Abstract
This work aimed to evaluate the effects on renal tissue integrity after hydroxyethyl starch (HES) 130/0.4 and Ringer's lactate (RL) administration in pigs under general anesthesia after acute bleeding. A total of 30 mL/kg of blood were passively removed from the femoral artery in two groups of Large White pigs, under total intravenous anesthesia with propofol and remifentanil. After bleeding, Group 1 (n = 11) received RL solution (25 mL/kg) and Group 2 (n = 11) received HES 130/0.4 solution (20 mL/kg). Additionally, Group 3 (n = 6) was not submitted to bleeding or volume replacement. Pigs were euthanized and kidneys were processed for histopathological and immunohistochemical analyses. Minimal to moderate glomerular, tubular, and interstitial changes, as well as papillary necrosis, were observed in all experimental groups. Pre-apoptosis and apoptosis indicators were higher in pigs that received HES 130/0.4, indicating a higher renal insult. Both HES 130/0.4 and RL administration may cause renal injury, although renal injury may be more significant in pigs receiving HES 13/0.4. Results also suggest that total intravenous anesthesia with propofol and remifentanil may cause renal injury, and this effect can be dose related.
It is reported that hydroxyethyl starch (HES) decreases glomerular filtration rate (GFR) [26], which is considered the main process leading to acute kidney injury (AKI) [2]. The mechanisms by which HES decreases GFR may be associated with increased oncotic pressure in the glomeruli [26]. HES with a higher molecular weight is more prone to cause renal injury than HES with a lower molecular weight [1237]. Blood hyperviscosity caused by HES administration may also compromise renal circulation and cause consequent renal ischemic injury [6].
On the other hand, crystalloid solutions increase transglomerular hydraulic pressure, leading to increased GFR [27], when compared to HES [19]. Ishikawa et al. [19] found no indication of an independent association between crystalloid total intraoperative volume and AKI, but these same authors demonstrated an association between HES administration and the development of postoperative AKI after lung resection surgery. However, hemodynamics may be improved by HES administration in patients after cardiac surgery, with similar effects in renal function as those from crystalloid solutions [22]. In addition, crystalloid solutions may cause prominent kidney injury in rats when used for resuscitation from hypovolemic shock [17].
But AKI is only developed after hours, days, or even months after renal insult takes place [2]. The evaluation of post-insult AKI is usually associated with clinical follow-up based on prerenal azotemia and serum creatinine concentrations, in accord with the AKI network classification [23], or with risk-injury-failure-loss-endstage renal disease criteria [20]. However, even with reduced GFR, prerenal azotemia may not be indicative of damage of the renal parenchyma [1].
Thus, it is possible that volume replacement solutions may cause injury in the renal parenchyma, but without post-insult-associated clinical signs of AKI. Even if crystalloid or colloid solutions are only administered for a brief period.
Therefore, the objective of the present study was to evaluate the integrity of renal parenchyma after volume replacement with HES 130/0.4 and Ringer's lactate (RL) solution (and not to evaluate the presence of AKI) after acute bleeding, in a pig model under general anesthesia.
Twenty-eight Large White pigs, three months old, were randomly selected thirty days before the beginning of the study. Pigs were housed separately under controlled temperature, humidity, air system filtration, and light/dark cycle conditions. The animals were fed a standard diet and tap water ad libitum. All procedures were approved by the Portuguese Ethics Committee for Animal Experimentation (Direção Geral de Alimentação e Veterinária; approval No. 000228).
Pigs were randomly divided into three experimental groups: Group 1 (RL solution, n = 11), Group 2 (HES 130/0.4 solution, n = 11), and Group 3 (control, n = 6).
All pigs in this study were also used in the Ortiz et al.'s study [28]. Additionally, twelve randomly selected pigs from this group were also included in two other studies by the same authors [3233] in which pigs received an intravenous 5 µg/kg remifentanil bolus before the bleeding period had started.
The material and methods related to experimental procedures of the present manuscript (anesthesia, monitoring procedures, study periods, and data collection), and the data comparing all groups (demographic, hemodynamic, body temperature, duration of the study periods, and gas analysis) are described in detail in a report by Ortiz et al. [28] and reports by Silva et al. [3233].
