Abstract
Purpose
To improve the treatment efficiency of optic nerve diseases by delivering therapeutic materials to the optic nerve directly.
Methods
We tried to optimize liposomal composition to deliver a payload to the optic nerve efficiently when it is injected intravitreally. After loading dexamethasone into this liposome, we tested the therapeutic effect of liposomes in this treatment using a murine model of ischemic optic neuropathy.
Results
Our optimized liposome can deliver its payload to the optic nerve more efficiently than other tested compositions. Moreover, dexamethasone-loaded liposomes had a significant therapeutic effect in a murine model of ischemic optic neuropathy.
Conclusions
Here, we demonstrate the optimal composition of liposomes that could efficiently deliver intravitreally injected exogenous compounds to the optic nerve. We expect that the intravitreal injection of liposomes with the suggested composition would improve the delivery efficacy of therapeutic compounds to the optic nerve.
The optic nerve is the main lesion site in various ocular diseases, such as optic neuropathy, optic neuritis, and glaucoma, which is the second leading cause of blindness worldwide [12]. Systemic steroid therapy is currently the method used for treating optic neuropathy and optic neuritis [3]. However, systemic steroid therapy has low delivery efficacy to the optic nerve, and it can cause mild to severe systemic side effects, such as insomnia, dyspepsia, hyperglycemia, and hypertension [4]. Glaucoma is caused by damage to the ganglion cell axon, but no treatment can restore the damaged ganglion cell directly or provide neuroprotection [5]. Instead, eye drops are used to lower the intraocular pressure and prevent further axonal damage.
Intravitreal injection is widely used to deliver therapeutic compounds directly to the eyes. For example, intravitreal injection of steroids is effective in managing diabetic macular edema [6]. In addition, intravitreal injection of anti-vascular endothelial growth factor prevents disease progression in patients with exudative age-related macular degeneration [7]. However, the short retention time of the injected drugs in the vitreous humor necessitates the injection of highly concentrated drugs or frequent intravitreal injections. This has a toxic effect on the retina or other eye structures, resulting in cataract or glaucoma. Frequent injections can also cause severe complications, such as endophthalmitis and retinal detachment [8]. Similarly, in cases of optic nerve disease, studies have shown that intravitreal injection of steroids to treat ischemic optic neuropathy (ION) has a therapeutic effect. However, the delivery efficacy of steroids to the optic nerve is very low, and they could cause severe complications, such as high intraocular pressure and cataract [91011].
To overcome these limitations of intravitreal injection, efforts have been made to inject drug-loaded nanoparticles intravitreally. The use of nanoparticles increases the retention time of the injected drug, thereby decreasing drug toxicity and injection frequency by preventing early degradation and promoting the slow release of drugs [1213]. Among the various kinds of nanoparticles, liposomes are biocompatible and can load hydrophilic and hydrophobic compounds separately or simultaneously. In addition, their physical properties are easily tunable [14]. Unfortunately, to the best of our knowledge, no study has reported the use of liposomes to deliver therapeutic compounds to the optic nerve.
Recently, we reported a liposome with a novel composition that could disperse in the vitreous humor and deliver its payload to the deep retinal layer when injected intravitreally [15]. Here, we demonstrate a technique for the intravitreal injection of this optimized liposome that could effectively deliver a hydrophobic model drug embedded in a lipid bilayer to the optic nerve. Additionally, we applied this technique to treat ION in a murine model by loading dexamethasone in the liposomes and revealed its significant therapeutic effect.
1,2-Dimyristoyl-sn-glycero-3-phosphocholine (DMPC), 1,2-distearoyl-sn-glycero-3-phosphoethanolamine-N-[methoxy(polyethylene glycol)-2000] (DSPE-PEG2000), and 1,2-dioleoyl-3-trimethylammonium-propane (DOTAP) were obtained from Avanti Polar Lipids (Alabaster, AL, USA). 1,1′-dioctadecyl-3,3,3′,3′-tetramethylindocarbocyanine perchlorate (DiI) was purchased from Thermo Scientific (New York, NY, USA).
All experiments were conducted in accordance with the ARVO Statement for the Use of Animals in Ophthalmic and Vision Research. Twelve-week-old C57BL/6 female mice were purchased from Koatech (Daejeon, Korea). The animals were maintained on a 12-hour light/12-hour dark cycle. The animals were handled and cared for in compliance with the relevant laws and institutional guidelines of Chonnam National University Hospital.
