Abstract
The goal of nutrition of the preterm infant is to “provide nutrients to approximate the rate of growth and composition of weight gain for a normal fetus of the same postmenstrual age and to maintain normal concentrations of blood and tissue nutrients” (American Academy of Pediatrics 2014). Failure to provide the necessary amounts of all of the essential nutrients to preterm infants has produced not only growth failure, but also increased morbidity and less than optimal neurodevelopment. This continues to be true despite many efforts to increase nutrition of the preterm infants. In contrast, enhanced nutrition of very preterm infants, both intravenous and enteral, beginning right after birth, promotes positive energy and protein balance and improves longer term neurodevelopmental outcomes. The benefits are long lasting too, particularly for prevention of later life chronic diseases.
The goal of nutrition of the preterm infant is to provide nutrients to meet the growth rate and body composition of the normal healthy fetus of the same gestational age in terms of weight, length, and head circumference, organ size, tissue components including cell number and structure, concentrations of blood and tissue nutrients, and developmental outcomes [1]. Failure to provide the necessary amounts of all of the essential nutrients to preterm infants has produced not only growth failure, but also increased morbidity and less than optimal brain growth that would limit neurodevelopment [23]. This continues to be true despite many efforts to increase nutrition of the preterm infants. Most preterm infants fail to grow after birth, even beyond the early postnatal decline in weight due to normal extracellular water loss; they do not keep up with intrauterine growth rates; and thus they end up growth restricted relative to normally growing fetuses of the same gestational age by term gestation [4]. A principal reason for this postnatal growth failure is under nutrition, which starts at birth and continues for many days, as evidenced by cumulative protein and energy deficits [5]. In contrast, enhanced nutrition of very preterm infants, both intravenous (IV) and enteral, beginning right after birth promotes positive energy and protein balance and improves longer term neurodevelopmental outcomes. First week protein and energy intakes are associated with 18-moth developmental outcomes even in in extremely low birth weight (ELBW), extremely preterm infants. In one important study, every 1 g/kg/day increase in protein intake was associated with an 8.2 point increase in mental developmental index at 18 months and every 10 kcal/kg/day increase in energy intake was associated with a 4.6 point increase in mental development index at 18 months [6]. Specific benefits also may be observed, such as the lower risk of severe retinopathy of prematurity (ROP) when weight gain approaches that of the normally growing fetus [7]. The benefits can be long lasting too, particularly for prevention of later life chronic diseases. For example, first 3 week protein and energy intakes in very preterm infants have been positively associated with body composition benefits in 20-year old adults born as very LBW infants. In this study, every 1 g/kg/day increase in protein intake (starting at low intakes) was associated with a 22.5% higher lean body mass and a 22.1% higher resting energy expenditure, while energy and fat intakes were most positively associated with greater percent body fat, indicating that growth of lean body mass with more protein intake should be preferable to limit the potential for later life obesity [8].
We still are not achieving nutrient goals estimated to achieve normal metabolism, let alone growth, often for many days after birth, despite increased efforts to do so. As a result, during the first few days after birth, preterm infants are catabolic, using limited body stores and amino acids to maintain energy production at the expense of growth. Reasons for failure to provide sufficient nutrition to preterm infants are many, including a delayed start to providing nutrients, e.g., low or no IV amino acids on day 1 (to sometimes several days after birth) and enteral feedings often are held, sometimes for days; the amount of nutrient supplies are low, e.g., IV amino acid infusion rates of <2 g/kg/day; advancements of IV amino acids are slow even after starting early; advancements of enteral feeds are delayed; dilute nutritional mixes are used, e.g., unfortified breast milk (mother's own or banked); and there often are insufficient essential amino acids in currently available IV amino acid solutions. There also are numerous reasons that come up during ordinary neonatal care after birth for not starting, slowly advancing, or stopping nutrition, IV or enteral, usually for unsubstantiated concerns for metabolic toxicities, feeding intolerance, risk of necrotizing enterocolitis (NEC), or other common neonatal morbidities [9]. Regardless of the reason, the end result is under nutrition and less than optimal growth, in terms of weight, length, head circumference, and body composition. Under nutrition is a critical problem in preterm infants, as these infants are at critical stages of development when insufficient nutrient substrates and anabolic (growth promoting) hormones that are produced in response lead to growth failure and long-lasting neurodevelopmental outcomes [101112]. Therefore, we need to know the “nutrients” and their rates of administration for preterm infants that would match preterm neonatal metabolism and growth to fetal metabolism and growth at the same gestational age, and maintain normal concentrations of blood and tissue nutrients. We also need to review methods of providing nutrients that are safe and effective, but are necessarily different according to postnatal age and metabolic and feeding capacities.
Several practical aspects of medical management to better optimize the benefits of enhanced and improved nutrition in very preterm infants right after birth should be noted.
Preterm infants are characteristically physiologically unstable, especially right after birth. They have low and high oxygen concentrations, their glucose concentrations fluctuate significantly, blood pressure and organ perfusion often is highly variable and often low, and they produce significant amounts of counter-regulatory hormones, such as adrenaline (principally norepinephrine), cortisol, glucagon, and growth hormone that promote variable changes in cardiac output, organ perfusion, and glucose and oxygen values. Medical responses including infusions of catecholamines to increase blood pressure and hydrocortisone to promote blood pressure contribute significantly to the common physiological disturbances in such infants. These factors also produce highly variable gastrointestinal function, at least in terms of motility. Antibiotics contribute in major but still poorly understood ways to alter intestinal microbiota and the capacity for appropriate handling of subsequent enteral nutrient supplies [13]. IV nutrition alone complicates enteral feeding by leading to reduced gastrointestinal villous development, decreased secretion of digestive enzymes, reduced secretion of incretins (gut hormones, such as glucagon-like peptides 1 and 2) that promote insulin secretion, and reduced gut motility [141516]. These and other disturbances limit the success of appropriate parenteral and enteral nutrition. Good medical care can help reduce these problems and improve the capacity to start parenteral and enteral nutrition sooner and advance more effectively.
Growth and normal metabolism require sufficient blood oxygen content. Current medical practices lead far too often to reduced blood oxygen content and the potential for poor growth. We allow ELBW-VLBW (very low birth weight) infants to become more anemic (hematocrits in lower 20s) to limit transfusion risks (transfusion-related inflammatory disorders, possibly NEC, strokes, lung injury). We keep PaO2 values lower to prevent oxygen toxicity (e.g., ROP, bronchopulmonary dysplasia). We remove large amounts of blood for all sorts of biochemical and hematological measurements. In effect, we produce iatrogenic bloodletting anemia, compounding the natural postnatal anemia of prematurity. Research has shown that fetal growth is maintained when blood oxygen content increases in response to low PO2 values by increased erythropoietin production that increases red blood cell number [17]. After birth, earlier and more optimally dosed recombinant erythropoietin can be used.
Transfusions can be given early, before anemia becomes severe and damages enterocytes in the gastrointestinal tract that might promote risk for NEC and sepsis. In a specific study, preterm infants with hemoglobin values less than 8.5 g/dL and hematocrits less than 24% did not grow as well as preterm infants who had early transfusion of red blood cells that increased the hemoglobin to greater than 11.4 g/dL and hematocrits greater than 34% [18]. We also could promote safe approaches to delayed cord clamping and cord stripping, allowing the infant to breath or be ventilated first. Clearly we could do much better at reducing blood sampling and we could “microsize” blood sample assays. We also could develop and use more and better noninvasive monitoring.