In brief, we summarize the methodology relevant to the present study, as originally described by Ortiz et al. [28]. Pigs were fasted overnight but they were allowed free access to water. Pigs were premedicated with intramuscular azaperone (4 mg/kg, Stresnil; Janssen Animal Health, Belgium) 30 min prior to anesthesia induction. After premedication, a 22-Ga catheter was inserted in the right auricular vein for drug and fluid administration. Anesthesia was induced with propofol (4 mg/kg, Propofol 1%; Fresenius Kabi, Germany), followed by tracheal intubation and mechanical ventilation. A propofol constant infusion rate of 15 mg/kg/h was initiated immediately after intubation and remained unchanged during the entire study. Simultaneously, a 0.3 µg/kg/min remifentanil (Ultiva; GSK, UK) constant infusion rate was initiated and maintained unchanged until invasive monitoring procedures have stopped. Subsequently, remifentanil administration was changed to a 0.2 µg/kg/min constant infusion rate until the end of the study.
An arterial catheter was inserted in the left femoral artery for the collection of blood samples, and for continuous monitoring of blood pressure. Also, a 16-Ga catheter (Abbott Animal Health, USA) was inserted in the right femoral artery for passive bleeding. Surgical approach to the ventral cervical region was performed in order to insert a 7-Fr Swan-Ganz optic catheter (Edwards Lifesciences, USA) in the cranial vena cava, via the external jugular vein.
A multiparametric hemodynamic monitor (Datex-Ohmeda S/5; GE Healthcare, Finland) was used to record body temperature, heart rate, invasive mean arterial pressure, and peripheral oxygen saturation. Data were recorded every 5 sec and stored in a computer running the Rugloop II MO + WAVES software (Demed Engineering, Belgium), via an RS-232 interface.
After completing all monitoring procedures, pigs from Groups 1 and 2 were subjected to a severe acute hemorrhage, by passive removal of 30 mL/kg of blood from the right femoral artery over a controlled period of 20 min. After the end of the bleeding, a 20 min waiting period begun, in which no additional volume was administered. After this waiting period, volume replacement was started at an infusion rate of 999 mL/h, using a 25 mL/kg RL solution (Lactato Ringer Braun; B. Braun Medical, Spain) in Group 1 and 20 mL/kg HES 130/0.4 solution (Voluven; Fresenius Kabi) in Group 2, via the catheter inserted in the cranial vena cava.
The 25 mL/kg RL solution dose used was the minimum dose necessary to obtain a similar hemodynamic response to that of HES 130/0.4 administration during volume replacement (hemodynamic response of Group 1 and Group 2 is reported in detail in Ortiz et al.'s study [28]), as determined during pretrial testing in pigs. After the end of volume replacement, pigs from Groups 1 and 2 were maintained under general anesthesia for an additional hour. Pigs from Group 3 were subjected to the same instrumentation and anesthetic procedures as those in Groups 1 and 2 but did not undergo controlled hemorrhage.
All pigs in all Groups also received an RL constant infusion rate of 6 + 1 mL/kg/h for each kg above 20 kg of weight (as described in another study [21]) during the entire anesthetic procedure in order to compensate for physiological undetected fluid loss.
Arterial blood samples were collected from the left femoral artery from pigs in Groups 1 and 2 for blood gas analyses (Instrumentation Laboratory, USA) during four periods: before beginning of bleeding; at the end of the waiting period (20 min after the end of controlled bleeding), at the end of the volume replacement period (time needed to infuse the total amount of calculated fluids); and at the end of the study. For Group 3, blood gas analyses were performed following instrumentation for monitoring procedures and at the end of the study. The time under general anesthesia for pigs in Group 3 was matched to the total time under general anesthesia for pigs in Groups 1 and 2.
Blood samples were collected from the left femoral artery before the beginning of the bleeding, and at the end of the study for determination of the blood levels of urea nitrogen (blood urea nitrogen) and creatinine. At the end of the experimental procedures, all animals were euthanatized by intravenous administration of potassium chloride (4 mmol/mL) in the cranial vena cava. A necropsy was performed and right and left kidneys were collected, rinsed in a solution of 0.9% sodium chloride, and immediately immersed in 10% neutral-buffered formalin for a maximum of 48 h for posterior analysis.
Histological analysis was performed on all animals. After fixation, the kidney samples were dehydrated through a graded ethanol series, embedded in paraffin wax, and 3 µm-thick sections were stained with H&E and with periodic acid-Schiff. Histological evaluation was performed under light microscopy (Zeiss Axioplan 2 Microscope; Zeiss, Germany) by two study-blinded pathologists. Renal damage was assessed for glomerular, tubular, interstitial and vascular lesions, and papillary necrosis following the classification previously used by other researchers [91134]. A grade from 0 to 3 was attributed for the majority of the renal lesions: Grade 0 (absence of lesion), Grade 1 (< 25% of the kidney area affected), Grade 2 (25–50% of the kidney area affected), and Grade 3 (> 50% of the kidney area affected). Tubular basement membrane irregularity was classified as regular (Score 0) or irregular (Score 1). Inflammation was assessed as absent (Grade 0), present around the atrophic tubules (Grade 1) and in more areas beyond the atrophic tubules (Grade 2).
