Abstract
Background and Objectives
Identifying the critical isthmus of slow conduction is crucial for successful treatment of scar-related ventricular tachycardia. Current 3D mapping is not designed for tracking the critical isthmus and may lead to a risk of extensive ablation. We edited the algorithm to track the delayed potential in order to visualize the isthmus and compared the edited map with a conventional map.
Subjects and Methods
We marked every point that showed delayed potential with blue color. After substrate mapping, we edited to reset the annotation from true ventricular potential to delayed potential and then changed the window of interest from the conventional zone (early, 50-60%; late, 40-50% from peak of QRS) to the edited zone (early, 80-90%; late, 10-20%) for every blue point. Finally, we compared the propagation maps before and after editing.
Results
We analyzed five scar-related ventricular tachycardia cases. In the propagation maps, the resetting map showed the critical isthmus and entrance and exit sites of tachycardia that showed figure 8 reentry. However, conventional maps only showed the earliest ventricular activation sites and searched for focal tachycardia. All of the tachycardia cases were terminated by ablating the area around the isthmus.
Catheter ablation of scar-related reentrant ventricular tachycardia (VT) is challenging, although it is necessary for managing patients with frequent implantable cardioverter-defibrillator (ICD) shock therapy.1)2) Identifying the critical isthmus of slow conduction is crucial for successfully treating scar-related VT.3)4)5) The current mapping strategy for characterizing the isthmus uses activation and entrainment mapping in reproducible and well-tolerated VT.4)6)7) Recently, in cases of hemodynamically unstable VT, various approaches such as substrate mapping, ablating the isolated late potentials, and eliminating local abnormal ventricular activities (LAVA) have been proposed.8)9)10)11) These substrate modifications have been attempted as endpoints for VT ablation, although these strategies were not specific and carry the risk of extensive ablation.12)13)14) In addition, substrate-based approaches require complicated steps including voltage mapping, characterizing a slow conduction zone by delayed potential mapping, and entrainment or pace mapping to define the VT circuit.10)11)15)
The aim of the present study was to identify the reentry circuit isthmus using a simple method based on substrate mapping and a new color-coded propagation mapping strategy using a specific method by resetting the window of interest.
Between March 2009 and February 2014, 13 consecutive patients who were undergoing catheter ablation for sustained, recurrent and drug-refractory VT with ischemic or non-ischemic cardiomyopathy (CMP) were reviewed. For the purpose of detailed activation mapping, the five patients (age 60.6±10.5 years, male 100%) with reproducible and hemodynamically tolerated monomorphic VT were included.
Detailed electroanatomic data were obtained from all of the patients using either the CARTO system (Biosense-Webster Inc., Diamond Bar, CA, USA) or the CARTO-RMT™ integration system (Stereotaxis Inc., St. Louis, MO, USA). Mapping and ablation were performed using 8 Fr catheters with a conventional 3.5 mm tip (NaviStar™ RMT; Biosense-Webster Inc., Diamond Bar, CA, USA) or a 3.5 mm irrigated tip electrode (NaviStar ThermoCool™, Biosense-Webster Ltd, Diamond Bar, CA, USA). All studies were done under local anesthesia and deep sedation after a preprocedural transthoracic or transesophageal echocardiogram to exclude the presence of intracardiac thrombus.
VT was induced by programmed stimulation, and substrate maps of the left ventricle were created initially. Before the procedure, we carefully analyzed the documented twelve lead electrocardiogram (ECG) and ICD electrograms and determined the characteristics of spontaneously induced clinical VT. We then selected the target VTs for ablation that would repeatedly lead to ICD shock. Clinical VT was defined as VT that was induced spontaneously or that showed the same axis and similar cycle lengths compared with the clinically documented ECG or ICD electrograms (Fig. 1). The voltage map was used in bipolar mode for identifying scar areas. The default color scale of the border zone was set from 0.5 mV (red) to 1.5 mV (purple), and the maps that had a voltage of <0.5 mV were considered scars and displayed in grey. All areas of the border zone and scar region were analyzed. Then, activation mapping was performed during the tachycardia. All sites with isolated delayed potentials, indicative of conduction block, were tagged with blue dots. Isolated delayed potentials were defined as low-amplitude, high-frequency potentials that spanned the diastolic period and separated from the ventricular electrograms.16) High-density fine mapping was performed, and only consistent potentials were tagged that facilitated differentiation from the far field potentials.
