and they tended to be middle-class, more educated, and healthier compared to the general population in Korea. This may lead to a lower smoking prevalence than that in the general population. Moreover, the prevalence of smoking among female lung cancer patients in Korea is very low. This could have resulted from the relatively low overall prevalence of smoking among Korean women compared to that of women from other countries.28 In addition, Korean female smokers tend to be reluctant in honestly answering questions about their smoking status, due to the social atmosphere in which tobacco smoking is not considered acceptable for women.
Analyses in the present study were performed under the assumption of a constant exposure to radon over one's lifetime. However, for each individual, exposure is likely to be modified by various physical and biological factors such as ventilation flow, breathing frequency, tracheobronchial configuration, and body size.19 Residential mobility can also influence estimates of radon-related lung cancer risk.29 Nevertheless, this is a common limitation in population-based analyses on radon exposure, as considering individual characteristics would be impossible in practice.
The present study had other limitations. We used data on indoor radon concentrations, population, and lung cancer deaths for 16 metropolitan cities and provinces. Data at this large spatial scale are unable to capture small scale variability, and could have affected uncertainties and accuracies of estimates. Moreover, we used dose-response relationships proposed from data of other countries due to the lack of a Korean model. The dose-response relationship in other countries could differ from that in South Korea, which may influence our estimates. Further study is needed to investigate dose-response relationships between indoor radon and lung cancer using a Korean database.
Strategies for radon control are needed to achieve an overall risk reduction in the population. Indoor radon control includes prevention during the construction of new dwellings and mitigation for existing dwellings. Several techniques, such as active/passive soil depressurization, ventilation, sealing of surfaces, barriers and membranes, and water treatment, can be used to prevent and reduce indoor radon concentrations. For successful and cost-effective prevention and mitigation actions, these control options must be adapted to building types and characteristics, radon sources, and transport mechanisms.4
In summary, exposure to indoor radon attributes considerably to lung cancer deaths in Korea. Such premature deaths pose a critical threat to public health, increasing the burden of the disease. Our findings suggest that reducing indoor radon concentrations would be helpful for decreasing lung cancer deaths. Since indoor radon is a modifiable risk factor, exposure to the substance can be controlled through effective strategies for remediation. Although the Korean Ministry of Environment has developed radon control guidelines, it is not mandatory. A more aggressive indoor radon control, based on strong and evidence-based strategies, is needed to promote public health.
ACKNOWLEDGEMENTS
This work was supported by grants 2015001350002 from the Korean Ministry of Environment as part of the “Environmental Health Action Program,” and the faculty research fund of Ajou University School of Medicine.
References
1. National Research Council. Health effects of exposure to radon: BEIR VI. Washington, DC: National Academy Press;1999.
2. International Agency for Research on Cancer. Man-made mineral fibres. Man-made mineral fibres. IARC Monogr Eval Carcinog Risks Hum. 1988; 43:39–171. PMID: 3065211.
3. Chen J, Moir D, Whyte J. Canadian population risk of radon induced lung cancer: a re-assessment based on the recent cross-Canada radon survey. Radiat Prot Dosimetry. 2012; 152:9–13. PMID: 22874897.


4. World Health Organization. WHO handbook on indoor radon: a public health perspective. Geneva: World Health Organization;2009.
5. Darby S, Hill D, Auvinen A, Barros-Dios JM, Baysson H, Bochicchio F, et al. Radon in homes and risk of lung cancer: collaborative analysis of individual data from 13 European case-control studies. BMJ. 2005; 330:223. PMID: 15613366.


