Abstract
Figures and Tables
Fig. 1
Representative speckle-tracking imaging examination performed for this study. (A) Representative longitudinal right ventricular (RV) strain profiles obtained from 3 segments (i.e., basal, middle, and apical) of the RV free wall (RVFW). The “VA Ferm” corresponds to pulmonary valve closure and the dotted line to the RVFW mean strain (RVFW systolic strain [StS]) curve over time. The corresponding color map below displays the change in strain over time in each RVFW segment during a single cardiac cycle. (B) Representative longitudinal RV strain rate profiles obtained from 3 segments (i.e., basal, middle, and apical) of the RVFW. The “VA Ferm” corresponds to pulmonary valve closure. The corresponding color map below displays the change in strain rate over time in each RVFW segment during a single cardiac cycle. SRS, systolic strain rate of the RVFW; SRE, early strain rate of the RVFW; SRA, late diastolic strain rate of the RVFW. (C) Representative calculations of the global RV StS and the global left ventricular (LV) StS. The global RV StS was assessed by averaging the 3 RVFW and the 3 interventricular septal (IVS) peak StS values. The global LV StS was assessed by averaging the 3 IVS and the 3 LV free wall (LVFW) peak StS values. RA, right atrium; LA, left atrium.
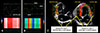
Fig. 2
Box plots showing absolute values of right ventricular (RV) free wall systolic strain (RVFW Strain), global RV systolic strain (Global RV Strain) and global left ventricular (LV) systolic strain (Global LV Strain). The box represents the interquartile range, with the median indicated by the horizontal line. The whiskers extend from the minimum to the maximum values, excluding outliers that are presented by circles or extreme outliers that are presented by asterisk.
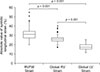
Table 1
Mean ± SD, median, minimum (Min) and maximum (Max) values, and interquartile ranges (IQR) of indices of right ventricular (RV) morphology and systolic function, and of two indices of left ventricular (LV) systolic function established in 104 healthy dogs using conventional echocardiographic and Doppler examination
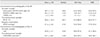
End-systolic RVFW:LVFW ratio, end-systolic RV free wall (RVFW) and LV free wall (LVFW) thicknesses ratio; RVIDD:LVIDD ratio, RV and LV internal end-diastolic diameters ratio; TAPSE, tricuspid annular plane systolic excursion; RFAC, right fractional area change; SPAP, systolic pulmonary arterial pressure; %SF, LV shortening fraction; LVESV/BSA, LV end-systolic volume indexed to the body surface area. *Assessed in 95/104 dogs (91.3%).
Table 2
Mean ± SD, median, minimum (Min) and maximum (Max) values, and interquartile ranges (IQR) of four systolic and diastolic indices of right ventricular (RV) function and of one index of left ventricular (LV) systolic function assessed in 104 healthy dogs using speckle-tracking echocardiography
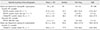
Table 3
Correlations between speckle-tracking imaging variables and either heart rate, age, or body weight, as well as conventional echocardiographic and Doppler variables assessed in a population of 104 healthy dogs
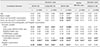
Values in bold are statistically significant. RV, right ventricular; LV, left ventricular; %SF, LV shortening fraction; End-systolic RVFW:LVFW ratio, end-systolic RV free wall (RVFW) and LV free wall (LVFW) thicknesses ratio; RVIDD/LVIDD ratio, RV and LV internal end-diastolic diameters ratio; TAPSE, tricuspid annular plane systolic excursion; LVESV/BSA: LV end-systolic volume indexed to the body surface area; RFAC, right fractional area change; SPAP, systolic pulmonary arterial pressure; StS, systolic strain; RVFW StS, longitudinal StS of the RVFW; Global RV StS, longitudinal StS of the whole RV; RVFW SRS, longitudinal systolic strain rate of the RVFW; RVFW SRE:SRA ratio, longitudinal early:late diastolic RVFW strain rate ratio; Global LV StS, longitudinal StS of the LV. *p < 0.05, statistically significant.
Table 4
Reference intervals (lower and upper limits with 90% CIs) of right ventricular (RV) conventional echocardiographic variables, as well as right and left ventricular (LV) variables assessed by speckle-tracking echocardiography
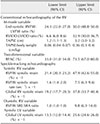
CI, confidence interval; End-systolic RVFW:LVFW ratio, end-systolic RV free wall (RVFW) and LV free wall (LVFW) thicknesses ratio; RVIDD:LVIDD ratio, RV and LV internal end-diastolic diameters ratio; TAPSE, tricuspid annular plane systolic excursion; RFAC, right fractional area change; RVFW systolic strain, longitudinal systolic strain of the RVFW; RVFW systolic strain rate, longitudinal systolic strain rate of the RVFW; Global RV systolic strain, longitudinal systolic strain of the whole RV; RVFW SRE:SRA ratio, longitudinal early:late diastolic RVFW strain rate ratio; Global LV systolic strain, longitudinal systolic strain of the LV.
Acknowledgments
References



































