Abstract
Artemisia capillaris has been widely used as an alternative therapy for treating obesity and atopic dermatitis. It has been used as a hepatoprotactant. It is also used for ameliorating inflammatory reactions. Although there are several investigations on other Artemisia species, there is no systematic study describing the role of A. capillaris MeOH extract, its solvent soluble fractions, or derived anti-inflammatory principal components in regulating inflammatory conditions. Therefore, the objective of this study was to elucidate anti-inflammatory mechanisms of A. capillaris. Results revealed that MeOH extract of A. capillaris could decrease LPS-stimulated NO secretion. Of tested fractions, CH2Cl2, EtOAc, and n-BuOH strongly inhibited NO release from RAW264.7 cells. Bioactive mediators derived from CH2 Cl2 and n-BuOH fractions elicited potent anti-inflammatory actions and strikingly abrogated LPS-triggered NO accumulation in RAW264.7 cells. Of particular interest, capillin and isoscopoletin possessed the most potent NO suppressive effects. Western blot analysis validated the molecular mechanism of NO inhibition and showed that capillin and isoscopoletin significantly down-regulated iNOS and COX-2 protein expression. Taken together, our results provide the first evidence that MeOH extract, CH2Cl2, EtOAc, and n-BuOH fractions from A. capillaris and its derived lead candidates can potently suppress inflammatory responses in macrophages by hampering NO release and down-regulating iNOS and COX-2 signaling.
References
(1). Murakami M., Hirano T.Front. Immunol. 2012; 3:1–2.
(2). Sharma J. N., Al-Omran A., Parvathy S. S.Inflammopharmacology. 2007; 15:252–259.
(3). Bognar E., Sarszegi Z., Szabo A., Debreceni B., Kalman N., Tucsek Z., Sumegi B., Gallyas F.Jr. PLoS One. 2013; 8:e65355.
(4). Rhule A., Navarro S., Smith J. R., Shepherd D. M. J.Ethnopharmacol. 2006; 106:121–128.
(5). Tang W., Eisenbrand G.Chinese drugs of plant origin, chemistry, phamacology and use in traditional and modern medicine: Springer Verlag, New York. 1992; 179.
(6). Hong J. H., Lee J. W., Park J. H., Lee I. S.Biofactors. 2007; 31:43–53.
(7). Seo K. S., Yun K. W.Korean J. Plant Res. 2008; 21:292–298.
(8). Lee C. J., Kim H. Y., Kim J. D., Kim C. H. J.Korean Orient. Med. 2000; 21:100–107.
(9). Choi J. H., Kim D. W., Yun N., Choi J. S., Islam M. N., Kim Y. S., Lee S. M. J.Nat. Prod. 2011; 74:1055–1060.
(10). Seo K. S., Jeong H. J., Yun K. W. J.Ecol. Field Biol. 2010; 33:141–147.
(11). Cha J. D., Moon S. E., Kim H. Y., Cha I. H., Lee K. Y. J.Food Sci. 2009; 74:75–81.
(13). Okuno I., Uchida K., Kadowaki M., Akahori A. Jpn. J.Pharmacol. 1981; 31:835–838.
(14). Jung H. A., Park J. J., Islam M. N., Jin S. E., Min B. S., Lee J. H., Sohn H. S., Choi J. S.Arch. Pharm. Res. 2012; 35:1021–1035.
(15). Kwon O. S., Choi J. S., Islam M. N., Kim Y. S., Kim H. P.Arch. Pharm. Res. 2011; 34:1561–1569.
(16). Nurul Islam M., Jung H. A., Sohn H. S., Kim H. M., Choi J. S.Arch. Pharm. Res. 2013; 36:542–552.
(17). Pautz A., Art J., Hahn S., Nowag S., Voss C., Kleinert H.Nitric Oxide. 2010; 23:75–93.
(18). Ribiere C., Jaubert A. M., Gaudiot N., Sabourault D., Marcus M. L., Boucher J. L., Denis-Henriot D., Giudicelli Y.Biochem. Biophys. Res. Commun. 1996; 222:706–712.