Renal apoptosis was assessed in six animals from each experimental group through the immunoexpression of M30 and by terminal deoxynucleotidyl transferase dUTP nick end labeling (TUNEL) assay. The occurrence of pre-apoptotic changes involving the mitochondrial membrane was evaluated through the immunoexpression of cytosolic cytochrome c.
Briefly, immunohistochemistry analysis for cytochrome c detection was performed by using the Avidin-Biotin indirect method, via the streptavidin-biotin complex (ImmunoCruz goat LSAB Staining System: sc-2053; Santa Cruz Biotechnology, USA). Sections were incubated overnight at room temperature with primary antibody for cytochrome c (cytochrome c [C-20]: sc-8385; Santa Cruz Biotechnology) at a dilution of 1:500. Cytochrome c immunoexpression was scored in terms of staining intensity (0, undetectable; 1, weak; 2, moderate; 3, intense; or 4, very intense) and staining pattern (diffuse or punctate). Additionally, the percentage of immunopositive cells was semi-quantitatively assessed based on the observation of ten representative fields at a magnification of 400× in each section.
The expression of M30 was evaluated by immunofluorescence using a commercial kit (M30 Cytodeath Fluorescein; Roche, Germany) according to the manufacturer's instructions. Similar to the scoring described above for cytochrome c, immunoreactivity was scored according to the staining intensity (graded from 0 to 4), staining pattern (diffuse or punctate) and extension (percentage of positive cells semi-quantitatively assessed).
The TUNEL assay was performed by using the In Situ Cell Death Detection Kit, POD (Roche) according to the manufacturer's instructions. The number of TUNEL-positive cells (nuclei/apoptotic bodies) was assessed in 40 random high-power fields (magnification of 400×): about 20 glomerular cross-sections and 20 fields of tubulointerstitium were examined from all animals [16].
Statistical analysis was performed by using SPSS for Windows (ver. 13.0; SPSS, USA). Data were tested for a normal distribution and variance by using the Shapiro-Wilk normality test and Levene's test, respectively. Analysis of variance (ANOVA) using Bonferroni corrections for pairwise comparisons was used to analyze data with a normal distribution. If a normal distribution was not assumed, data were analyzed by using the Kruskal-Wallis test with Dunn's post-test for multiple comparisons. Continuous data are expressed as mean ± SD values or as percentage values, as appropriate; p values lower than 0.05 were considered statistically significant.
Resuming, no significant differences were observed between groups in terms of demographics, hemodynamic variables, body temperature, gas analysis, or in the duration of the different periods of the study. Additionally, no significant differences were observed in BUN and in creatinine values among groups (Table 1).
The histological analysis was performed for all animals and revealed minimal to moderate changes in the renal tissue of pigs in Groups 1 and 2. The lesions were categorized as glomerular, tubular, interstitial, and papillary (Table 2, Figs. 1,2,3).
When comparing the groups, glomerular hypercellularity was highest in Group 3 (p < 0.05), and the congestion was lowest in Group 2 (p < 0.05) (Table 2).
Pre-apoptotic events were assessed through examination of the immunoexpression of cytochrome c. Apoptosis was assessed through examination of the immunoexpression of M30 and by performing the TUNEL assay. These analyses were performed in six animals from each experimental group.
All epithelial cells (100%) of the proximal and distal convoluted tubules from all experimental groups exhibited punctate cytoplasmic staining for cytochrome c but with variable intensity. Similarly, all epithelial cells of the thick segment of Henle's loop were immunopositive for cytochrome c in all experimental groups, with an intensity mainly classified as 4 (very intense) (Table 3, Fig. 4). Glomeruli and transitional epithelium were negative for cytochrome c antibody in all experimental groups.
Regarding the thin segment of Henle's loop, three animals in Group 1 (50%) presented punctate cytoplasmic immunolabeling for cytochrome c, with 50% to 75% of immunopositive cells, and a staining intensity varying between weak and moderate. In Group 2, six animals (100%) presented similar punctate cytoplasmic immunolabeling for cytochrome c as that in Group 1. In Group 3, the thin segment of Henle's loop was negative for cytochrome c antibody in all animals (Table 3).
All animals from Group 1 exhibited an immunoreactivity for cytochrome c in 75% of the collecting tubules' cells, changing from weak to moderate. In Group 2, immunoreactivity was observed in 75% to 100% of the collecting tubule cells, and with similar staining intensity as that in Group 1. Cells from collecting tubules presented an immunopositivity of less than 25% in four animals, and of 75% in the other two animals, with a staining intensity varying from moderate to very intense (Table 3).