6. Darby S, Hill D, Deo H, Auvinen A, Barros-Dios JM, Baysson H, et al. Residential radon and lung cancer--detailed results of a collaborative analysis of individual data on 7148 persons with lung cancer and 14,208 persons without lung cancer from 13 epidemiologic studies in Europe. Scand J Work Environ Health. 2006; 32(Suppl 1):1–83.
7. Krewski D, Lubin JH, Zielinski JM, Alavanja M, Catalan VS, Field RW, et al. Residential radon and risk of lung cancer: a combined analysis of 7 North American case-control studies. Epidemiology. 2005; 16:137–145. PMID: 15703527.
8. Krewski D, Lubin JH, Zielinski JM, Alavanja M, Catalan VS, Field RW, et al. A combined analysis of North American case-control studies of residential radon and lung cancer. J Toxicol Environ Health A. 2006; 69:533–597. PMID: 16608828.


9. Lubin JH, Wang ZY, Boice JD Jr, Xu ZY, Blot WJ, De Wang L, et al. Risk of lung cancer and residential radon in China: pooled results of two studies. Int J Cancer. 2004; 109:132–137. PMID: 14735479.


10. Kim SH, Hwang WJ, Cho JS, Kang DR. Attributable risk of lung cancer deaths due to indoor radon exposure. Ann Occup Environ Med. 2016; 28:8. PMID: 26925236.


11. GBD 2013 Risk Factors Collaborators. Forouzanfar MH, Alexander L, Anderson HR, Bachman VF, Biryukov S, Brauer M, et al. Global, regional, and national comparative risk assessment of 79 behavioural, environmental and occupational, and metabolic risks or clusters of risks in 188 countries, 1990-2013: a systematic analysis for the Global Burden of Disease Study 2013. Lancet. 2015; 386:2287–2323. PMID: 26364544.
12. Lee CM, Kang DY, Koh SB, Cho YS, Lee DJ, Lee SB. Development of predictive model for annual mean radon concentration for assessment of annual effective dose of radon exposure. J Environ Sci Int. 2016; 25:1107–1114.


13. Pinel J. Fearn T, Darby SC, Miles JCH. Seasonal correction factors for indoor radon measurements in the United Kingdom. Radiat Prot Dosimetry. 1995; 58:127–132.
14. Yun YD, Back JH, Ghang H, Jee SH, Kim Y, Lee SM, et al. Hazard ratio of smoking on lung cancer in Korea according to histological type and gender. Lung. 2016; 194:281–289. PMID: 26718701.


15. Lubin JH, Boice JD, Edling C, Hornung RW, Howe G, Kunz E. Radon and lung cancer risk: a joint analysis of 11 underground miners studies. Bethesda (MD): National Institutes of Health;1994.
16. World Health Organization. WHO methods and data sources for global burden of disease estimates 2000-2011. Geneva: World Health Organization;2013.
17. Catelinois O, Rogel A, Laurier D, Billon S, Hemon D, Verger P, et al. Lung cancer attributable to indoor radon exposure in France: impact of the risk models and uncertainty analysis. Environ Health Perspect. 2006; 114:1361–1366. PMID: 16966089.


18. Lee HA, Lee WK, Lim D, Park SH, Baik SJ, Kong KA, et al. Risks of lung cancer due to radon exposure among the regions of Korea. J Korean Med Sci. 2015; 30:542–548. PMID: 25931783.


19. Veloso B, Nogueira JR, Cardoso MF. Lung cancer and indoor radon exposure in the north of Portugal--an ecological study. Cancer Epidemiol. 2012; 36:e26–e32. PMID: 22075535.
20. Advisory Group on Ionising Radiation. Radon and public health. Oxford: Health Protection Agency;2009.
21. Menzler S, Piller G, Gruson M, Rosario AS, Wichmann HE, Kreienbrock L. Population attributable fraction for lung cancer due to residential radon in Switzerland and Germany. Health Phys. 2008; 95:179–189. PMID: 18617799.


22. Yoon SJ, Kim HS, Ha J, Kim EJ. Measuring the environmental burden of disease in South Korea: a population-based study. Int J Environ Res Public Health. 2015; 12:7938–7948. PMID: 26184265.