(19). Mitrovic B., Ignarro L. J., Montestruque S., Smoll A., Merrill J. E.Neuroscience. 1994; 61:575–585.
(20). Kawachi H., Moriya N. H., Korai T., Tanaka S. Y., Watanabe M., Matsui T., Kawada T., Yano H.Mol. Cell. Biochem. 2007; 300:61–67.
67.(21) Rayburn E. R.., Ezell S. J.., Zhang R.Mol. Cell Pharmacol. 2009. 1:29–43.
(22). Mulabagal V., Alexander-Lindo R. L., Dewitt D. L., Nair M. G.Evid. Based Complement. Alternat. Med. 2011; 11:1–6.
(23). Yan Y., Li J., Ouyang W., Ma Q., Hu Y., Zhang D., Ding J., Qu Q., Subbaramaiah K., Huang C. J.Cell Sci. 2006; 119:2985–2994.
(25). Burk D. R., Cichacz Z. A., Daskalova S. M. J.Med. Plant. Res. 2010; 4:225–234.
(26). Valles J., Torrell M., Garnatje T., Garcia-Jacas N., Vilatersana R., Susanna A.Plant Biol. 2003; 5:274–284.
(27). Lim H. K., Cho S. K., Park S., Cho M. J.Korean Soc. Appl. Biol. Chem. 2010; 53:275–282.
(28). Jang M., Jeong S. W., Kim B. K., Kim J. C.BioMed. Res. Int. 2015; 2015:872718.
(29). Ali M. Y., Jannat S., Jung H. A., Choi R. J., Roy A., Choi J. S. Asian Pac. J.Trop. Med. 2016; 9:103–111.
(30). Han J., Zhao Y. L., Shan L. M., Huang F. J., Xiao X. H. Chin. J.Integr. Med. 2005; 11:54–56.
(31). Kim E. K., Kwon K. B., Han M. J., Song M. Y., Lee J. H., Lv N., Choi K. B., Ryu D. G., Kim K. S., Park J. W., Park B. H.Int. J. Mol. Med. 2007; 19:535–540.
(32). Kim Y. S., Bahn K. N., Hah C. K., Gang H. I., Ha Y. L.J. Food Sci. 2008; 73:16–20.
(33). Janbaz K. H., Gilani A. H.J. Ethnopharmacol. 1995; 47:43–47.
(34). Benli M., Kaya I., Yigit N.Cell Biochem. Funct. 2007; 25:681–686.
(35). Shahriyary L., Yazdanparast R.J. Ethnopharmacol. 2007; 114:194–198.
(36). Aniya Y., Shimabukuro M., Shimoji M., Kohatsu M., Gyamfi M. A., Miyagi C., Kunii D., Takayama F., Egashira T.Biol. Pharm. Bull. 2000; 23:309–312.
(37). Zhang Z., Guo S. S., Zhang W. J., Geng Z. F., Liang J. Y., Du S. S., Wang C. F., Deng Z. W.Ind. Crops Prod. 2017; 100:132–137.
(38). Islam M. N., Choi R. J., Jung H. A., Oh S. H., Choi J. S.Arch. Pharm. Res. 2016; 39:340–349.
(39). Tanaka K.Seikagaku. 1961; 33:399–409.
(40). Masuda Y., Asada K., Satoh R., Takada K., Kitajima J.Phytomedicine. 2015; 22:545–552.
(41). Jihed B., Bhouri W., Ben Sghaier M., Bouhlel I., Kriffi M., Skandrani I., Dijoux F. M., Ghedira K., Chekir-Ghedira L.Nutr. Cancer. 2012; 64:1095–1102.
(42). Kiso Y., Ogasawara S., Hirota K., Watanabe N., Oshima Y., Konno C., Hikino H.Planta Med. 1984; 50:81–85.
Fig. 2.
Effects of MeOH extract on cell viability and LPS-induced nitrite formation in RAW264.7 cells. Cell viability was measured by MTT assay.