Glomeruli, distal convoluted tubules, thin segment of Henle's loop, and transitional epithelia were negative for M30 in all experimental groups. All animals from Group 1 exhibited a punctate cytoplasmic immunopositivity for M30 in all epithelial cells of proximal convoluted tubules, with an intensity varying from weak to intense. Animals in Group 2 exhibited a similar cytoplasmic immunopositivity, but with a higher level of staining intensity, varying from intense to very intense. In Group 3, only one animal presented immunopositivity of proximal convoluted tubules' cells for M30, with a weak intensity (Table 4, Fig. 4).
Only one animal from Group 1 exhibited immunopositivity of epithelial cells of the thick segment of Henle's loop for M30, with a weak intensity, and none from the control group was positive. On the other hand, four animals from this group exhibited immunopositivity of all cells of the thick segment of Henle's loop for M30, with an intensity varying from moderate to intense. The cells of the thick segment of Henle's loop were negative for M30 in all animals from Group 3 (Table 4, Fig. 4).
When considering collecting tubules, four animals in Group 2 exhibited an immunopositivity of less than 25% in the cells of collecting tubules, with a weak intensity. All animals from Group 2 exhibited an immunopositivity of less than 25% in the cells of collecting tubules, with an intensity varying from moderate to intense. One animal in Group 3 exhibited an immunopositivity of less than 25% in the cells of collecting tubules, with a weak intensity (Table 4, Fig. 4).
no differences were observed between groups in the glomeruli, nor in the interstitial tissue. The number of positive cells in renal tubules was lower in Group 1 than in Group 3 (p < 0.05). Although the difference in the immunopositive cells between Group 1 and Group 2 did not achieve statistical difference, it is very important to note the huge difference in the cells marked in these two groups (281 [Group 1]) vs. 1,598 [Group 2]) (Table 5, Fig. 4).
Our study addressed the effects of RL and HES 130/0.4 administration on renal tissue in a pig hemorrhagic model under general anesthesia. In general, the histopathological renal lesions were discrete in both Group 1 and Group 2. On the other hand, immunohistochemistry analyses suggested that RL administration may be more effective than HES 130/0.4 in preserving the integrity of kidney cells after severe acute blood loss, by decreasing the level of pre-apoptotic and apoptotic events.
It has been reported that remifentanil and propofol itself, or combined with other drugs, may cause renal injury in healthy human patients [51035]. Additionally, all pigs from all groups of our study were premedicated with azaperone, and this drug accumulates in pigs' kidneys and may also contribute to kidney damage [372438]. A significant blood loss followed by volume replacement causes hemodilution. While maintaining constant the drugs' infusion rates, the propofol and remifentanil plasma concentrations may have decreased in Groups 1 and 2 due to this hemodilution. The histological lesions observed in pigs from Group 3, although less severe when compared with the bleeding groups, suggests that using higher plasmatic concentrations of propofol and remifentanil during total intravenous anesthesia may induce a higher renal damage, despite keeping all cardiovascular and ventilatory parameters within their normal range of values.
The inclusion of a positive control group in our study, where bleeding would have also occurred but was not followed by volume replacement, would have provided significant information about possible parenchymal renal lesions due to the hypoperfusion itself. However, it is known that transient ischemia due to hypovolemia is a common cause of renal injury [15], particularly due to lesions caused in proximal tubular epithelial cells, highly sensitive to acute ischemia [4], which, in turn, would cause a reduction in the GFR due to an increased backleak mechanism [25]. On the other hand, propofol infusions may cause a significant decrease in renal perfusion [29], an important fact to consider when performing a renal histological evaluation after more than four hours of total intravenous anesthesia with propofol, as occurred with all pigs in our study. Thus, Group 3 in our study was used to provide information about the influence of prolonged total intravenous anesthesia with propofol on renal tissue.
When analyzing renal lesions individually, it was observed that the animals from all groups exhibited lesions suggestive of acute (hyaline casts, degeneration) and chronic processes. The fibrosis and inflammatory infiltrate, which were observed in all experimental groups but more frequently in Group 3, may be associated with background chronic processes and not related to the experimental study. On the other hand, the acute lesions, namely hyaline casts and degeneration, may have occurred because of decreased glomerular filtration or tubular damage.