23. Hänninen O, Knol AB, Jantunen M, Lim TA, Conrad A, Rappolder M, et al. Environmental burden of disease in Europe: assessing nine risk factors in six countries. Environ Health Perspect. 2014; 122:439–446. PMID: 24584099.


24. Lubin JH, Boice JD Jr. Estimating Rn-induced lung cancer in the United States. Health Phys. 1989; 57:417–427. PMID: 2777548.


25. Brand KP, Zielinski JM, Krewski D. Residential radon in Canada: an uncertainty analysis of population and individual lung cancer risk. Risk Anal. 2005; 25:253–269. PMID: 15876202.


26. Peterson E, Aker A, Kim J, Li Y, Brand K, Copes R. Lung cancer risk from radon in Ontario, Canada: how many lung cancers can we prevent? Cancer Causes Control. 2013; 24:2013–2020. PMID: 23982909.


27. Darby S, Hill D, Doll R. Radon: a likely carcinogen at all exposures. Ann Oncol. 2001; 12:1341–1351. PMID: 11762803.


28. Organisation for Economic Co-operation and Development. OECD health statistics 2016. accessed on 2016 November 9. Available at: http://www.oecd.org/els/health-systems/health-data.htm.
29. Warner KE, Courant PN, Mendez D. Effects of residential mobility on individual versus population risk of radon-related lung cancer. Environ Health Perspect. 1995; 103:1144–1149. PMID: 8747021.