IV feeding, including amino acids, should be started right after birth at rates that are appropriate for the gestational age of the infant. For several proven reasons, IV nutrition is fundamental in all infants who cannot tolerate full enteral feedings [19]. Metabolic and thus nutritional requirements do not stop with birth; this includes protein accretion. IV feeding is always indicated when normal metabolic needs are not met by normal enteral feeding. The smaller the infant, the less body stores (protein, fat, and glycogen) are available to provide nutrients for metabolic needs. The metabolic and thus nutrient requirements of the newborn are equal to or greater than those of the fetus of the same gestational age. It is reasonable, therefore, to provide the preterm infant with at least what the fetus of the same gestational age receives for nutrition to maintain normal metabolism.
A common approach to early IV dextrose is to infuse at 6 to 8 mg/kg/minute beginning at birth, increasing to 12 to 14 µg/minute/kg for full IV nutrition (~70–80 kcal/kg/day). But, the normal glucose utilization rate (GUR) is maximal at ~6–7 µg/kg/minute in preterm infants (largely for the brain and heart), and preterm infants quickly develop and maintain hepatic glucose production rates of 2 to 3 µg/kg/minute, which do not get shut down by dextrose infusion or normal to high glucose and insulin concentrations [2021]. Therefore, if one infuses 6 to 8 µg/kg/minute dextrose, total glucose entry is 8 to 11 µg/kg/minute, in excess of maximal GUR, leading to hyperglycemia, which gets even worse when the glucose infusion rate (GIR) is increased to 12 to 14 µg/kg/minute, especially if stress conditions develop and/or catecholamines, glucocorticoids, and high rates of IV lipid are infused for other medical conditions.
Low glucose values (hypoglycemia) are less common in preterm infants due to early and relatively high rates of IV dextrose infusions [22]. Studies have shown that such management is associated with normal school age neurodevelopmental and cognitive outcomes [23]. Extremely low glucose concentrations should be corrected immediately with increased IV dextrose infusion rates. Bolus glucose infusions are only indicated when severe signs of hypoglycemia, such as seizures or coma, are present.
Hyperglycemia is best prevented by starting with lower dextrose infusion rates, 3 to 4 µg/minute/kg, increasing or decreasing as needed to maintain glucose concentrations within the normal fetal level glucose concentration range, greater than 3 mMol/L and less than 6 mMol/L, in response to frequent plasma glucose concentration measurements to prevent hypo- and hyperglycemia. High glucose values are common and potentially deleterious, and are compounded by other risks for hyperglycemia, including reduced insulin secretion, limited enteral feedings with diminished secretion of “incretins” that promote insulin secretion, stress of all kinds but particularly sepsis that increases catecholamine and glucocorticoid secretion (as well as infusions of these hormones), early and high rates of IV lipid infusion that provide fatty acids to compete with glucose carbon for oxidation and also stimulate gluconeogenesis, and persistent hepatic glucose production that begins in utero in infants with growth restriction. There is no advantage to and many complications of higher dextrose infusion rates (Table 1).
Hyperglycemia also has been associated with increased incidence and severity of ROP [24]. It clearly leads to increased cellular glucose uptake, especially when promoted by insulin infusions, overwhelming its oxidative capacity in mitochondria and leading to pro-inflammatory reactive oxygen species production, which cause cellular damage and death and systemic inflammation [2526]. Bacteria thrive in high glucose environments, and hyperglycemia drives intestinal barrier dysfunction and increased permeability, systemic influx of microbial products, and enhanced dissemination of enteric infection, which might explain why hyperglycemia is associated with increased rates of bacterial sepsis and NEC [27]. Hyperglycemia also has a marked adverse impact on producing more unfavorable outcomes in infants with prior hypoxic-ischemic encephalopathy [28]. Fetal and neonatal animal studies increasingly are demonstrating that marked, prolonged hyperglycemia restricts dendritic spine development and thus synapse formation [29]. Previous studies have found no difference in many potential adverse outcomes by using a lower starting rate for IV dextrose infusion vs. insulin infusion, suggesting that the safest approach is to just lower the GIR rather than add insulin and its many risks [30], reserving insulin infusion for potentially dangerously high glucose concentrations, perhaps above 200 to 250 mg/dL. Insulin infusions will rapidly lower plasma glucose concentrations in preterm infants, but there are several risks of major concern (Table 2) [31]. While there are studies showing reduced mortality from hyperglycemia by using insulin infusions, it likely is the lowering of glucose concentrations and not just the use of insulin that produces this valuable outcome.
Practical approaches to preventing hyperglycemia are suggested in Table 3. Dextrose infusion rates should be started at lower infusion rates, 3 to 4 µg/kg/minute, unless there already is symptomatic and severe hypoglycemia. Earlier and more rapidly advanced enteral feeding should be started as soon as possible after birth [32]. Optimal dosing of IV amino acids according to gestational age should be started right after birth and is valuable in reducing glucose concentrations and producing fewer episodes of hyperglycemia, presumably by increasing insulin secretion and also by promoting energy, and thus glucose, requirements for protein synthesis [3334]. Dextrose infusion rates should be adjusted in response to relatively frequent measurements of plasma glucose concentrations, aiming to keep the glucose concentration in the normal fetal range, >3 mMol/L (>54 µg/dL) and <6 mMol/L (<108 mg/dL) as much of the time as possible. In the future, in infants with unstable glucose concentrations, it might be beneficial to consider using a continuous subcutaneous glucose monitor to do this [35].
Extremely preterm infants are slow to clear plasma of lipids (primarily triglycerides) due to maturational deficiency of lipases (inversely related to gestational age) and low levels of carnitine palmitoyltransferase that transports long chain fatty acids into mitochondria where they can be oxidized for energy production. But, even in preterm infants, right after birth, carnitine in mother's milk and preterm formulas promotes lipid oxidation, and milk also provides lipases. The best strategy to promote lipid oxidation and energy production, therefore, is to feed enterally as soon as possible.
IV lipid infusions are primarily important to prevent essential fatty acid deficiency, particularly of docosahexaenoic acid (DHA). Fetal white adipose tissue accumulation during last trimester of n-3 polyunsaturated fatty acids results in total body accretion rates of about 45 to 65 mg/day, most of which is the 22:6n-3 DHA. At 3.7 g fat/dL human milk with 0.2% to 0.4% fatty acids as 22:6n-3, a 1 kg preterm infant fed at full enteral feeds of 180 mL/day would get only 13 to 25 µg 22:6n-3/day, clearly below normal in utero accretion rates. As a result, postnatal DHA deficiency is an inevitable consequence of current practices for feeding milk, milk supplements, and formulas in preterm infants [36]. Preterm infants fed increased 22:6n-3 (DHA) have higher visual acuity, particularly at 2 and 4 months and improved Bayley mental development and MacArthur Communicative inventories at 12 months [3738]. Longer term studies, however, do not show a clear benefit [39]. Thus, the current diet for preterm infants is deficient in DHA, but the long term significance of this deficiency is not known or how these infants would develop if fed to sufficiency. The best strategy is to provide early enteral feeding, as milk and formulas have more DHA than IV lipids.
Current available IV lipid products are quite variable in their lipid composition. Soybean oil products are highly associated with parenteral nutrition associated liver disease (PNALD), perhaps due to low concentrations of anti-inflammatory alpha-tocopherol, absent DHA, and high concentrations of highly inflammatory phytosterols [40]. Exclusive fish oil products that contain DHA and alpha-tocopherol but no phytosterols produce little or no PNALD (and can rescue infants from progressive PNALD even when advanced in severity and chronicity) [41]. Other products have mixtures of these oils and intermediate degrees of PNALD. Together, customary IV dextrose and lipid provide more than sufficient non-protein calories for normal metabolism and adipose lipid storage, but only increased amino acid intakes can promote protein accretion during exclusive IV nutrition feeding periods.