Alterations in glomerular dynamics are the basis for the development of acute renal failure, as they interfere with the net transglomerular hydraulic pressure. This can be reduced by a decrease in the hydraulic pressure in the glomerular capillary [27]. The GFR is dependent on adequate renal perfusion. As kidneys receive up to 25% of the cardiac output, any compromise of the general circulation or intrarenal circulation, for example by hypovolemia following acute hemorrhage (as in the present study), impaired cardiac output, severe pulmonary hypertension, or sepsis, may cause prerenal azotemia [2]. But, prerenal azotemia is not indicative of damage to the renal parenchyma, even with reduced GFR [1].
Ishikawa et al. [19] reported that colloid infusions (10% pentastarch 250/0.45, and 6% HES 130/0.4) may cause postoperative AKI after lung resection surgery in a dose-dependent effect. In fact, according to these authors, the odds of AKI development increase 1.5-fold with each 250 mL aliquot of HES. However, it is important to consider that 94% of the patients involved in the Ishikawa et al.'s study [19] were ASA II and III, and with a risk of developing postoperative AKI. On the other hand, Magder et al. [22] reported that, when compared to a crystalloid solution, HES improved hemodynamics in patients after cardiac surgery, and without an increase in renal dysfunction.
HES with a higher molecular weight may cause more pronounced renal injury than HES with a lower molecular weight [1237] by either causing higher hyperviscosity with resulting renal ischemic injury [6] or by more significantly decreasing the GFR due to a hyperoncotic glomerular status, with a reduction in the glomerular filtration fraction [26].
In the present study, pigs were healthy individuals, but it is very likely that after acute hemorrhage, a decrease in the GFR has occurred [2]. However, BUN and creatinine values were within physiologic range during the entire study, supporting the observation that even in conditions of decreased GFR, prerenal azotemia is not indicative of damage to the renal parenchyma [1]. Nevertheless, BUN and creatinine values in the present study must be interpreted carefully, because even in the presence of AKI, a rapid decline in glomerular filtration usually occurs after hours or days [2]. Therefore, the objective of the present study was to evaluate the integrity of renal tissue in a clinical situation under general anesthesia, following volume replacement with a colloid or crystalloid solution after acute hemorrhage and not to evaluate the effect on renal function. Also, in the present study, pigs that received HES 130/0.4 showed a significant degree of pre-apoptotic and apoptotic insult to kidney cells, when compared to those receiving the RL solution. It is expected that the higher the kidney insult, the higher is the probability of compromising renal function, during the hours or days after injury. But, it is important to note that impairment of renal function may not occur, despite kidney injury has been caused.
Apoptosis is a physiological gene-driven mode of cell death characterized by typical morphological changes (shrinkage of the cell and nucleus, fragmentation into membrane-bound apoptotic bodies) and DNA fragmentation [31]. Although it has a very important role in renal pathophysiology, it may be activated by several factors that may lead to a considerable loss of cells causing a decrease/loss in renal function [16]. Despite their great ability for self-regulation, kidneys are extremely sensitive to hypoperfusion leading to acute renal failure [13]. Blood loss is a frequent clinical condition following a serious traumatic event or a major surgery, which may lead to ineffective tissue perfusion of vital organs (hypoperfusion), such as the kidneys [14], and consequently to nutritional and oxidative stress [13]. The markers of pre-apoptosis (cytochrome c) or apoptosis (M30 and TUNEL) were evaluated in all experimental groups.
Cytochrome c is essential for mitochondrial electron transport and intrinsic type II apoptosis. It is now generally accepted that the release of cytochrome c into the cytosol, where it binds with apoptotic protease-activating factor 1, is a key step in the apoptotic cascade [1836]. The moderate to intense immunoreaction present in the convoluted tubules and the thick segment of Henle's loop, in the animals from Groups 1 and 3 could have been due to the elevated number of mitochondria present in these structures. However, the higher intensity of reaction exhibited by the same structures in the animals from Group 2 could be related to the release of cytochrome c into the cytosol following pre-apoptotic stimuli. This finding is suggestive of a higher degree of insult to the cells present in the structures mentioned above in animals that received HES 130/0.4 when compared with the animals in which RL solution was used. In addition, the mildly increased intensity of reaction present in the thin segment of Henle's loop, as well as the mildly increased numbers of cells marked in the convoluted tubules, are supportive of a higher degree of insult to the cellular component of these structures in animals that received HES 130/0.4.