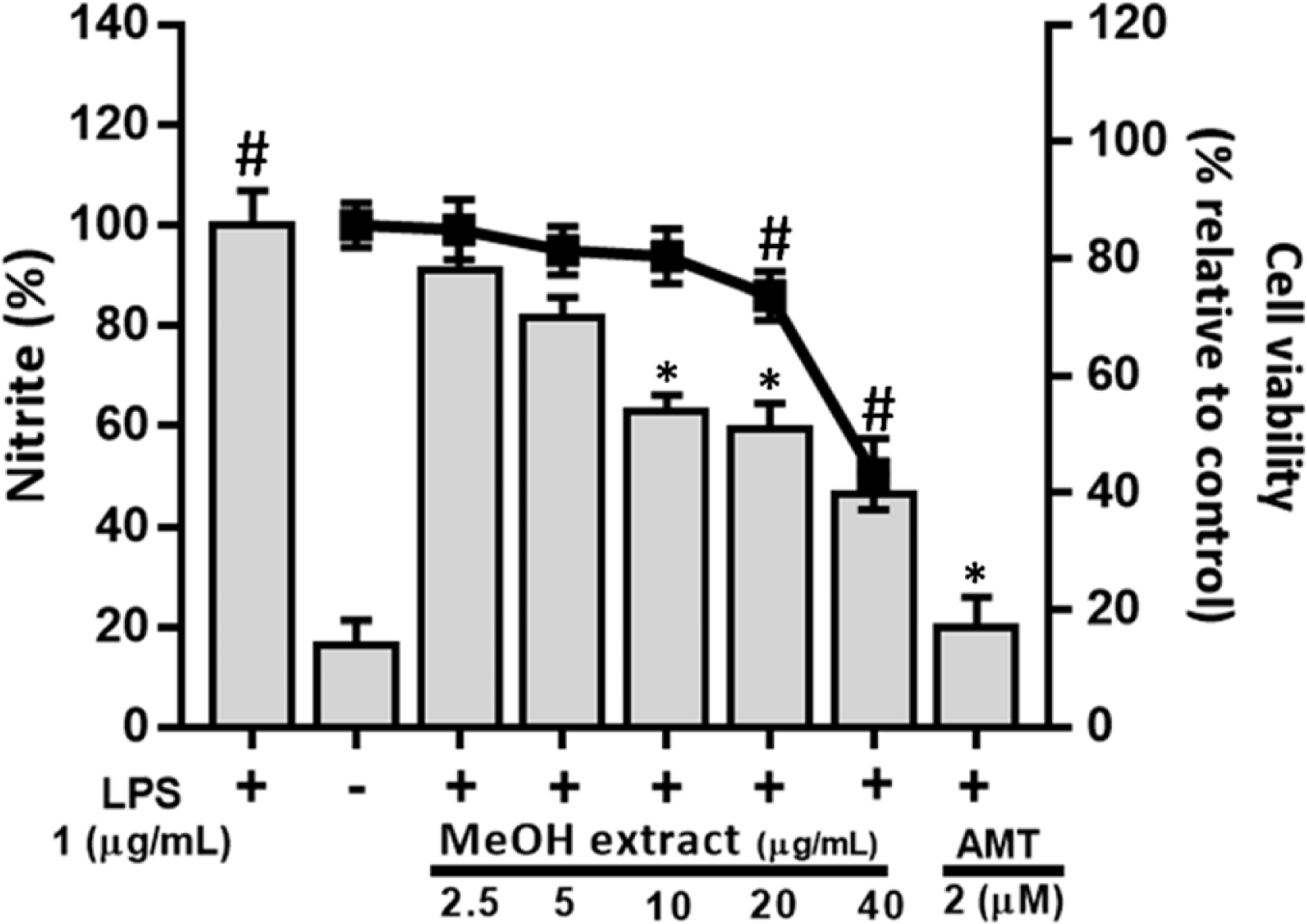
Fig. 3.
Effects of solvent soluble fractions of MeOH extract from Artemisia capillaris on cell viability (a) and LPS-induced nitrite formation (b) in RAW264.7 cells. Cells were pre-treated with different concentrations (10, 50 and 100 μg/mL) of CH2Cl2 (C), EtOAc (E), BuOH (B), or H2 O (H) fraction of MeOH extract from Artemisia capillaris followed by treatment with LPS (1 μg/ mL) and incubation for 18 h.