Appropriate strategies for lipid infusions include starting IV lipid on day 1 at 2–3 g/kg/day, advancing over 2–3 days to 3.0–3.5 g/kg/day. It makes sense to use an IV lipid emulsion that contains fish oil for DHA and α-tocopherol (vitamine E) and to avoid the high doses of phytosterols found in soybean oil products. Overall, it is best to start milk feedings on day 1 to provide lipases and especially carnitine.
The largest requirement for growth, of the entire body, not just lean body mass, is protein, accounting for 80% to 90% of non-water growth over the latter third of gestation (fat accumulation accounts for 10% to 20% of body fat mass by term gestation). Adequate and gestational age-appropriate IV amino acid intake is fundamental for promoting growth of all cells in all tissues and organs, including in particularly the brain. Fractional protein synthetic and growth rates are much higher in the early gestational age fetus and thus in the extremely preterm infant, requiring up to 4 g/kg/day for normal protein balance and growth at 24 to 28 weeks gestation [42] (Fig. 1). At later gestational ages, fractional growth and protein synthetic rates decline, requiring less IV amino acids for protein balance, to rates of 2.5 to 3.5 g/kg/day between 32 and 37 weeks. At term, amino acid requirements decrease to those of the normal breast fed infant, or about 1.5 to 2.0 g/kg/day [43]. Importantly, even in the first 48 hours and in relatively unstable very preterm infants there is a direct correlation between amino acid supply and net protein balance [44].
Furthermore, maintaining a gestational age appropriate amino acid supply is important for growth of individual organs and tissues, particularly the brain, and this is important to do as IV nutrition is gradually reduced and enteral feedings advanced, as far too often, IV nutrition is minimized before full enteral feedings are achieved, leading to often extensive periods in which total nutrition, particularly of protein, is insufficient [4546].
Concerns about excess amino acids and potential toxicity are commonly noted but are not substantiated. However, there is great need for more and better research to resolve such concerns and to determine optimal amino acid solutions and rates of administration. As recently summarized in a Chochrane Database Systematic Review [47], low-quality evidence suggests that higher amino acid intake in parenteral nutrition does not affect mortality. Very low-quality evidence suggests that higher amino acid intake reduces the incidence of postnatal growth failure. Evidence currently is insufficient to show an effect of amino acid dosing on neurodevelopment. Very low-quality evidence suggests that higher amino acid intake reduces ROP but not severe ROP. Higher amino acid intake is associated with potentially adverse effects from excess amino acid load, including azotaemia, although there is no consistent relationship among birth weight, gestational age, amino acid intake, and plasma blood urea nitrogen (BUN) or ammonia concentrations [48]. Furthermore, slightly increased ammonia and BUN concentrations reflect a normal response of increased amino acid oxidation as protein accretion (net protein balance) is achieved and a normally functioning liver and urea cycle activity that is detoxifying ammonia. Most cases of increased BUN reflect inadequate urine flow rates. Clearly, adequately powered trials in very preterm infants are required to determine the optimal intake of amino acids and effects of caloric balance in parenteral nutrition on the brain and on neurodevelopment.
Increasing protein synthesis and net protein balance (protein accretion) require increasing amounts of energy, but this is only true at lower energy intakes when protein accretion is augmented by more energy as well as protein. At higher energy intakes, regardless of the protein intake, this is not the case, as with sufficient energy, above ~70 (IV) or ~90 (enteral) non-protein kcal/kg/day, there is no further increase in protein gain for a further increase in energy intake. Protein gain in this situation primarily depends on protein intake, as it also does at any energy intake. Protein accretion is augmented by more protein, not more energy once sufficient energy is supplied, and excess energy only leads to more fat production in adipose tissue and in organs, such as the heart and liver, where it produces harmful inflammation [4950].
Under nutrition, principally of protein, at critical stages of development permanently limits brain growth and subsequent neurological function. Under nutrition reduces structural growth of the brain in several ways, including reduced neuronal number, axonal length, dendritic spine formation and dendritic number, dendritic arborization, and synapse formation. In later life, these structural deficits lead to limitations in functional development, including reduced cognitive functions such as learning and memory, and interactive behavior [5152].
Balanced amounts of energy and protein supplies also are essential to maintain to promote growth of the brain and its contents, with improved magnetic resonance imaging (MRI) gray matter scores directly correlated with energy (fat and carbohydrate) and protein intakes. Brain volumes studied by MRI vs. gestational age show that preterm infants lag behind in growth of all regions of the brain, as well as total brain volume [53]. Of note, however, such improvements in neurological growth and development have limits with respect to energy and protein intakes. Higher than needed amounts of energy and protein do not correlate with further increases in brain growth and neurodevelopment, and might indicate that even higher amounts might become harmful [54].
In very preterm infants, however, even gestational age appropriate total amino acid intakes may not produce overall growth as well as length and head circumference [55]. This starts early after birth, as current IV amino acid solutions contain lower amounts than needed of certain essential amino acids, particularly leucine [56]. Another advantage of increased IV amino acid infusion rates, as already noted, is their stimulation of protein synthesis and insulin secretion, both of which lead to lower glucose concentrations and reduced incidence and severity of hyperglycemia [3334]. There also is clear evidence of improved neurodevelopmental outcomes with improved and increased and balanced energy and protein intakes [5758].
There are several strategies for ensuring adequate protein intake in preterm infants. The first is to start on day 1 of postnatal life, preferably upon admission to the neonatal intensive care unit (NICU), with gestational age appropriate IV amino acid infusion rates: 24 to 30 weeks, 3.5 to 4.0 g/kg/day; 30 to 34 weeks, 3.0 to 3.5 g/kg/day; 34 to 36 weeks, 2.5 to 3.0 g/kg/day. These slightly higher rates are good targets, as there usually are many interruptions to early IV nutrition. Once enteral nutrition is advancing, to maintain appropriate water intake and water balance, IV amino acid solutions can be concentrated to maintain amino acid intakes at gestational age appropriate rates. Enteral feeding should be started as soon as possible and advanced as tolerated.
In preterm infants, there still is no evidence that insulin infusion to “enhance” nutrition is beneficial, and there are many potential problems (e.g., increased number of episodes of hypoglycemia; increased cortisol secretion, and higher blood pressure). For the most part, insulin treatment to increase growth will simply make the baby fatter (and produce pathologically fatty livers and hearts and promote systemic inflammation). There also is no evidence that insulin increases brain glucose uptake or brain growth. Furthermore, negative feedback mechanisms limit a sustained effect of continued high insulin concentrations on amino acid synthesis into proteins and protein accretion. Prophylactic insulin administration in fact has led to shorter legs in treated infants [59].
IV feeding may be essential, but it should not be a long term substitute for enteral feeding and nutrition. Enteral feeding should be started as soon as possible after birth, using mother's colostrum and milk as first choices. Enteral feedings promote gastrointestinal development, even when given in small, “trophic” or gut priming”, amounts. Gastrointestinal mucosal growth and development depend on enteral, not parenteral, nutrients. Enteral feeding promotes the capacity for improved feeding tolerance and growth, less need for phototherapy (by promoting stooling), decreases cholestasis, decreases osteopenia (more calcium and phosphorus in enteral than IV feedings), promotes gastrointestinal trophic hormone surges, improves gastrointestinal motility [60].