M30 is a caspase-cleaved product of keratin 18, a type I intermediate filament protein and a major component of single-layer and glandular epithelial cells. It is released into circulation during apoptotic events of malignant and normal epithelial cells. M30 is expressed during early apoptosis, and its assessment is considered a promising alternative to the TUNEL assay for the detection of apoptotic cells [830]. The low or moderated reaction present in convoluted tubules in Group 1, could be justified by the effect of anesthesia and hypoperfusion, compared with Group 3, in which immunopositivity of proximal convoluted tubules' cells for M30 was almost absent. The higher intensity exhibited by the same structures in Group 2 indicates a higher renal insult in animals that have received HES 130/0.4. Also, the higher staining intensity present in the thin segment of Henle's loop, as well as the higher number of cells marked in proximal convoluted tubules' cells support this conclusion. The results obtained with M30 showed high specificity to apoptotic events, suggesting higher apoptosis in kidney cells after volume replacement with HES 130/0.4 when compared to the administration of RL solution.
TUNEL method relies on the presence of nicks in the DNA which may be identified by terminal deoxynucleotidyl transferase [10]. The number of animals studied was six in each group. This small number of animals resulted in high SD values. The results in our study indicate a possible protective effect of RL solution on renal tubules, by decreasing the number of apoptotic cells. As mentioned in the results section, although the difference in the immunopositive cells between Groups 1 and 2 did not achieve statistical difference, it is very important to note the enormous difference in the number of cells marked in these two groups (281 [Group 1] vs. 1,598 [Group 2]).
This study addressed kidney injury associated with RL or HES 130/0.4 administration over a brief period of time. Animals that received HES 130/0.4 presented a higher degree of pre-apoptotic and apoptotic insult to the kidney cells when compared to those receiving RL solution. Thus, short-term administration of HES 130/0.4 for volume replacement after severe blood loss during general anesthesia with propofol and remifentanil, may cause a significantly higher degree of renal injury, when compared to RL.
Additionally, our results suggest that total intravenous anesthesia with propofol and remifentanil may cause kidney injury, despite all cardiovascular and ventilatory parameters being maintained within physiological normal range values.
Figures and Tables
Fig. 1
Representative sections of kidney showing glomerular lesions. Glomerular hypercellularity (Grade 2, A; Grade 1, B), hyaline droplet degeneration in Bowman's space (Grade 2, C; Grade 1, D), and mesangial expansion (Grade 2, E; Grade 1, F) in Group 1 (Ringer's lactate-treated) (A, C, and E) and Group 2 (hydroxyethyl starch 130/0.4) (B, D, and F). Periodic acid-Schiff stain (A–F). Scale bars = 25 µm (A–F).
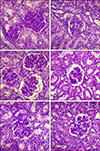
Fig. 2
Representative sections of kidney showing tubular and interstitial lesions. Tubular blebbing of renal tubules (Grade 3, A; Grade 2, B), hyaline casts (Grade 1, C and D), hyaline material deposits (Grade 1, E and F) and interstitial fibrosis and tubular atrophy (Grade 2, G; Grade 1, H) in Group 1 (Ringer's lactate) (A, C, E, and G) and Group 2 (hydroxyethyl starch 130/0.4) (B, D, F, and H). Periodic acid-Schiff stain (A–F). H&E stain (G and H). Scale bars = 25 µm (A, B, G, and H), 50 µm (C–F).
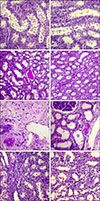
Fig. 3
Representative sections of kidney with vascular lesions. Edema (Grade 2, A), congestion (Grade 1, B), hemorrhage (Grade 2, C) and hyperemia (Grade 2, D) in Group 1 (Ringer's lactate) (A and C) and Group 2 (hydroxyethyl starch 130/0.4) (B and D). Periodic acid-Schiff stain (A). H&E stain (B–D). Scale bars = 25 µm (A, B, and D), 100 µm (C).
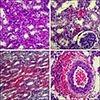
Fig. 4
Immunoreactivity results for cytochrome c (A–C), M30 (D–F), and terminal deoxynucleotidyl transferase dUTP nick end labeling (TUNEL) (G–I) in all experimental groups. (A) Very intense cytoplasmic immunolabeling in convoluted tubules in Group 1 (Ringer's lactate). (B) Very intense cytoplasmic immunolabeling in convoluted tubules in Group 2 (hydroxyethyl starch 130/0.4). (C) Moderate cytoplasmic immunolabeling in convoluted tubules in Group 3 (control). (D) Moderate immunolabeling in proximal convoluted tubules in Group 1. (E) Intense immunolabeling in collecting tubules in Group 2. (F) Absence of immunolabeling in collecting tubules in Group 3. (G) Weak immunostaining in convoluted tubules in Group 1. (H) Intense nuclear immunostaining in convoluted tubules in Group 2. (I) Negative immunostaining in Group 3. H&E stain (A–I). Scale bars = 25 µm (A–C and G–I). 20× (D and F), 40× (E).
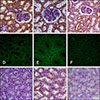
Table 1
Blood values of blood urea nitrogen (BUN) and creatinine before bleeding and after the end of the study