The lipid film was prepared with the lipid mixture at a molar ratio of 3.8% DSPE-PEG2000 : 96.2-χ% DMPC : χ% DOTAP (χ: 0%, 10%, 20%, and 40%) and at a molar ratio of 80% DMPC : 20% DOTAP with 26.3 µg of DiI. The film was then hydrated with phosphate buffered saline (PBS). The size of the liposomes was adjusted by membrane extrusion (100 nm). Dexamethasone-loaded liposomes (Dex-liposomes) were synthesized according to methods described in a previous report [16]. Briefly, a thin lipid film containing dexamethasone was prepared using a lipid mixture at a molar ratio of 3.8% DSPE-PEG2000 : 76.2% DMPC : 20% DOTAP with 22.1 µg of dexamethasone per 1 mL of liposome solution. Then, the film was hydrated with PBS and extruded using a 100 nm membrane and extrusion kit from Avanti Polar Lipids. The concentration of dexamethasone was determined by dissolving the liposome solution in methanol and analyzing it using UV spectroscopy.
C57BL/6 mice were anesthetized with an intraperitoneal injection of a mixture of zolazepam/tiletamine (Zoletil 50; Virbac, Carros, France) and xylazine (Rompun; Bayer HealthCare, Berlin, Germany). The eyes were dilated with one drop of a fixed combination of 0.5% tropicamide with 0.5% phenylephrine (Mydrin-P; Santen, Osaka, Japan), and 1.5 µL of the liposomal solution was injected into the vitreous cavity using a 31-gauge needle.
At each time point after the intravitreal injection of the liposomal solution, the mice were sacrificed, and a 10-µm frozen section of each enucleated eye was prepared. For nuclear staining, the frozen sections were fixed with 4% paraformaldehyde for 10 minutes and then stained with Hoechst 33342 (Invitrogen, Carlsbad, CA, USA). Fluorescent images of the sectioned retinas were acquired using confocal microscopy.
The murine non-arteritic ischemic optic neuropathy (NAION) model was developed using anesthetized animals as previously described [17]. Briefly, 2.5 mM of rose bengal solution (1 mL/kg) was injected intravenously, and the optic nerve of the treated eye was illuminated with a 530-nm wavelength, 300-µm laser spot for 10 seconds. Immediately following the laser illumination, an equal amount of dexamethasone solution or Dex-liposome was administered intravitreally with a Hamilton syringe (Hamilton Company, Reno, NV, USA). Four weeks after the intravitreal injection, sections containing the section site and optic nerve were obtained. For nuclear staining, the eye sections were fixed with 4% paraformaldehyde for 7 minutes and then stained with Hoechst 33342. In each group, 16 images from four eyes (the same four pixel images of each eye) were obtained to count the number of surviving retinal ganglion cells (RGCs). A retinal section of a healthy mouse was used as a positive control, and the NAION model treated with PBS was used as a negative control.
To evaluate the effect of surface charge, liposomes with various surface charges were prepared by adjusting the molar ratio of the positive lipid DOTAP. The physical properties of various liposomes are described in Table 1. Positive lipids, DOTAP and polyethylene glycol (PEG), influence the surface charge of liposomes. As the proportion of DOTAP was increased, the surface charge of the liposomes also increased. Liposomes without PEG showed a higher surface charge than did liposomes with PEG, despite having the same proportion of DOTAP.
Various kinds of liposomes loaded with DiI were injected intravitreally into the murine eye. Seventy-two hours after intravitreal injection, the mice were sacrificed, and cryosections of the enucleated eyes were prepared to evaluate the delivery of the injected liposome to the optic nerve. Liposomes with 10% or less DOTAP, 40% DOTAP, and 20% DOTAP without PEG did not reach the optic nerve (Fig. 1A, 1B). Only liposomes with 20% DOTAP and with PEG showed a significant DiI signal at the optic nerve. This meant that both a positive surface charge and PEG were required for the liposomes to reach the optic nerve.
To evaluate the correlation between retinal distribution and optic nerve delivery, we compared the DiI signal in the retina and optic nerve at the same time point. In most cases, the DiI signal appeared first in the retina and then in the optic nerve (Fig. 2A, 2B). Moreover, the highest fluorescence signal in the ganglion cell layer was observed two days after the intravitreal injection. However, the highest fluorescence signal in the optic nerve was observed three days after the injection (Fig. 2). Moreover, the fluorescence signal in the retina showed a gradation from the inner layers to the outer layers; however, the entire optic nerve showed uniform signal intensity. This implied that the delivery of liposomal payloads to the optic nerve was mediated by axonal connections with the ganglion cells rather than direct penetration of the liposomes into the optic nerve.
Next, we investigated whether this liposomal composition would have a therapeutic effect in the murine NAION model. Dexamethasone, a hydrophobic steroid, was loaded into the liposomes and injected intravitreally into the eyes of NAION model mice. Four weeks after the intravitreal injection, the number of RGCs in the same pixel area was counted. Interestingly, compared to a free dexamethasone injection (same concentration of dexamethasone as that loaded into the Dex-liposome) and control liposome injection, the Dex-liposome injection significantly prevented RGC loss in the murine NAION model (Fig. 3A, 3B).