Maternal milk feedings reduce the incidence of late onset sepsis and NEC and improve neurodevelopmental outcomes in preterm infants. Even donor human milk, despite its reduced immune functions and protein concentrations, reduces the incidence of NEC relative to intact cow milk protein formulas. Enteral feeding with milk enhances gut development and growth, hence the correct terms of “trophic” or “gut priming” feeding. Mucosal growth and development depend on enteral (not parenteral) nutrients, shown by increased gut weight, gut protein content, and gut DNA content. Enteral feedings also lead to improved motility and feeding tolerance, less need for phototherapy, decreased development of cholestasis and PNALD, decreased osteopenia, and increased gastrointestinal trophic hormone surges [61]. Most studies indicate increased gut blood flow with enteral feeding [62].
Enteral feeding should begin with trophic amounts of 20 to 30 mL/kg on day 1 and advanced as rapidly as tolerated, decreasing IV nutrition accordingly, while maintaining nutrient intakes at recommended rates [63]. Most studies now show that enteral feeding, particularly of maternal colostrum and milk, can start as early as the 1st day of life in relatively stable infants at rates as high as 20 to 30 mL/kg/day and can be advanced up to 30 to 40 mL/kg/day, advancing to full enteral feeding by 7 to 10 days, even in extremely preterm infants [6465]. Currently available data do not provide evidence that slower rates of advancement (less than 24 mL/kg/day) of enteral feed volumes reduces the risk of NEC in VLBW infants. Increasing the volume of enteral feeds at slow rather than faster rates results in several days delay in regaining birth weight and establishing full enteral feeds. This also is true of relatively stable infants with intrauterine growth restriction. In essentially all studies, feeding protocols have proven valuable for advancing enteral feedings more quickly and safely as well as improving nutrition and related outcomes.
Particularly for donor human milk, but also for mature maternal milk, protein content is insufficient at normal enteral feeding rates (160–180 mL/kg/day) to support gestational age appropriate protein accretion and growth rates [66]. Most commercial fortifiers are recommended at doses that are inadequate to compensate for very low protein concentrations in donor and mature maternal milk [67]. In most recent studies, higher fortifier or additional protein supplements are necessary to promote more positive protein balance and growth rates, particularly in extremely preterm, ELBW infants [68]. Fortification in such infants is necessary, therefore, not just an increase in feeding volume, to achieve positive protein balance and growth of lean body mass, the brain, and bones [69]. In more mature preterm infants, those in the late preterm to early term gestational age range, often can be fed larger amounts of milk without fortifier, in the rage of 180 to 240 mL/kg/day, which can provide sufficient protein and energy for growth, though not necessarily of calcium, phosphorus, or other micronutrients. This becomes less of a problem as the infant reaches term to early post-term age when growth rates naturally decline.
Protocols to guide nutrition have been found particularly useful in busy NICUs when otherwise highly variable nutritional practices produce highly variable growth outcomes. Some programs also are tailoring nutritional amounts of different products (mother's milk after birth, mature mother's or donor milk) and fortification according to measured amounts of nutrients in the milk. Milk analyzers are not generally available and are very expensive. A simpler approach in very preterm infants is just to add at least 1 g/kg/day of protein to the milk, fortified or not, which usually improves protein balance [70].
Enteral feeding strategies to prevent postnatal growth restriction are clear. Feed enterally as soon as possible. Use maternal colostrum right after birth, advancing to maternal milk as soon as available, even on day 1. Advance enteral nutrition as tolerated, aiming for 30 to 40 mL/kg/day (although many go slower in ELBW infants based on historical concerns for feeding intolerance). Use mother's milk as much as possible; fortify when enteral intake is advancing well. Use a standard feeding protocol, but consider a milk analyzer to help with individualized nutrition.
Growth outcomes of ELBW infants remain suboptimal, because they are not fed enough, especially of protein. It is worth reflecting, therefore, on what is normal fetal nutrition if we are trying to mimic this in preterm infants. In the normally growing fetus, amino acids are actively transported by the placenta into the fetus at rates and concentrations that are higher than the fetus can use. The excess amino acid load is oxidized for energy.
Glucose is taken up according to its rate of utilization and used to meet energy needs. In contrast, with current, “customary” ELBW/VLBW nutrition, glucose is pumped into the infant at rates and concentrations that are higher than the infant can use. The excess glucose load produces potentially deleterious hyperglycemia. This is compounded by high rates of lipid infusion. In general, preterm infants have been fed excessive energy, which only makes them fatter; but they still lack essential fatty acids (DHA). Amino acids, however are usually provided at rates that often are less than needed for normal rates of growth, particularly of the brain.
Current IV nutritional practices that support growth and development in preterm infants directly follow what we have learned about the nutrition of the fetus that supports its normal growth and development. Gestational age specific protein intake promotes positive protein balance. Appropriate supplies/concentrations of essential amino acids are necessary, but still may be insufficient in current IV nutrition solutions. Appropriate energy is necessary to support metabolism and growth, but excessive energy intake leads to fat deposition, not greater protein balance. IV GIRs should be adjusted frequently to maintain normal glucose concentrations (3–6 mMol/L), and essential lipids (e.g., DHA) and valuable, not just more calories in the form of lipids. Growth factors (e.g., insulin) should occur in response to appropriate nutrient supplies, not infused.
Optimal IV nutrition with increased amino acid to energy ratios reduce the time to regain birth weight, supporting the positive effects on growth and development.
There are several strategies to improve early ELBW/VLBW nutrition. Early protein losses are minimized by infusing amino acids into the infant at rates and concentrations appropriate for gestational age: 4 g/kg/day for extremely preterm infants, 3 g/kg/day for moderately preterm infants, and 2 g/kg/day for infants close to term; less amino acids and protein could lead to neurological deficits. Initial amino acid supplies should be supplemented with ~70 (IV) to 90 (enteral) non-protein kcal/kg/day and 3 to 4 g/kg/day. The excess amino acid load in all cases will be oxidized, producing useful energy (or lost in the urine). These amino acid and protein intakes may approximate fetal protein accretion and growth in reasonably healthy ELBW infants and improve neurodevelopmental and growth outcomes, both short and long term. Glucose should be provided first to meet acute glucose needs: usually, 4 to 5 µg/kg/minute will be sufficient to maintain normal glucose concentrations, but should be adjusted frequently in response to plasma glucose concentration measurements. At most, 7 to 10 µg/min/kg could be infused (about 28 to 40 kcal/kg/day), but this usually requires maximizing amino acid infusion rates first to maximize protein synthesis and to stimulate insulin secretion. Lipid should be provided to meet essential fatty acid requirements first, and for additional energy needs only secondarily: 2 to 3 g/kg/day=18 to 36 kcal/kg/day.
In all cases, trophic or gut priming gastric colostrum and milk “feedings” should start on day 1 in all “healthy” preterm infants at 20 to 30 mL/kg/day and advance by 30 to 40 mL/kg/day as long as tolerated. Further research is needed to determine the optimal nutrition and rate of growth in preterm infants that will achieve optimal neurocognitive benefits while minimizing the longer-term risk of chronic diseases.
Figures and Tables
Fig. 1
Relationship between fractional protein synthetic rates (Ks) and fractional growth rates (Kg) and gestational age in fetal lambs. Adapted from Hay WW Jr et al. (In: Polin RA, et al, eds. Fetal and Neonatal Physiology, 4th ed.) [42].
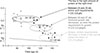
ACKNOWLEDGEMENTS
The author is funded by NIH grants K12 HD068372, UL1TR002535, and T32 HD007186.
The author should like to dedicate this review to Richard A. Ehrenkranz, MD, who has made so many substantial contributions to the nutrition and health of preterm infants.
References
1. Kleinman RE, Greer FR. American Academy of Pediatrics. Pediatric Nutrition. 7th ed. Elk Grove Village, IL: American Academy of Pediatrics;2014.
2. Bouyssi-Kobar M, du Plessis AJ, McCarter R, Brossard-Racine M, Murnick J, Tinkleman L, et al. Third trimester brain growth in preterm infants compared with in utero healthy fetuses. Pediatrics. 2016; 138:e20161640.


