Abstract
Background and Objectives
Chronic impairment of β-adrenergic receptor signaling increases cardiac apoptosis, hypertrophy and fibrosis. The aim of this study was to investigate whether isoproterenol (ISO), an agonist of the adrenergic receptor, can enhance tumor necrosis factor-related apoptosis-inducing ligand (TRAIL)-induced apoptosis in human embryonic kidney (HEK) 293 cells.
Materials and Methods
HEK 293 cells were treated with ISO and/or TRAIL for 24 hours. Cell viability was evaluated by microscopy and an established viability assay, and apoptotic cell death was analyzed by staining with fluorescein isothiocynate-annexin-V/propidium iodide (PI) and caspase activation. To confirm the mechanism of cell death induced by co-treatment with ISO and TRAIL, expression of TRAIL receptor 2 (death receptor 5, DR5) was evaluated by immunoblotting.
Results
Although ISO or TRAIL treatment decreased HEK 293 cell viability by 13% and 17%, respectively, co-treatment with ISO and TRAIL resulted in a markedly higher death rate of 35% after 24 hours. Increases were evident in early apoptotic cells (i.e., annexin-V positive/PI negative; 19.4%), late apoptotic cells (i.e., annexin-V positive/PI positive; 6.3%) and dead cells (i.e., annexin-V negative/PI positive; 1.1%) when cells were co-treated with ISO and TRAIL, compared to cells treated with either ISO or TRAIL. In addition, marked increases of cleaved cas-3, cleaved poly (adenosine diphosphate-ribose) polymerase and DR5 were observed in HEK 293 cells co-treated with ISO and TRAIL.
Heart disease remains the leading cause of death worldwide. The heart has a limited capacity for regeneration and repair. Cell death is a hallmark of various heart diseases, including heart failure (HF), myocardial infarction (MI), and ischemia/reperfusion (I/R).1) Cardiac myocytes express Fas cell surface death receptor (FAS) and tumor necrosis factor (TNF) receptor 1, which have both been implicated in cardiovascular pathology through stimulation of FAS ligand, TNF-α, or TNF-related apoptosis-inducing ligand (TRAIL).1) TRAIL, a member of the TNF superfamily, is expressed either as a transmembrane protein on the cell surface of a variety of cell types or is released as a soluble protein.2) In humans, four membrane-bound TRAIL receptors have been identified. Among these, TRAIL-R1 (death receptor [DR]-4) and TRAIL-R2 (DR5) are apoptosis-inducing receptors, and TRAIL-R3 (decoy receptor [DcR] 1) and TRAIL-R4 (DcR2) act as decoy receptors because of absent or nonfunctional death domains.3) TRAIL binds to the death receptors DR4 and DR5, and triggers the apoptosis signaling pathway by recruiting Fas-associated death domain (FADD), subsequently activating caspase-8. Caspase-8 activates executioner caspases, such as caspase-3, -6, and -7, leading to cleavage of numerous intracellular proteins.4) Unlike FasL and TNF-α, TRAIL selectively induces cell death in malignant cells but not in normal cells5) because of the relative selectivity of DR4 and DR5 for malignant cells over normal or healthy cells.6)7) However, up-regulation of DR4 or DR5 in normal cells can induce apoptosis by stimulating TRAIL. Numerous signaling molecules trigger DR5 and DR4 induction, including activation of mitogen-activated protein kinases (MAPKs) and the binding of the CCAAT/enhancer binding protein (C/EBP) homologous protein (CHOP) to the DR5 promoter.8)
β-Adrenergic receptors (β-AR) play key roles in the regulation of cardiac function, both under normal conditions and under pathological conditions, such as during HF.9) Chronic impairment of β-AR signaling contributes to alterations in cardiac structure through increased apoptosis, hypertrophy, fibrosis and steadily decreased contractile function as HF progresses.10)11) Isoproterenol (ISO), an agonist of the adrenergic receptor, induces compromised and unstable cardiac hemodynamics and aggravates cell death, thereby inducing HF.12)13) Several related signaling pathways including those involved in oxidative stress, calcium dysregulation and activation of apoptotic genes contribute to ISO-induced heart damage. Apoptosis is a critical step in provoking systolic dysfunction and congestive HF.14) Clinical studies have demonstrated that adrenergic blockers significantly improve cardiac function and reduce cardiac apoptosis in patients with HF.15) However, the mechanism of ISO action in TRAIL-induced cardiac apoptosis remains unknown.