After the initial mapping, a complete activation and propagation map during VT was performed, and the critical isthmus was identified. To better identify the direction of reentry, the propagation map was carefully re-analyzed. The annotation was moved from the true ventricular activation potential to the beginning of the local isolated diastolic potential. Then, the onset of the window of interest was reset to the diastolic phase by changing the ratio from the conventional zone (early, 50-60%; late, 40-50%) to the adjusted zone (early, 80-90%; late, 10-20%) (Fig. 2). The duration of the window of interest corresponds to the interval of the arrhythmia cycle length (CL) that spans 95% of the CL. Finally, we conducted entrainment mapping to confirm the entrance, exit or bystander sites.
After the analysis of the maps, either focal or linear ablation was performed targeting the exit site of the critical isthmus and the isolated delayed potentials. The endpoint was the non-inducibility of the clinical VT. All patients were followed every 3-6 months by ICD programming to assess the recurrence of VT.
Four patients (80%) had ischemic CMP, and one (20%) had non-ischemic CMP. All patients had implanted ICDs, and two were documented as VT only by ICD. The mean LV ejection fraction was 27.1±9.6%. Four patients had frequent episodes of ICD therapy, one had electrical storm and all had single clinical VT morphology with variable cycle lengths. Other clinical characteristics are shown in Table 1.
All of the mapping was obtained during VT. A total of 15 VTs were induced, and three of them were hemodynamically unstable. The mean number of points was 266±50 in the complete maps, and mean substrate volume was 164±59 cm3. By resetting the ratio of the window of interest from 6:4 to 9:1, we could trace the delayed potentials that spanned the entire diastolic phase. Fig. 3 shows the presence of delayed potentials close to the border and scar areas, and the activation sequence of the delayed potentials represents the direction of ongoing VT. Before we edited the propagation maps, they showed focal tachycardia patterns and could not identify the reentrant circuit. The edited activation and propagation maps were able to identify the channel and the direction of the reentrant circuits that showed figure 8 reentry (Figs. 4 and 5). In Fig. 4, the activation map showed the area where the early zone met the late zone. In addition, delayed potentials were observed close to the scar border zones on the voltage maps. The clinical VT terminated during isthmus ablation, and after final ablation, clinical tachycardia was not induced in any patients.
The mean follow-up duration was 17.6±12.5 months. Of the five patients, two died. One died during the hospitalization course, and the other had VT recurrence after one month and died ten months after VT ablation. Another patient had VT recurrence three months after ablation. The remaining two patients had successful ablation and no ventricular arrhythmia events for 31 months and 25 months.
This study has important clinical impacts regarding how this mapping technique was demonstrated to be effective in reconstructing the whole VT reentrant circuit using a simple method. Different mapping and ablation strategies have been reported for ablating scar-related VT. Conventional electrophysiologic methods using entrainment or pace mapping with electroanatomic activation mapping can be used to identify the isthmus. Recently, the presence of isolated late potentials or LAVA and eliminating these potentials were suggested for detailed mapping, although these approaches are complex and involve a number of steps. Based on this, we proposed a simple method for characterizing the critical isthmus using electroantomic activation mapping guided by delayed potential.
Classic entrainment mapping was a useful technique, but it is difficult to maintain VT during pacing, and different VTs can be induced during entrainment.6) Recently, three-dimensional electroanatomical mapping techniques have been suggested to reconstruct scar-related VT circuits.7)10)14) Scar zones can be characterized by electroanatomical mapping.17) However, current 3D mapping is not designed for tracking the critical isthmus; more than one channel can be observed, and the majority of them are bystanders. In addition, it is difficult to locate the ablation target, and empiric ablation can cause extensive ablation.18) For more detailed and specific mapping, Mountantonakis et al.11) suggest that voltage channels that contain isolated late potentials can increase the specificity of predicting the clinical channel. However, these channels did not include the critical isthmus in some cases. In the present investigation, we also could not identify the critical isthmus by voltage map, and it was then useful to merge the voltage map with the edited delayed potential map to visualize the direction of the reentrant circuit. We reset the window of interest to the diastolic phase by changing the ratio to 9:1, which enabled coverage of the whole diastolic phase. The reset ratio was chosen to include the delayed potentials in the window of interest. Delayed potential represents the local slow conduction of the surviving myocardium,19) which showed along the scar border zone. These heterogeneous scar border areas are critical lesions for reentry.20) Therefore, the activation sequence of the delayed potentials represents the propagation of the critical isthmus. Using this method, we were able to locate the clinical channel and make it the ablation target.