3. Ehrenkranz RA, Dusick AM, Vohr BR, Wright LL, Wrage LA, Poole WK. Growth in the neonatal intensive care unit influences neurodevelopmental and growth outcomes of extremely low birth weight infants. Pediatrics. 2006; 117:1253–1261.


4. Horbar JD, Ehrenkranz RA, Badger GJ, Edwards EM, Morrow KA, Soll RF, et al. Weight growth velocity and postnatal growth failure in infants 501 to 1500 grams:2000-2013. Pediatrics. 2015; 136:e84–e92.
5. Dinerstein A, Nieto RM, Solana CL, Perez GP, Otheguy LE, Larguia AM. Early and aggressive nutritional strategy (parenteral and enteral) decreases postnatal growth failure in very low birth weight infants. J Perinatol. 2006; 26:436–442.


6. Stephens BE, Walden RV, Gargus RA, Tucker R, McKinley L, Mance M, et al. First-week protein and energy intakes are associated with 18-month developmental outcomes in extremely low birth weight infants. Pediatrics. 2009; 123:1337–1343.


7. Binenbaum G, Bell EF, Donohue P, Quinn G, Shaffer J, Tomlinson LA, et al. G-ROP Study Group. Development of modified screening criteria for retinopathy of prematurity: primary results from the postnatal growth and retinopathy of prematurity study. JAMA Ophthalmol. 2018; 136:1034–1040.