Fig. 2
Lung cancer deaths that could be prevented if all homes above the mitigation levels of radon concentration were remediated. Calculations were performed based on dose-response relationships proposed by BEIR VI Committee and Darby, et al. (A) BEIR VI-EAC, (B) BEIR VI-EAD, and (C) Darby, et al. Values at the right side of bars indicate the number of preventable lung cancer deaths (standard error). BEIR VI, the sixth Biological Effects on Ionizing Radiations; EAD, Exposure-Age-Duration; EAC, Exposure-Age-Concentration.
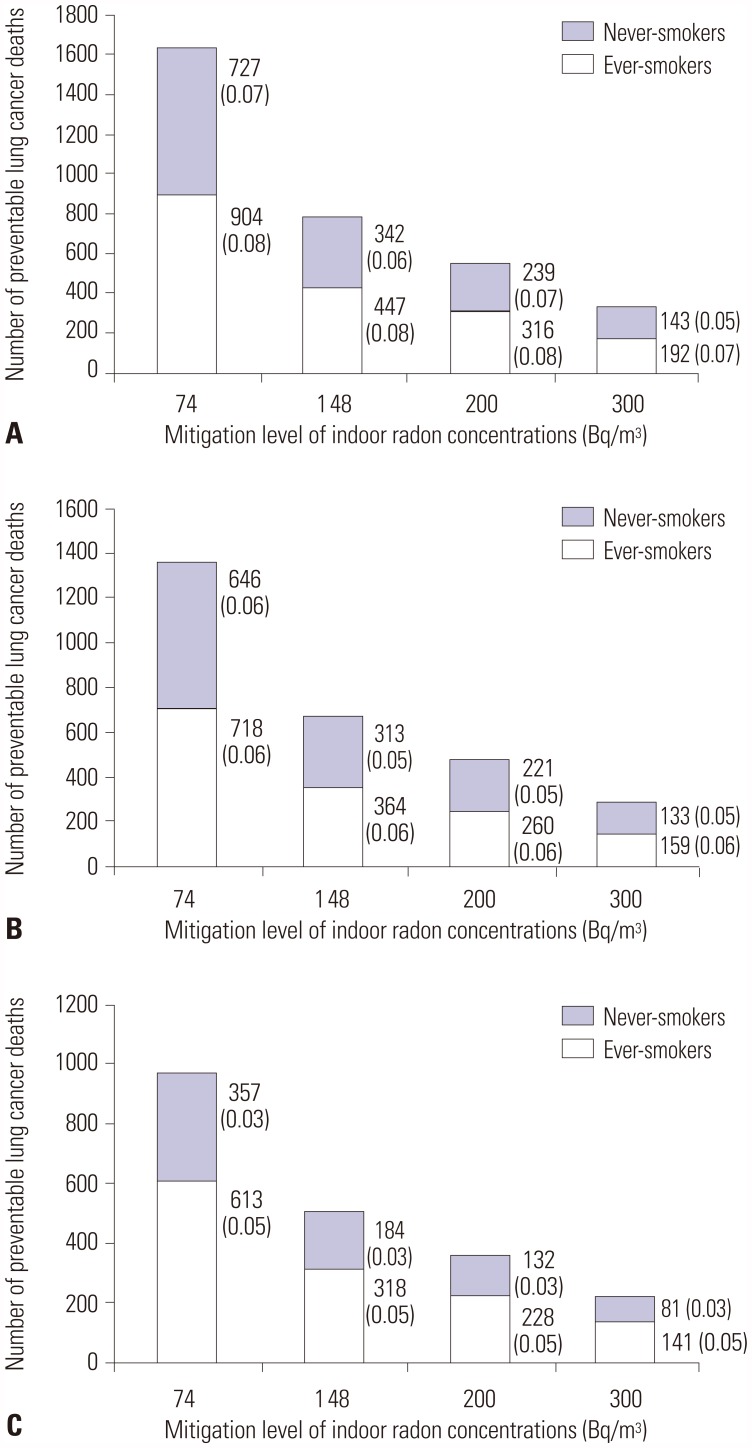
Fig. 3
Years of life lost (YLLs) due to lung cancer deaths that could be saved if all homes above the mitigation levels of radon concentration were remediated. Calculations were performed based on dose-response relationships proposed by BEIR VI Committee and Darby, et al. (A) BEIR VI-EAC, (B) BEIR VI-EAD, and (C) Darby, et al. Values at the right side of bars indicate YLLs (standard error). BEIR VI, the sixth Biological Effects on Ionizing Radiations; EAD, Exposure-Age-Duration; EAC, Exposure-Age-Concentration.
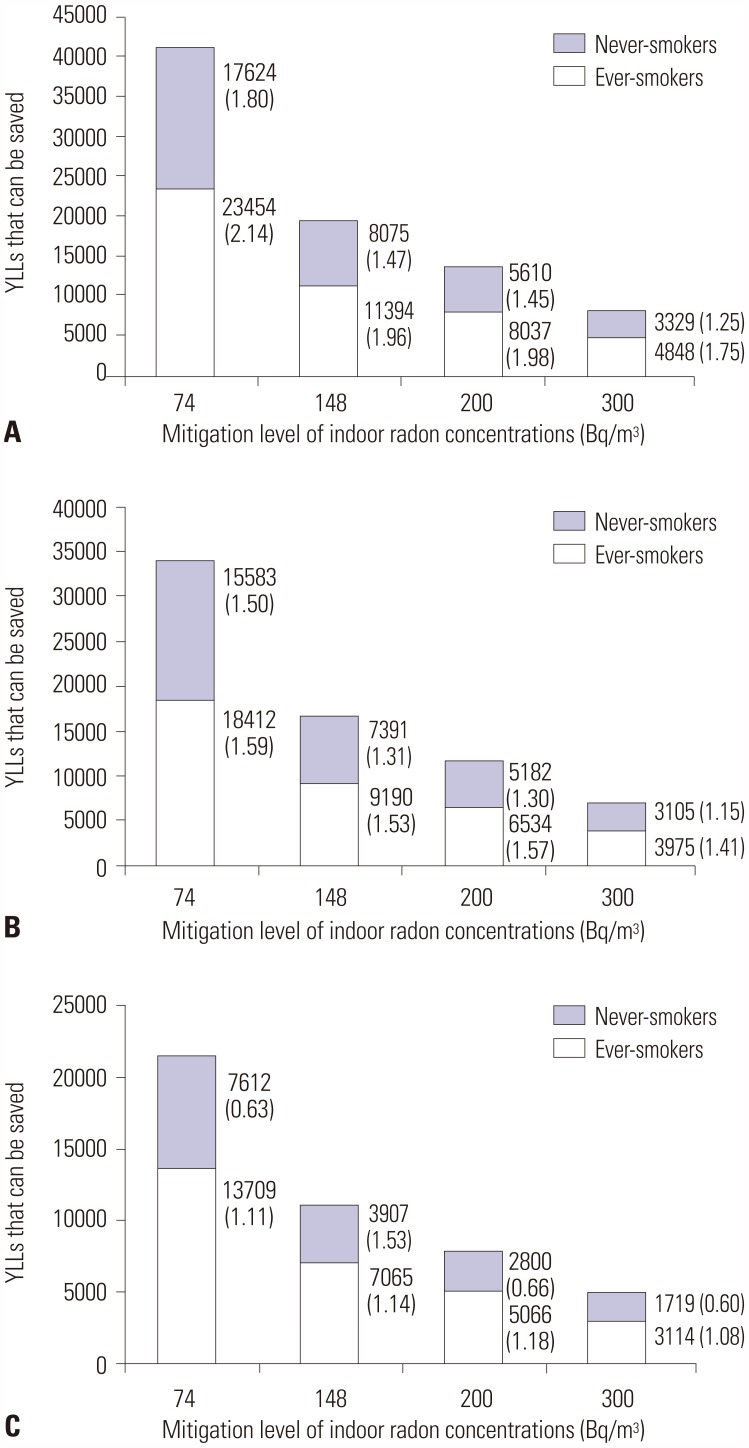
Table 1
Number (Percentage) of Lung Cancer Deaths Attributable to Indoor Radon Exposure in Korea in 2010