Various VTs could be induced during or even after the ablation procedure.21) However, our purpose in ablating was to reduce ICD shock or electrical storm. Therefore, the ablation target was only clinical VT that induced spontaneously and caused repeated ICD shock. Even when we ablated only the target VTs, we never saw the various kinds of VTs that were induced in the EP lab in the EGM analysis after ablation, and we could prevent frequent episodes of ICD shock.
Furthermore, most of our cases used the CARTO-RMT™ integration system, which facilitates more mapping points and can overcome the difficult access by achieving optimal catheter position and contact, making for more detailed and effective electroanatomical mapping.
In patients with non-inducible or non-tolerated VT, we could not apply the new mapping strategy. Therefore, this study had a limited number of patients in whom to propose the new method. Moreover, it is not possible to prove the long-term efficacy of ablation in these patients.
In addition, we conducted the entrainment after final mapping, although in some cases, we could not carry out the entrainment at all delayed potential sites because of the irreproducibility of clinical VT. These cases were identified as non-inducible after ablation wether the critical isthmus was ablated. In this sense, the new method for identifying the channel can easily overcome the limitation of entrainment.
Figures and Tables
Fig. 1
12-lead ECG of clinical VT and that of VT that was induced and targeted during the induction study. Nonclinical VT showed different axis and cycle lengths. VTs that were documented in the ICD electrograms showed similar cycle lengths and could not be terminated by anti-tachycardial pacing. ECG: electrocardiogram, VT: ventricular tachycardia, ICD: implantable cardioverter-defibrillator.
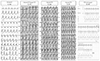
Fig. 2
Editing the annotation and the window of interest. (A) In conventional mapping, annotation was set to the maximum ventricular potential (arrow), and the window of interest was set to 5:5. (B) After we reset the conventional map, annotation was set to the local delayed potential (arrow), and the window of interest was reset to 9:1.
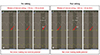
Fig. 3
Voltage map showing the locations of delayed potentials (AP view). Blue dots denote delayed potentials, which are noted along the scar border zone. Tracing of the diastolic potentials (black arrow) and entrainment of the exit and bystander sites were shown. Entrainment showed concealed fusion. AP: anterior-posterior, S-QRS: stim to QRS, PPI: post-pacing interval, VTCL: ventricular tachycardia cycle length.
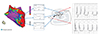
Fig. 4
Activation map before (A) and after (B) editing. Voltage map showing the locations of the delayed potentials (C). Delayed potentials are tagged with blue dots, and scar sites are tagged with grey dots. After editing, the activation map shows the area where the early zone meets the late zone, which represents the reentry mechanism.
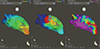
Fig. 5
Propagation map before (A) and after (B) editing. Delayed potentials are tagged with blue dots, and scar sites are tagged with grey dots. The white arrow shows the direction of propagation. (A) from apex to base; (B) figure 8 reentry through the isthmus.
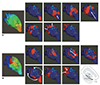
Table 1
Baseline characteristics
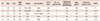
References
1. Pedersen CT, Kay GN, Kalman J, et al. EHRA/HRS/APHRS expert consensus on ventricular arrhythmias. Heart Rhythm. 2014; 11:e166–e196.
2. Kuck KH. Should catheter ablation be the preferred therapy for reducing ICD shocks?: ventricular tachycardia in patients with an implantable defibrillator warrants catheter ablation. Circ Arrhythm Electrophysiol. 2009; 2:713–720.
3. Morady F, Frank R, Kou WH, et al. Identification and catheter ablation of a zone of slow conduction in the reentrant circuit of ventricular tachycardia in humans. J Am Coll Cardiol. 1988; 11:775–782.