In a previous study, we reported the optimal composition for a liposome that could diffuse well into the vitreous humor and could deliver its hydrophobic and hydrophilic payloads to the deep layers of the retina [15]. Our previous results showed that highly cationic liposomes were easily entrapped in the vitreous humor due to electrostatic interactions (generally, the vitreous humor shows a negative charge), while PEGylated liposomes with neutral and low positive surface charges were cleared out shortly after intravitreal injection. PEGylated liposomes with a moderate positive surface charge showed a uniform distribution in the vitreous humor and effective interaction with the inner retinal layers after retinal delivery.
In the present study, liposomes that were not well diffused in the vitreous humor could not deliver their payloads to the optic nerve. However, liposomes that were diffused in the vitreous humor delivered the model drug to the optic nerve effectively. This finding demonstrated that, similar to the deep layers of the retina, the injected compounds should be well diffused in the vitreous humor to be delivered to the optic nerve. Nevertheless, the following question remains: how are the liposomal payloads delivered to the optic nerve after reaching the retina? We postulated that the delivery of liposomal payloads to the optic nerve is mediated via retina-optic nerve connections, such as the nerve fiber axon or axoplasmic flow, rather than direct penetration of the liposomes into the optic nerve. The reasons for this postulation are as follows. DiI signal intensity appeared earlier in the retina than in the optic nerve. Moreover, the peak fluorescence signal in the ganglion cell layer was observed two days after intravitreal injection, but the optic nerve showed the highest value at three days (Fig. 2). Finally, the fluorescence signal in the retina moves from the inner layers to the outer layers, but the entire optic nerve shows a uniform change in signal intensity. An indepth study is needed to elucidate the exact delivery mechanism of exogenous payloads.
We tested the effectiveness of this liposomal composition for treating optic nerve disease by developing a murine model of NAION, although this model is not the same as human NAION [18]. As shown earlier, Dex-liposomes had a significant preventive effect on RGC loss. Interestingly, the free dexamethasone solution showed no therapeutic effect, implying that this liposomal delivery method can improve the delivery efficacy of the same drug concentration. This enables the use of low drug concentrations and reduces the incidence of side effects.
In conclusion, we developed a composition for liposomes that could effectively deliver drugs to the optic nerve, and these liposomes showed a significant therapeutic effect. We hope this method can be used to improve the delivery efficacy of various therapeutic compounds to the optic nerve.
Figures and Tables
Fig. 1
Delivery of liposomes with various compositions to the optic nerve (ON) 72 hours after intravitreal injection. (A) DiI-LP-20 with 17.4 mV of surface charge showed the highest fluorescence signal at the ON. (B) Fluorescence quantification of intravitreally injected liposome at the ON. Liposomes were tagged with DiI (red) to image their distribution in the ON. Nuclei were stained with Hoechst (blue). GCL = ganglion cell layer; RPE = retinal pigment epithelium. Scale bar: 100 µm. Data represent average ± SD (n = 4, ***p < 0.001, one-way analysis of variance).
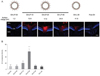
Fig. 2
Correlation between retinal distribution and optic nerve (ON) delivery of liposomal payloads (DiI). (A) Confocal microscopic images of the retina and ON. (B) Fluorescence quantification of intravitreally injected liposome at ganglion cell layer (GCL) and ON at each time point. Liposomes were tagged with DiI (red). Nuclei were stained with Hoechst (blue). RPE = retinal pigment epithelium. Scale bar: 100 µm. Data represent average ± SD (n = 4, *** p < 0.001, one-way analysis of variance).
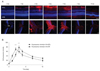
Fig. 3
Therapeutic effsssect of dexamethasone-loaded liposome (Dex-liposome) on the murine non-arteritic ischemic optic neuropathy model. (A) Confocal microscopic images of the retina four weeks after intravitreal injection of phosphate buffered saline (PBS), free dexamethasone (Dex), liposome without payloads, and Dex-liposome. (B) Statistical analysis of retinal ganglion cell (RGC) number in each group. Nuclei were stained with Hoechst (blue). Scale bar: 100 µm. Data represent average ± SD (** p < 0.01, *** p < 0.001, one-way analysis of variance).
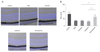
Acknowledgements
This study was financially supported by Chonnam National University (2014-2057, 2015-3037).
References
1. Tian K, Shibata-Germanos S, Pahlitzsch M, Cordeiro MF. Current perspective of neuroprotection and glaucoma. Clin Ophthalmol. 2015; 9:2109–2118.
2. Lopez Sanchez MI, Crowston JG, Mackey DA, Trounce IA. Emerging mitochondrial therapeutic targets in optic neuropathies. Pharmacol Ther. 2016; 165:132–152.