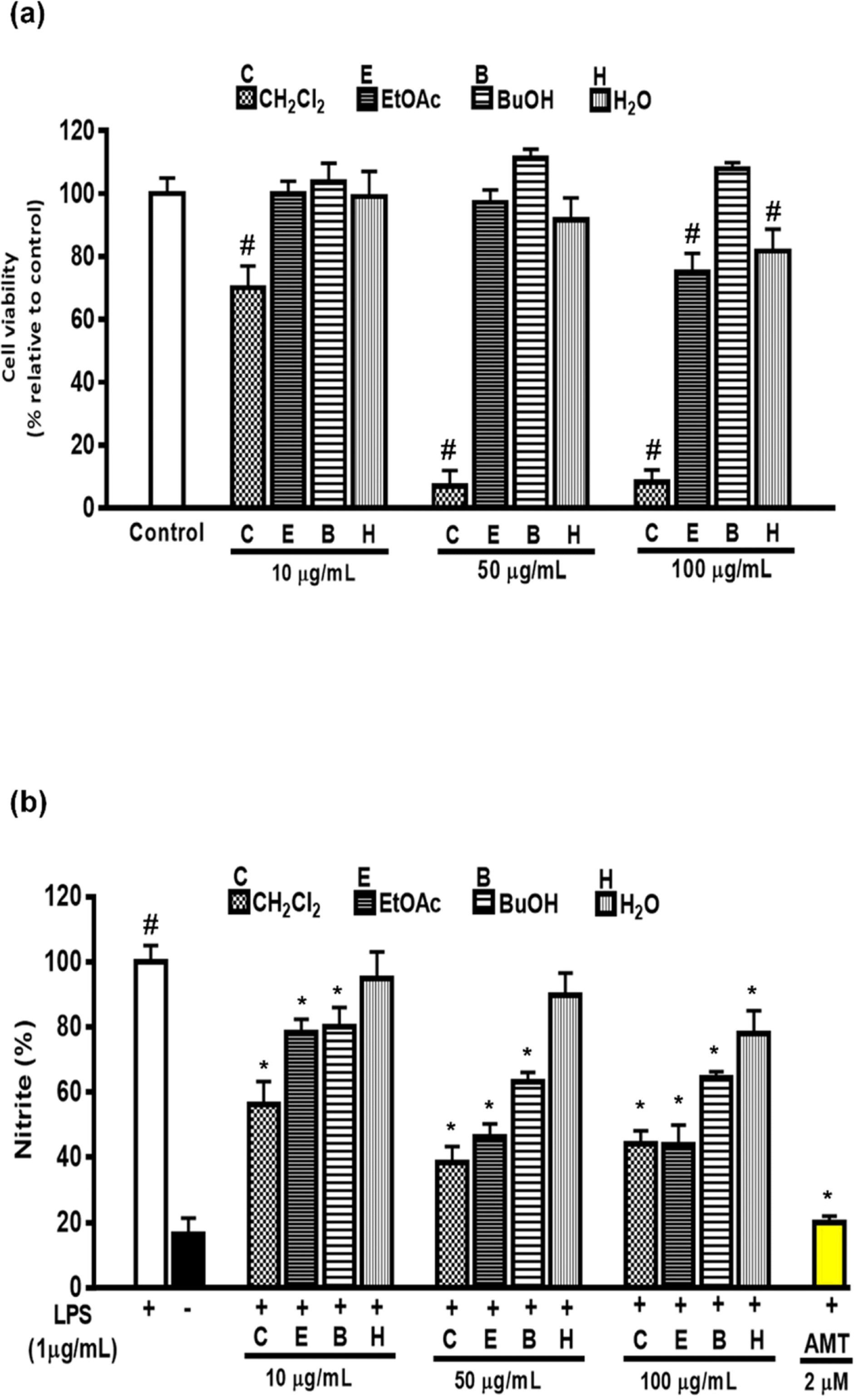
Fig. 4.