4. Stevenson WG, Khan H, Sager P, et al. Identification of reentry circuit sites during catheter mapping and radiofrequency ablation of ventricular tachycardia late after myocardial infarction. Circulation. 1993; 88(4 Pt 1):1647–1670.
5. de Chillou C, Magnin-Poull I, Andronache M, et al. Showing up channels for postinfarct ventricular tachycardia ablation. Pacing Clin Electrophysiol. 2012; 35:897–904.
6. Stevenson WG, Friedman PL, Sager PT, et al. Exploring postinfarction reentrant ventricular tachycardia with entrainment mapping. J Am Coll Cardiol. 1997; 29:1180–1189.
7. de Chillou C, Lacroix D, Klug D, et al. Isthmus characteristics of reentrant ventricular tachycardia after myocardial infarction. Circulation. 2002; 105:726–731.
8. Arenal A, Glez-Torrecilla E, Ortiz M, et al. Ablation of electrograms with an isolated, delayed component as treatment of unmappable monomorphic ventricular tachycardias in patients with structural heart disease. J Am Coll Cardiol. 2003; 41:81–92.
9. Jaïs P, Maury P, Khairy P, et al. Elimination of local abnormal ventricular activities: a new end point for substrate modification in patients with scar-related ventricular tachycardia. Circulation. 2012; 125:2184–2196.
10. Vergara P, Trevisi N, Ricco A, et al. Late potentials abolition as an additional technique for reduction of arrhythmia recurrence in scar related ventricular tachycardia ablation. J Cardiovasc Electrophysiol. 2012; 23:621–627.
11. Mountantonakis SE, Park RE, Frankel DS, et al. Relationship between voltage map "channels" and the location of critical isthmus sites in patients with post-infarction cardiomyopathy and ventricular tachycardia. J Am Coll Cardiol. 2013; 61:2088–2095.
12. Verma A, Marrouche NF, Schweikert RA, et al. Relationship between successful ablation sites and the scar border zone defined by substrate mapping for ventricular tachycardia post-myocardial infarction. J Cardiovasc Electrophysiol. 2005; 16:465–471.
13. Haqqani HM, Marchlinski FE. Electrophysiologic substrate underlying postinfarction ventricular tachycardia: characterization and role in catheter ablation. Heart Rhythm. 2009; 6:8 Suppl. S70–S76.
14. Vergara P, Roque C, Oloriz T, Mazzone P, Della Bella P. Substrate mapping strategies for successful ablation of ventricular tachycardia: a review. Arch Cardiol Mex. 2013; 83:104–111.
15. de Chillou C, Groben L, Magnin-Poull I, et al. Localizing the critical isthmus of postinfarct ventricular tachycardia: the value of pace-mapping during sinus rhythm. Heart Rhythm. 2014; 11:175–181.
16. Issa ZF, Miller JM, Zipes DP. Clinical Arrhythmology and Electrophysiology : A Companion to Braunwald's Heart Disease. 2nd ed. Philadelphia, PA: Elseiver/Saunders;2012. p. 537.
17. Fahmy TS, Wazni OM, Jaber WA, et al. Integration of positron emission tomography/computed tomography with electroanatomical mapping: a novel approach for ablation of scar-related ventricular tachycardia. Heart Rhythm. 2008; 5:1538–1545.
18. Di Biase L, Santangeli P, Burkhardt DJ, et al. Endo-epicardial homogenization of the scar versus limited substrate ablation for the treatment of electrical storms in patients with ischemic cardiomyopathy. J Am Coll Cardiol. 2012; 60:132–141.
19. de Bakker JM, Wittkampf FH. The pathophysiologic basis of fractionated and complex electrograms and the impact of recording techniques on their detection and interpretation. Circ Arrhythm Electrophysiol. 2010; 3:204–213.
20. Nakahara S, Tung R, Ramirez RJ, et al. Distribution of late potentials within infarct scars assessed by ultra high-density mapping. Heart Rhythm. 2010; 7:1817–1824.
21. Yokokawa M, Desjardins B, Crawford T, Good E, Morady F, Bogun F. Reasons for recurrent ventricular tachycardia after catheter ablation of post-infarction ventricular tachycardia. J Am Coll Cardiol. 2013; 61:66–73.