8. Matinolli HM, Hovi P, Männistö S, Sipola-Leppänen M, Eriksson JG, Mäkitie O, et al. Early protein intake is associated with body composition and resting energy expenditure in young adults born with very low birth weight. J Nutr. 2015; 145:2084–2091.


9. Hay WW. Optimizing nutrition of the preterm infant. Zhongguo Dang Dai Er Ke Za Zhi. 2017; 19:1–21.
10. Belfort MB, Rifas-Shiman SL, Sullivan T, Collins CT, McPhee AJ, Ryan P, et al. Infant growth before and after term: effects on neurodevelopment in preterm infants. Pediatrics. 2011; 128:e899–e906.


11. Belfort MB, Gillman MW, Buka SL, Casey PH, McCormick MC. Preterm infant linear growth and adiposity gain: trade-offs for later weight status and intelligence quotient. J Pediatr. 2013; 163:1564–1569.


12. Latal-Hajnal B, von Siebenthal K, Kovari H, Bucher HU, Largo RH. Postnatal growth in VLBW infants: significant association with neurodevelopmental outcome. J Pediatr. 2003; 143:163–170.


13. Chong CYL, Bloomfield FH, O'Sullivan JM. Factors affecting gastrointestinal microbiome development in neonates. Nutrients. 2018; 10:274.


14. Commare CE, Tappenden KA. Development of the infant intestine: implications for nutrition support. Nutr Clin Pract. 2007; 22:159–173.


15. Berni Canani R, Passariello A, Buccigrossi V, Terrin G, Guarino A. The nutritional modulation of the evolving intestine. J Clin Gastroenterol. 2008; 42:Suppl 3 Pt 2. S197–S200.


16. Amin H, Holst JJ, Hartmann B, Wallace L, Wright J, Sigalet DL. Functional ontogeny of the proglucagon- derived peptide axis in the premature human neonate. Pediatrics. 2008; 121:e180–e186.
17. Kitanaka T, Alonso JG, Gilbert RD, Siu BL, Clemons GK, Longo LD. Fetal responses to long-term hypoxemia in sheep. Am J Physiol. 1989; 256:R1348–R1354.


18. Stockman JA 3rd, Clark DA. Weight gain: a response to transfusion in selected preterm infants. Am J Dis Child. 1984; 138:828–830.


19. Harding JE, Cormack BE, Alexander T, Alsweiler JM, Bloomfield FH. Advances in nutrition of the newborn infant. Lancet. 2017; 389:1660–1668.