Table 2
Histopathological evaluation of renal damage in all experimental groups (number and percentage of animals affected in each experimental group)
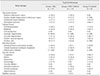
Table 3
Percentage of immunopositive cells semi-quantitatively assessed, as well as staining pattern and staining intensity for cytochrome c in all experimental groups
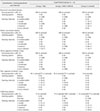
Table 4
Percentage of immunopositive cells semi-quantitatively assessed, as well as staining pattern and staining intensity for M30 in all experimental groups
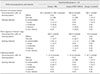
Acknowledgments
We would like to thank the Instituto Politécnico de Viseu and CI&DETS, UTAD and CITAB, and Évora University and ICAAM for their support. This work was financed by national funds through FCT - Fundação para a Ciência e Tecnologia, under projects UID/Multi/04016/2016, and UID/AGR/04033/2013. This work was also supported by European Investment Funds by FEDER/COMPETE/POCI - Operational Competitiveness and Internationalization Programme, under Project POCI-01-0145-FEDER.
References
1. Badr KF, Ichikawa I. Prerenal failure: a deleterious shift from renal compensation to decompensation. N Engl J Med. 1988; 319:623–629.


2. Basile DP, Anderson MD, Sutton TA. Pathophysiology of acute kidney injury. Compr Physiol. 2012; 2:1303–1353.


3. Bock C, Stachel CS. Development and validation of a confirmatory method for the determination of tranquilisers and a β-blocker in porcine and bovine kidney by LC-MS/MS. Food Addit Contam Part A Chem Anal Control Expo Risk Assess. 2013; 30:1000–1011.


4. Bonegio R, Lieberthal W. Role of apoptosis in the pathogenesis of acute renal failure. Curr Opin Nephrol Hypertens. 2002; 11:301–308.


5. Casserly B, O'Mahony E, Timm EG, Haqqie S, Eisele G, Urizar R. Propofol infusion syndrome: an unusual cause of renal failure. Am J Kidney Dis. 2004; 44:e98–e101.


6. Castro VJ, Astiz ME, Rackow EC. Effect of crystalloid and colloid solutions on blood rheology in sepsis. Shock. 1997; 8:104–107.


7. Cerkvenik-Flajs V. Determination of residues of azaperone in the kidneys by liquid chromatography with fluorescence detection. Anal Chim Acta. 2007; 586:374–382.


8. Cevatemre B, Ulukaya E, Sarimahmut M, Oral AY, Frame FM. The M30 assay does not detect apoptosis in epithelial-derived cancer cells expressing low levels of cytokeratin 18. Tumour Biol. 2015; 36:6857–6865.


9. de Azevedo VLF, Santos PSS, de Oliveira GS Jr, Módolo GP, Domingues MAC, Castiglia YMM, Vianna PTG, Vane LA, Módolo NSP. The effect of 6% Hydroxyethyl starch vs. Ringer's lactate on acute kidney injury after renal ischemia in rats. Acta Cir Bras. 2013; 28:5–9.
10. Demir A, Yılmaz FM, Ceylan C, Doluoglu OG, Uçar P, Züngün C, Guclu CY, Ünal U, Karadeniz U, Günertem E, Lafci G, Çağlı K, Özgök A. A comparison of the effects of ketamine and remifentanil on renal functions in coronary artery bypass graft surgery. Ren Fail. 2015; 37:819–826.


11. Ebcioglu Z, Cohen DJ, Crew RJ, Hardy MA, Ratner LE, D'Agati VD, Markowitz GS. Osmotic nephrosis in a renal transplant recipient. Kidney Int. 2006; 70:1873–1876.


12. Ertmer C, Köhler G, Rehberg S, Morelli A, Lange M, Ellger B, Pinto BB, Rübig E, Erren M, Fischer LG, Van Aken H, Westphal M. Renal effects of saline-based 10% pentastarch versus 6% tetrastarch infusion in ovine endotoxemic shock. Anesthesiology. 2010; 112:936–947.


13. Flemming B, Seeliger E, Wronski T, Steer K, Arenz N, Persson PB. Oxygen and renal hemodynamics in the conscious rat. J Am Soc Nephrol. 2000; 11:18–24.


14. Hammond KL, Margolin DA. Surgical hemorrhage, damage control, and the abdominal compartment syndrome. Clin Colon Rectal Surg. 2006; 19:188–194.


16. Hughes J, Gobe G. Identification and quantification of apoptosis in the kidney using morphology, biochemical and molecular markers. Nephrology (Carlton). 2007; 12:452–458.


17. Hussmann B, Lendemans S, de Groot H, Rohrig R. Volume replacement with Ringer-lactate is detrimental in severe hemorrhagic shock but protective in moderate hemorrhagic shock: studies in a rat model. Crit Care. 2014; 18:R5.


18. Hüttemann M, Pecina P, Rainbolt M, Sanderson TH, Kagan VE, Samavati L, Doan JW, Lee I. The multiple functions of cytochrome c and their regulation in life and death decisions of the mammalian cell: from respiration to apoptosis. Mitochondrion. 2011; 11:369–381.