Dose-response relationships | Ever-smokers | Never-smokers | Combined | ||||||
---|---|---|---|---|---|---|---|---|---|
Men | Women | Total | Men | Women | Total | Men | Women | Total | |
BEIR VI-EAC | 1906 (20.6) | 108 (18.3) | 2014 (20.5) | 738 (34.0) | 1111 (30.7) | 1849 (32.0) | 2644 (23.2) | 1219 (29.0) | 3863 (24.7) |
BEIR VI-EAD | 1430 (15.5) | 85 (14.4) | 1515 (15.4) | 589 (27.2) | 917 (25.3) | 1506 (26.0) | 2019 (17.7) | 1002 (23.8) | 3021 (19.3) |
Darby, et al.5 | 1157 (12.5) | 73 (12.4) | 1230 (12.5) | 271 (12.5) | 445 (12.3) | 716 (12.4) | 1428 (12.5) | 518 (12.3) | 1946 (12.5) |
Table 2
Years of Life Lost from Lung Cancer Deaths Attributable to Indoor Radon Exposure in Korea in 2010

Dose-response relationships | Ever-smokers | Never-smokers | Combined | ||||||
---|---|---|---|---|---|---|---|---|---|
Men | Women | Total | Men | Women | Total | Men | Women | Total | |
BEIR VI-EAC | 51045 (264) | 2915 (86) | 53960 (238) | 19248 (425) | 28647 (138) | 47895 (189) | 70293 (295) | 31562 (131) | 101855 (212) |
BEIR VI-EAD | 37593 (195) | 2198 (65) | 39791 (175) | 15140 (334) | 23033 (111) | 38173 (151) | 52733 (221) | 25231 (104) | 77964 (162) |
Darby, et al.5 | 26189 (136) | 1522 (45) | 27711 (122) | 6143 (136) | 9286 (45) | 15429 (61) | 32332 (136) | 10808 (45) | 43140 (90) |