20. Sunehag A, Ewald U, Larsson A, Gustafsson J. Glucose production rate in extremely immature neonates (< 28 weeks) studied by use of deuterated glucose. Pediatr Res. 1993; 33:97–100.


21. Chacko S, Sunehag A. Gluconeogenesis continues in premature infants receiving total parenteral nutrition. Arch Dis Child Fetal Neonatal Ed. 2010; 95:F413–F418.


22. Rozance PJ, Hay WW Jr. IModern management of preterm infants prevents adverse developmental outcomes from hypoglycemia. Pediatrics. 2016; 138:e20162881.


23. Goode RH, Rettiganti M, Li J, Lyle RE, Whiteside-Mansell L, Barrett KW, et al. Developmental outcomes of preterm infants with neonatal hypoglycemia. Pediatrics. 2016; 138:e20161424.


24. Mohsen L, Abou-Alam M, El-Dib M, Labib M, Elsada M, Aly H. A prospective study on hyperglycemia and retinopathy of prematurity. J Perinatol. 2014; 34:453–457.


25. Picard M, Juster RP, McEwen BS. Mitochondrial allostatic load puts the ‘gluc’ back in glucocorticoids. Nat Rev Endocrinol. 2014; 10:303–310.


26. Stoll B, Horst DA, Cui L, Chang X, Ellis KJ, Hadsell DL, et al. Chronic parenteral nutrition induces hepatic inflammation, steatosis, and insulin resistance in neonatal pigs. J Nutr. 2010; 140:2193–2200.


27. Wang A, Huen SC, Luan HH, Yu S, Zhang C, Gallezot JD, et al. Opposing effects of fasting metabolism on tissue tolerance in bacterial and viral inflammation. Cell. 2016; 166:1512–1525.


28. Basu SK, Kaiser JR, Guffey D, Minard CG, Guillet R, Gunn AJ. CoolCap Study Group. Hypoglycaemia and hyperglycaemia are associated with unfavourable outcome in infants with hypoxic ischaemic encephalopathy: a post hoc analysis of the CoolCap Study. Arch Dis Child Fetal Neonatal Ed. 2016; 101:F419–F455.


29. Jing YH, Song YF, Yao YM, Yin J, Wang DG, Gao LP. Retardation of fetal dendritic development induced by gestational hyperglycemia is associated with brain insulin/ IGF-I signals. Int J Dev Neurosci. 2014; 37:15–20.


30. Meetze W, Bowsher R, Compton J, Moorehead H. Hyperglycemia in extremely- low-birth-weight infants. Biol Neonate. 1998; 74:214–221.


31. Beardsall K, Vanhaesebrouck S, Ogilvy-Stuart AL, Ahluwalia JS, Vanhole C, Palmer C, et al. A randomised controlled trial of early insulin therapy in very low birth weight infants, “NIRTURE” (neonatal insulin replacement therapy in Europe). BMC Pediatr. 2007; 7:29.


32. Zamir I, Tornevi A, Abrahamsson T, Ahlsson F, Engström E, Hallberg B, et al. Hyperglycemia in extremely preterm infants-insulin treatment, mortality and nutrient intakes. J Pediatr. 2018; 200:104–110.


33. Burattini I, Bellagamba MP, Spagnoli C, D'Ascenzo R, Mazzoni N, Peretti A, et al. Targeting 2.5 versus 4 g/kg/day of amino acids for extremely low birth weight infants: a randomized clinical trial. J Pediatr. 2013; 163:1278–1282.


34. Bellagamba M, Carmenati E, D'Ascenzo R, Malatesta M, Spagnoli C, Biagetti C, et al. One extra gram of protein to preterm infants from birth to 1800 g: a single-blinded randomized clinical trial. J Pediatr Gastroenterol Nutr. 2016; 62:879–884.
35. Ogilvy-Stuart AL, Beardsall K. Management of hyperglycaemia in the preterm infant. Arch Dis Child Fetal Neonatal Ed. 2010; 95:F126–F131.


36. Lapillonne A, Eleni dit Trolli S, Kermorvant-Duchemin E. Postnatal docosahexaenoic acid deficiency is an inevitable consequence of current recommendations and practices in preterm infants. Neonatology. 2010; 98:397–403.


37. Tam EWY, Chau V, Barkovich AJ, Ferriero DM, Miller SP, Rogers EE, et al. Early postnatal docosahexaenoic acid levels and improved preterm brain development. Pediatr Res. 2016; 79:723–730.


38. Carlson SE, Werkman SH, Rhodes PG, Tolley EA. Visual acuity development in healthy preterm infants: effect of marine oil supplementation. Am J Clin Nutr. 1993; 58:35–42.


39. Gould JF, Colombo J, Collins CT, Makrides M, Hewawasam E, Smithers LG. Assessing whether early attention of very preterm infants can be improved by an omega-3 long-chain polyunsaturated fatty acid intervention: a follow-up of a randomised controlled trial. BMJ Open. 2018; 8:e020043.


40. El Kasmi KC, Anderson AL, Devereaux MW, Vue PM, Zhang W, Setchell KD, et al. Phytosterols promote liver injury and Kupffer cell activation in parenteral nutrition-associated liver disease. Sci Transl Med. 2013; 5:206ra137.


41. Anez-Bustillos L, Dao DT, Fell GL, Baker MA, Gura KM, Bistrian BR, et al. Redefining essential fatty acids in the era of novel intravenous lipid emulsions. Clin Nutr. 2018; 37:784–789.