Therefore, we investigated whether ISO enhanced TRAIL-induced apoptosis in human embryonic kidney (HEK) 293 cells. In this study, we report that TRAIL-induced apoptosis is synergistically increased by ISO through the up-regulation of DR5.
Human embryonic kidney (HEK 293) cells were cultured in Dulbecco's modified Eagle's medium (DMEM; Gibco BRL, Rockville, MD, USA) supplemented with 10% fetal bovine serum (FBS; Sigma-Aldrich, St. Louis, MO, USA), streptomycin (50 µg/mL) and penicillin (100 U/mL). Cells were incubated at 37℃ in a humidified atmosphere of 5% CO2.
HEK 293 cells were plated at 1×104 cells/cm2 in 96-well plates. After 24 hours, the medium was changed to LG-DMEM, and ISO (100 µM) and/or TRAIL (100 ng/mL) were added. Cells were cultured in each condition for an additional 24 hours. Then, 0.5 mg of methylthiazolyldiphenyl-tetrazolium bromide (MTT: Sigma-Aldrich, San Diego, CA, USA) dissolved in phosphate-buffered saline (PBS) was added to each well (final concentration, 5 mg/mL),16) and cells were incubated at 37℃ for 1 hour. MTT formazan that formed was dissolved in 100 µL dimethylsulfoxide and incubation was continued for an additional 15 minutes with stirring before the optical density of each well at 570 nm was determined using a microplate reader.
HEK 293 cells were treated with ISO (100 µM) and/or TRAIL (100 ng/mL) for 24 hours. Cells were harvested, washed with ice-cold PBS and solubilized using lysis buffer (0.1% sodium dodecyl sulfate [SDS], 1% Triton X-100, 50 mM Tris-HCl [pH 7.4], 150 mM NaCl) containing Halt Protease and Phosphatase Inhibitor Cocktail (Thermo Scientific, Rockford, IL, USA). Proteins were separated by 10% SDS-polyacrylamide gel electrophoresis (SDS-PAGE) and transferred to an Immobilon membrane. Each membrane was blocked with 5% skim milk in TBST (Tris-HCl buffered saline containing 0.1% Tween 20) and incubated with primary antibodies against caspase (Cas)-3, cleaved Cas-3, and poly (ADP-ribose) polymerase (PARP; 1:1000; Cell Signaling Technology, Danvers, MA, USA), DR5 (1:1000, R&D Systems, Minneapolis, MN, USA) and glyceraldehydes-3-phosphate dehydrogenase (GAPDH; 1:1000; Santa Cruz Biotechnology, Santa Cruz, CA, USA). Bound primary antibodies were detected with horseradish peroxidase-conjugated secondary antibody (1:2000; Santa Cruz Biotechnology, Santa Cruz, CA, USA), treated with EZ-Western Lumi Pico (DOGEN, Seoul, Korea) and visualized using the FluorChem FC2 system (Alpha Innotech, Santa Clara, CA, USA).
A fluorescein isothiocynate (FITC)-annexin-V apoptosis detection kit (BD Biosciences, Franklin Lakes, NJ, USA) was used according to the manufacturer's instructions. Cells were harvested, washed twice with cold PBS and re-suspended in 1xbinding buffer. Cells were stained with FITC-annexin-V and PI for 15 minutes at room temperature in the dark. Cells were analyzed on a flow cytometer without washing within 1 hour of the completion of staining.
To investigate whether ISO can enhance TRAIL-induced apoptosis, HEK 293 cells were treated with ISO (100 µM) and TRAIL (100 ng/mL) for 24 hours. Cell viability of the treated cells was examined by light microscopy, and cytotoxicity of ISO and/or TRAIL was quantified using the MTT assay (Fig. 1). ISO or TRAIL treatment decreased the viability of HEK 293 cell populations by 13% and 17%, respectively (Fig. 1B). However, co-treatment with ISO and TRAIL resulted in the cell death of 35% of HEK 293 cells after 24 hours. This result suggested that ISO enhances TRAIL-induced apoptosis in HEK 293 cells.
