Abstract
Figures and Tables
Table 1
Anti-inflammatory effects of extract and fractions on LPS-stimulated bone marrow-derived dendritic cells

a The inhibitory effects are represented as giving 50% inhibition (IC50) relative to the vehicle control. These data represent the average values of three repeated experiments (mean ± SD). IC50 values for selected extracts are given in column IL-12 p40, IL-6 and TNF-α. Values < 25 µM are considered to be active.
b SB203580 was used as a positive control.
Table 2
Anti-inflammatory effects of compounds on LPS-stimulated bone marrow-derived dendritic cells
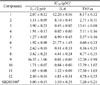
a The inhibitory effects are represented as giving 50% inhibition (IC50) relative to the vehicle control. These data represent the average values of three repeated experiments (mean ± SD). IC50 values for selected extracts are given in column IL-12 p40, IL-6 and TNF-α. Values < 25 µM are considered to be active.
b SB203580 was used as a positive control.