3. Bennett JL, Nickerson M, Costello F, et al. Re-evaluating the treatment of acute optic neuritis. J Neurol Neurosurg Psychiatry. 2015; 86:799–808.


4. Pula JH, Macdonald CJ. Current options for the treatment of optic neuritis. Clin Ophthalmol. 2012; 6:1211–1223.


5. Wang X, Lin J, Arzeno A, et al. Intravitreal delivery of human NgR-Fc decoy protein regenerates axons after optic nerve crush and protects ganglion cells in glaucoma models. Invest Ophthalmol Vis Sci. 2015; 56:1357–1366.


6. Jonas JB, Kreissig I, Sofker A, Degenring RF. Intravitreal injection of triamcinolone for diffuse diabetic macular edema. Arch Ophthalmol. 2003; 121:57–61.


7. Kovach JL, Schwartz SG, Flynn HW Jr, Scott IU. Anti-VEGF treatment strategies for wet AMD. J Ophthalmol. 2012; 2012:786870.


8. Honda M, Asai T, Umemoto T, et al. Suppression of choroidal neovascularization by intravitreal injection of liposomal SU5416. Arch Ophthalmol. 2011; 129:317–321.


9. Radoi C, Garcia T, Brugniart C, et al. Intravitreal triamcinolone injections in non-arteritic anterior ischemic optic neuropathy. Graefes Arch Clin Exp Ophthalmol. 2014; 252:339–345.


10. Roth DB, Verma V, Realini T, et al. Long-term incidence and timing of intraocular hypertension after intravitreal triamcinolone acetonide injection. Ophthalmology. 2009; 116:455–460.


11. Atkins EJ, Bruce BB, Newman NJ, Biousse V. Treatment of nonarteritic anterior ischemic optic neuropathy. Surv Ophthalmol. 2010; 55:47–63.


12. Ebrahim S, Peyman GA, Lee PJ. Applications of liposomes in ophthalmology. Surv Ophthalmol. 2005; 50:167–182.


13. Yasukawa T, Ogura Y, Tabata Y, et al. Drug delivery systems for vitreoretinal diseases. Prog Retin Eye Res. 2004; 23:253–281.


14. Sasaki H, Karasawa K, Hironaka K, et al. Retinal drug delivery using eyedrop preparations of poly-L-lysine-modified liposomes. Eur J Pharm Biopharm. 2013; 83:364–369.


15. Lee J, Goh U, Lee HJ, et al. Effective retinal penetration of lipophilic and lipid-conjugated hydrophilic agents delivered by engineered liposomes. Mol Pharm. 2017; 14:423–430.


16. Choksi A, Sarojini KV, Vadnal P, et al. Comparative anti-inflammatory activity of poly(amidoamine) (PAMAM) dendrimer-dexamethasone conjugates with dexamethasone-liposomes. Int J Pharm. 2013; 449:28–36.