To further confirm that co-treatment with ISO and TRAIL is cytotoxic, apoptosis in HEK 293 cells was evaluated by flow cytometry using annexin-V and PI. Increases were apparent in early apoptotic cells (i.e., annexin-V positive/PI negative; 19.4%), late apoptotic cells (i.e., annexin-V positive/PI positive; 6.3%) and dead cells (i.e., annexin-V negative/PI positive; 1.1%) when cells were co-treated with ISO and TRAIL, compared to cells treated with ISO or TRAIL (Fig. 2A and B). Next, caspase-3 activation and PARP degradation were examined using an immunoblotting assay. Both events are commonly observed in apoptotic cell death. Precursor Cas-3 (32 kDa) can be cleaved into 17 kDa and 12 kDa fragments, resulting in activation. PARP can be cleaved by caspases, which separates the PARP amino-terminal DNA binding domain (24 kDa) from the carboxy-terminal catalytic domain (89 kDa) in response to apoptotic signals. Substantial amounts of the 17 kDa cleaved cas-3 and 89 kDa cleaved PARP were observed in HEK 293 cell preparations co-treated with ISO and TRAIL (Fig. 2C and D). These results suggested that ISO and TRAIL synergistically induce apoptosis in HEK 293 cells.
TRAIL (1 mg/mL) increases the level of DR5, but not DR4, in HEK 293 cells.17) We investigated whether DR5 could be up-regulated by co-treatment with ISO and TRAIL in HEK 293 cells. The cells were treated with ISO (100 µM) and/or TRAIL (100 ng/mL) for 24 hours. The expression of DR5 protein increased in cells co-treated with ISO and TRAIL, although individual treatment with ISO or TRAIL did not induce increased DR5 expression (Fig. 3). Taken together, the results indicated that co-treatment with ISO with TRAIL may be responsible for death of HEK 293 cells through DR5 up-regulation.
We report that co-treatment with ISO and TRAIL enhances HEK 293 cell death through DR5 up-regulation, although individual treatment with either ISO or TRAIL does not increase DR5 expression. This implies an important finding with respect to understanding the mechanism of cell death induced by β-AR activation. Contrary to other TNF-family members, membrane-bound TRAIL is conditionally expressed in immune cells, such as natural killer cells, B cells, monocytes and dendritic cells following cytokine stimulation.18)19)20)21) Intracellular stores of TRAIL have also been found in polymorphonuclear neutrophils22)23) and are released in response to a variety of stimuli.24)25) Moreover, β-AR activation induces inflammatory responses in the heart; in vivo ISO infusion activates nuclear factor-κB (NF-kB), κB-responsive TNF-α, and interleukin-1β, and -6 in the heart.17)26) TRAIL is released by cardiac myocytes,27) but no additional information is available about the effect of TRAIL on the heart. Taken together, the prior and current results suggest that inflammation induced by β-AR activation may be responsible for TRAIL expression in cardiac myocytes and activated immune cells. Subsequently, β-AR activation and TRAIL expression induce cardiac cell death through DR5 up-regulation, which promotes apoptosis.
Various mechanisms of DRs up-regulation have been reported.28) Briefly, production of reactive oxygen species (ROS), expression of CCAAT/enhancer-binding protein -homologous protein, p53, Sp1 and Yin Yang 1 (YY1), and activation of extracellular signal-regulated kinase, c-jun N-terminal kinase and NF-κB regulate expression of DRs.28) In particular, TRAIL induces DR5 expression in several cell types. The TNF family including TRAIL induces ROS production.29)30) ROS regulate DRs through modulation of various proteins including p53.28) In addition, TRAIL (1 mg/mL) increases expression of DR5 in HEK 293, MCF-7 and MDB-MB-231 epithelial cell lines through NF-kB activation, without an effect on DR4 expression. Blockage of NF-κB activation, either by expression of dominant negative I-κB or treatment with the proteasome inhibitor lactacystin, eliminates TRAIL-induced DR5 expression.17) Moreover, by overexpressing the p65 subunit of NF-κB, which increases NF-κB transcriptional activity, DR5 expression is increased compared to vector-only-expressing cells.17) Thus, TRAIL-mediated NF-κB activation increases DR5 expression, thereby amplifying the apoptotic response of TRAIL in epithelial derived cells.17)
Although we did not analyze the mechanisms through which expression of DR5 is increased, treatment with only TRAIL did not induce DR5 expression, while co-treatment with ISO and TRAIL increased DR5 expression in HEK 293 cells. Taken together, our results suggest that treatments combining ISO with TRAIL may be responsible for HEK 293 cell death through DR5 up-regulation. More detailed studies of cardiac cell death conditions, such as β-AR activation and inflammation in the heart, will be required to understand the pathogenesis of cardiac disease.
Figures and Tables
Fig. 1
Cell viability of HEK 293 cells treated with ISO and/or TRAIL. (A) Cell death induced by treatment with ISO and/or TRAIL after 24 hours. Cells were photographed (100X magnification) using a microscope. (B) Cytotoxicity caused by treatment with ISO and/or TRAIL after 24 hours. Cytotoxicity was measured by the MTT assay. Standard error was determined on the basis of three independent experiments. HEK: human embryonic kidney, ISO: isoproterenol, TRAIL: tumor necrosis factor-related apoptosis-inducing ligand, MTT: methylthiazolyldiphenyl-tetrazolium bromide.