19. Ishikawa S, Griesdale DE, Lohser J. Acute kidney injury after lung resection surgery: incidence and perioperative risk factors. Anesth Analg. 2012; 114:1256–1262.
20. Lopes JA, Fernandes P, Jorge S, Gonçalves S, Alvarez A, Costa e Silva Z, França C, Prata MM. Acute kidney injury in intensive care unit patients: a comparison between the RIFLE and the Acute Kidney Injury Network classifications. Crit Care. 2008; 12:R110.


21. Madjdpour C, Dettori N, Frascarolo P, Burki M, Boll M, Fisch A, Bombeli T, Spahn DR. Molecular weight of hydroxyethyl starch: is there an effect on blood coagulation and pharmacokinetics? Br J Anaesth. 2005; 94:569–576.


22. Magder S, Potter BJ, Varennes BD, Doucette S, Fergusson D. Fluids after cardiac surgery: a pilot study of the use of colloids versus crystalloids. Crit Care Med. 2010; 38:2117–2124.


23. Mehta RL, Kellum JA, Shah SV, Molitoris BA, Ronco C, Warnock DG, Levin A. Acute Kidney Injury Network: report of an initiative to improve outcomes in acute kidney injury. Crit Care. 2007; 11:R31.


24. Mitrowska K, Posyniak A, Zmudzki J. Rapid method for the determination of tranquilizers and a beta-blocker in porcine and bovine kidney by liquid chromatography with tandem mass spectrometry. Anal Chim Acta. 2009; 637:185–192.


26. Moran M, Kapsner C. Acute renal failure associated with elevated plasma oncotic pressure. N Engl J Med. 1987; 317:150–153.


27. Myers BD, Carrie BJ, Yee RR, Hilberman M, Michaels AS. Pathophysiology of hemodynamically mediated acute renal failure in man. Kidney Int. 1980; 18:495–504.


28. Ortiz AL, Vala H, Venâncio C, Mesquita J, Silva A, Gonzalo-Orden JM, Ferreira D. The influence of Ringer's lactate or HES 130/0.4 administration on the integrity of the small intestinal mucosa in a pig hemorrhagic shock model under general anesthesia. J Vet Emerg Crit Care (San Antonio). 2017; 27:96–107.


29. Piriou V, Chiari P, Lehot JJ, Foëx P, Arvieux CC. Effects of propofol on haemodynamics and on regional blood flows in dogs submitted or not to a volaemic expansion. Eur J Anaesthesiol. 1999; 16:615–621.


30. Saigusa S, Inoue Y, Tanaka K, Okugawa Y, Toiyama Y, Uchida K, Mohri Y, Kusunoki M. Lack of M30 expression correlates with factors reflecting tumor progression in rectal cancer with preoperative chemoradiotherapy. Mol Clin Oncol. 2014; 2:99–104.


31. Saraste A, Pulkki K. Morphologic and biochemical hallmarks of apoptosis. Cardiovasc Res. 2000; 45:528–537.


32. Silva A, Ortiz AL, Venâncio C, Souza AP, Ferreira LM, Branco PS, de Pinho PG, Amorim P, Ferreira DA. Effects of acute bleeding followed by hydroxyethyl starch 130/0.4 or a crystalloid on propofol concentrations, cerebral oxygenation, and electroencephalographic and haemodynamic variables in pigs. Vet Med Int. 2014; 2014:710394.


33. Silva A, Venâncio C, Ortiz AL, Souza AP, Amorim P, Ferreira DA. The effect of high doses of remifentanil in brain near-infrared spectroscopy and in electroencephalographic parameters in pigs. Vet Anaesth Analg. 2014; 41:153–162.


34. Tervaert TW, Mooyaart AL, Amann K, Cohen AH, Cook HT, Drachenberg CB, Ferrario F, Fogo AB, Haas M, de Heer E, Joh K, Noël LH, Radhakrishnan J, Seshan SV, Bajema IM, Bruijn JA. Pathologic classification of diabetic nephropathy. J Am Soc Nephrol. 2010; 21:556–563.


35. Vianna PT, Castiglia YM, Braz JR, Viero RM, Beier S, Vianna Filho PT, Vitória A, Reinoldes Bizarria Guilherme G, de Assis Golim M, Deffune E. Remifentanil, isoflurane, and preconditioning attenuate renal ischemia/reperfusion injury in rats. Transplant Proc. 2009; 41:4080–4082.


36. Wang X. The expanding role of mitochondria in apoptosis. Genes Dev. 2001; 15:2922–2933.
37. Winkelmayer WC, Glynn RJ, Levin R, Avorn J. Hydroxyethyl starch and change in renal function in patients undergoing coronary artery bypass graft surgery. Kidney Int. 2003; 64:1046–1049.


38. Zawadzka I, Rodziewicz L. [Determination of azaperone and carazolol residues in animals kidney using LC-MS/MS method]. Rocz Panstw Zakl Hig. 2009; 60:19–23. Polish.