Effects of capillin (a), scopoletin (b), isoscopoletin (c), scopolin (d), isoscopolin (e), or scoparone (f) isolated from Artemisia capillaris on cell viability and LPS-induced NO production in RAW264.7 cells.
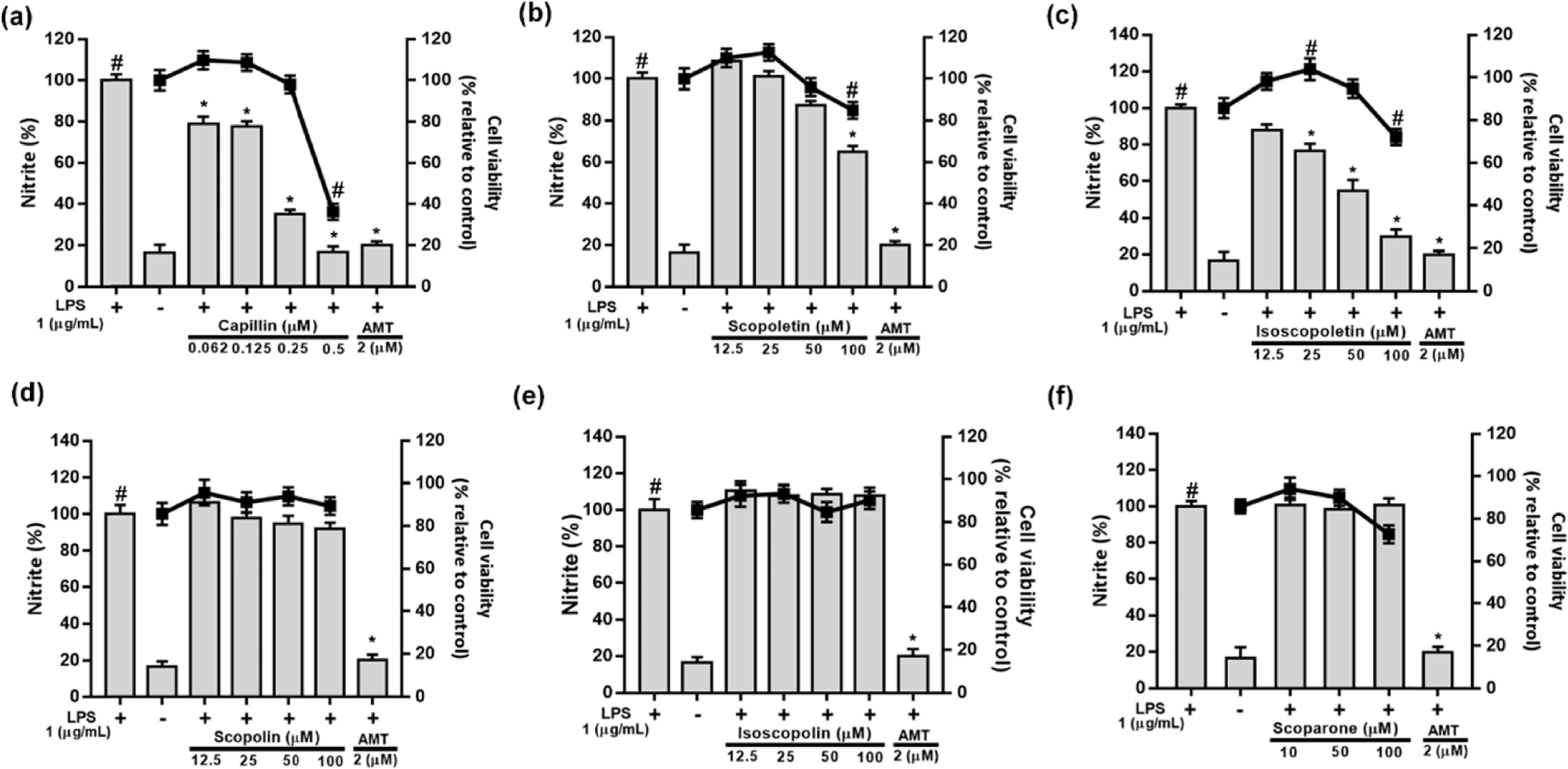