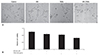
Fig. 2
Synergistic apoptotic cell death caused by treatment with ISO and/or TRAIL. HEK 293 cells were treated with ISO (100 µM) and/or TRAIL (100 ng/mL) for 24 hours. (A and B) Apoptotic cell death of HEK 293 cells treated with ISO and/or TRAIL. The percentage of apoptotic cells was determined by FITC-annexin-V/PI staining. (C) Caspase-3 activation by ISO and TRAIL treatment. Caspase-3 activation and PARP cleavage were observed in HEK 293 cells treated with ISO and TRAIL. (D) The band intensities of cleaved cas-3 and PARP were analyzed by densitometry. GAPDH was used as the housekeeping gene. Standard error was determined on the basis of three independent experiments; *p<0.05. ISO: isoproterenol, TRAIL: tumor necrosis factor-related apoptosis-inducing ligand, HEK: human embryonic kidney, FITC: fluorescein isothiocynate, PI: propidium iodide, PARP: polymerase, GAPDH: glyceraldehydes-3-phosphate dehydrogenase.
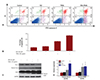
Fig. 3
Up-regulation of DR5 in HEK 293 cells treated with ISO and TRAIL. HEK 293 cells were treated with ISO (100 µM) and/or TRAIL (100 ng/mL) for 24 hours. DR5 expression was evaluated by immunoblotting, and the band intensity of DR5 was analyzed by densitometry. GAPDH was used as the housekeeping gene. Standard error was determined on the basis of three independent experiments; *p<0.05. DR: death receptor, HEK: human embryonic kidney, ISO: isoproterenol, TRAIL: tumor necrosis factor-related apoptosis-inducing ligand, GAPDH: glyceraldehydes-3-phosphate dehydrogenase.
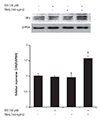
Acknowledgments
This study was supported by a grant from the Korean Society of Cardiology (201001-01).
References
1. Whelan RS, Kaplinskiy V, Kitsis RN. Cell death in the pathogenesis of heart disease: mechanisms and significance. Annu Rev Physiol. 2010; 72:19–44.
2. Zauli G, Secchiero P. The role of the TRAIL/TRAIL receptors system in hematopoiesis and endothelial cell biology. Cytokine Growth Factor Rev. 2006; 17:245–257.
3. Ashkenazi A, Dixit VM. Apoptosis control by death and decoy receptors. Curr Opin Cell Biol. 1999; 11:255–260.
4. Galligan L, Longley DB, McEwan M, Wilson TR, McLaughlin K, Johnston PG. Chemotherapy and TRAIL-mediated colon cancer cell death: the roles of p53, TRAIL receptors, and c-FLIP. Mol Cancer Ther. 2005; 4:2026–2036.
5. Kim Y, Seol DW. TRAIL, a mighty apoptosis inducer. Mol Cells. 2003; 15:283–293.
6. Ashkenazi A. Targeting death and decoy receptors of the tumour-necrosis factor superfamily. Nat Rev Cancer. 2002; 2:420–430.
7. Wilson NS, Dixit V, Ashkenazi A. Death receptor signal transducers: nodes of coordination in immune signaling networks. Nat Immunol. 2009; 10:348–355.
8. Yamaguchi H, Wang HG. CHOP is involved in endoplasmic reticulum stress-induced apoptosis by enhancing DR5 expression in human carcinoma cells. J Biol Chem. 2004; 279:45495–45502.
9. Naga Prasad SV, Nienaber J, Rockman HA. Beta-adrenergic axis and heart disease. Trends Genet. 2001; 17:S44–S49.
10. Engelhardt S, Hein L, Wiesmann F, Lohse MJ. Progressive hypertrophy and heart failure in beta1-adrenergic receptor transgenic mice. Proc Natl Acad Sci U S A. 1999; 96:7059–7064.
11. Houser SR, Margulies KB, Murphy AM, et al. Animal models of heart failure: a scientific statement from the American Heart Association. Circ Res. 2012; 111:131–150.
12. Communal C, Singh K, Pimentel DR, Colucci WS. Norepinephrine stimulates apoptosis in adult rat ventricular myocytes by activation of the beta-adrenergic pathway. Circulation. 1998; 98:1329–1334.