42. Hay WW Jr, Brown , LD , Regnault TRH. Fetal requirements and placental transfer of nitrogenous compounds. In : Polin R, Abman S, Benetz W, Rowitch D, editors. Fetal and neonatal physiology. 5th ed. Philadelphia: Elsevier;2016. p. 444–458.
43. Ziegler EE, O'Donnell AM, Nelson SE, Fomon SJ. Body composition of the reference fetus. Growth. 1976; 40:329–341.
44. Thureen PJ, Anderson AH, Baron KA, Melara DL, Hay WW Jr, Fennessey PV. Protein balance in the first week of life in ventilated neonates receiving parenteral nutrition. Am J Clin Nutr. 1998; 68:1128–1135.


45. Hay WW, Ziegler EE. Growth failure among preterm infants is not innocuous and must be prevented. J Perinatol. 2016; 36:500–502.


46. Miller M, Vaidya R, Rastogi D, Bhutada A, Rastogi S. From parenteral to enteral nutrition: a nutrition-based approach for evaluating postnatal growth failure in preterm infants. JPEN J Parenter Enteral Nutr. 2014; 38:489–497.
47. Osborn DA, Schindler T, Jones LJ, Sinn JK, Bolisetty S. Higher versus lower amino acid intake in parenteral nutrition for newborn infants. Cochrane Database Syst Rev. 2018; 3:CD005949.


48. Ridout E, Melara D, Rottinghaus S, Thureen PJ. Blood urea nitrogen concentration as a marker of amino- acid intolerance in neonates with birthweight less than 1250 g. J Perinatol. 2005; 25:130–133.


49. Micheli JL, Schutz Y. Protein. In : Tsang RC, Lucas A, Uauy R, Zlotkin S, editors. Nutritional needs of the preterm infant; Scientific basis and practical guidelines. Pawling, NY: Caduceus Medical Publishers;1993. p. 29–46.
50. Kashyap S, Forsyth M, Zucker C, Ramakrishnan R, Dell RB, Heird WC. Effects of varying protein and energy intakes on growth and metabolic response in low birth weight infants. J Pediatr. 1986; 108:955–963.


51. Smart JL. Vulnerability of developing brain to undernutrition. Ups J Med Sci Suppl. 1990; 48:21–41.
52. Smart JL. Critical periods in brain development. In : Bock GR, Whelan J, editors. The childhood environment and adult disease: Ciba Foundation Symposium 156. Chichester: Wiley;1991. p. 109–128.
53. Beauport L, Schneider J, Faouzi M, Hagmann P, Hüppi PS, Tolsa JF, et al. Impact of early nutritional intake on preterm brain: a magnetic resonance imaging study. J Pediatr. 2017; 181:29–36.e1.


54. Reid J, Makrides M, McPhee AJ, Stark MJ, Miller J, Collins CT. The effect of increasing the protein content of human milk fortifier to 1.8 g/100 mL on growth in preterm infants: a randomised controlled trial. Nutrients. 2018; 10:634.


55. Morgan C, McGowan P, Herwitker S, Hart AE, Turner MA. Postnatal head growth in preterm infants: a randomized controlled parenteral nutrition study. Pediatrics. 2014; 133:e120–e128.


56. Clark RH, Chace DH, Spitzer AR. Effects of two different doses of amino acid supplementation on growth and blood amino acid levels in premature neonates admitted to the neonatal intensive care unit: a randomized, controlled trial. Pediatrics. 2007; 120:1286–1296.


57. Poindexter BB, Langer JC, Dusick AM, Ehrenkranz RA. National Institute of Child Health and Human Development Neonatal Research Network. Early provision of parenteral amino acids in extremely low birth weight infants: relation to growth and neurodevelopmental outcome. J Pediatr. 2006; 148:300–305.


58. Lucas A, Morley R, Cole TJ, Gore SM, Lucas PJ, Crowle P, et al. Early diet in preterm babies and developmental status at 18 months. Lancet. 1990; 335:1477–1481.


59. Tottman AC, Alsweiler JM, Bloomfield FH, Gamble G, Jiang Y, Leung M, et al. Long-term outcomes of hyperglycemic preterm infants randomized to tight glycemic control. J Pediatr. 2018; 193:68–75.e1.


60. SIFT Investigators Group. Early enteral feeding strategies for very preterm infants: current evidence from Cochrane reviews. Arch Dis Child Fetal Neonatal Ed. 2013; 98:F470–F472.
61. Owens L, Burrin DG, Berseth CL. Minimal enteral feeding induces maturation of intestinal motor function but not mucosal growth in neonatal dogs. J Nutr. 2002; 132:2717–2722.


62. Niinikoski H, Stoll B, Guan X, Kansagra K, Lambert BD, Stephens J, et al. Onset of small intestinal atrophy is associated with reduced intestinal blood flow in TPN-fed neonatal piglets. J Nutr. 2004; 134:1467–1474.


63. Arnon S, Sulam D, Konikoff F, Regev RH, Litmanovitz I, Naftali T. Very early feeding in stable small for gestational age preterm infants: a randomized clinical trial. J Pediatr (Rio J). 2013; 89:388–393.


64. Abiramalatha T, Thomas N, Gupta V, Viswanathan A, McGuire W. High versus standard volume enteral feeds to promote growth in preterm or low birth weight infants. Cochrane Database Syst Rev. 2017; 9:CD012413.


65. Oddie SJ, Young L, McGuire W. Slow advancement of enteral feed volumes to prevent necrotising enterocolitis in very low birth weight infants. Cochrane Database Syst Rev. 2017; 8:CD001241.


66. Radmacher PG, Adamkin DH. Fortification of human milk for preterm infants. Semin Fetal Neonatal Med. 2017; 22:30–35.


67. Kuschel CA, Harding JE. Multicomponent fortified human milk for promoting growth in preterm infants. Cochrane Database Syst Rev. 2004; (1):CD000343.


68. Arnold M, Adamkin D, Radmacher P. Improving fortification with weekly analysis of human milk for VLBW infants. J Perinatol. 2017; 37:194–196.