13. Zaugg M, Xu W, Lucchinetti E, Shafiq SA, Jamali NZ, Siddiqui MA. Beta-adrenergic receptor subtypes differentially affect apoptosis in adult rat ventricular myocytes. Circulation. 2000; 102:344–350.
14. Liu JJ, Li DL, Zhou J, et al. Acetylcholine prevents angiotensin IIinduced oxidative stress and apoptosis in H9c2 cells. Apoptosis. 2011; 16:94–103.
15. Groenning BA, Nilsson JC, Sondergaard L, Fritz-Hansen T, Larsson HB, Hildebrandt PR. Antiremodeling effects on the left ventricle during beta-blockade with metoprolol in the treatment of chronic heart failure. J Am Coll Cardiol. 2000; 36:2072–2080.
16. Park JB, Lee JS, Cho BP, et al. Adipose tissue-derived mesenchymal stem cells cultured at high cell density express brain-derived neurotrophic factor and exert neuroprotective effects in a 6-hydroxydopamine rat model of Parkinson's disease. Genes Genom. 2015; 37:213–221.
17. Shetty S, Gladden JB, Henson ES, et al. Tumor necrosis factor-related apoptosis inducing ligand (TRAIL) up-regulates death receptor 5 (DR5) mediated by NFkappaB activation in epithelial derived cell lines. Apoptosis. 2002; 7:413–420.
18. Fanger NA, Maliszewski CR, Schooley K, Griffith TS. Human dendritic cells mediate cellular apoptosis via tumor necrosis factor-related apoptosis-inducing ligand (TRAIL). J Exp Med. 1999; 190:1155–1164.
19. Griffith TS, Wiley SR, Kubin MZ, Sedger LM, Maliszewski CR, Fanger NA. Monocyte-mediated tumoricidal activity via the tumor necrosis factor-related cytokine, TRAIL. J Exp Med. 1999; 189:1343–1354.
20. Kemp TJ, Moore JM, Griffith TS. Human B cells express functional TRAIL/Apo-2 ligand after CpG-containing oligodeoxynucleotide stimulation. J Immunol. 2004; 173:892–899.
21. Zamai L, Ahmad M, Bennett IM, Azzoni L, Alnemri ES, Perussia B. Natural killer (NK) cell-mediated cytotoxicity: differential use of TRAIL and Fas ligand by immature and mature primary human NK cells. J Exp Med. 1998; 188:2375–2380.
22. Cassatella MA, Huber V, Calzetti F, et al. Interferon-activated neutrophils store a TNF-related apoptosis-inducing ligand (TRAIL/Apo-2 ligand) intracellular pool that is readily mobilizable following exposure to proinflammatory mediators. J Leukoc Biol. 2006; 79:123–132.
23. Koga Y, Matsuzaki A, Suminoe A, Hattori H, Hara T. Neutrophil-derived TNF-related apoptosis-inducing ligand (TRAIL): a novel mechanism of antitumor effect by neutrophils. Cancer Res. 2004; 64:1037–1043.
24. Kemp TJ, Ludwig AT, Earel JK, et al. Neutrophil stimulation with Mycobacterium bovis bacillus Calmette-Guerin (BCG) results in the release of functional soluble TRAIL/Apo-2L. Blood. 2005; 106:3474–3482.
25. Simons MP, Leidal KG, Nauseef WM, Griffith TS. TNF-related apoptosis-inducing ligand (TRAIL) is expressed throughout myeloid development, resulting in a broad distribution among neutrophil granules. J Leukoc Biol. 2008; 83:621–629.
26. Murray DR, Prabhu SD, Chandrasekar B. Chronic beta-adrenergic stimulation induces myocardial proinflammatory cytokine expression. Circulation. 2000; 101:2338–2341.
27. Liao X, Wang X, Gu Y, Chen Q, Chen LY. Involvement of death receptor signaling in mechanical stretch-induced cardiomyocyte apoptosis. Life Sci. 2005; 77:160–174.
28. Prasad S, Kim JH, Gupta SC, Aggarwal BB. Targeting death receptors for TRAIL by agents designed by Mother Nature. Trends Pharmacol Sci. 2014; 35:520–536.
29. Lee MW, Park SC, Kim JH, et al. The involvement of oxidative stress in tumor necrosis factor (TNF)-related apoptosis-inducing ligand (TRAIL)-induced apoptosis in HeLa cells. Cancer Lett. 2002; 182:75–82.
30. Chandel NS, Schumacker PT, Arch RH. Reactive oxygen species are downstream products of TRAF-mediated signal transduction. J Biol Chem. 2001; 276:42728–42736.