Abstract
Hepatitis B virus (HBV) infection remains a major global health problem; indeed, there are 250 million carriers worldwide. The host range of HBV is narrow; therefore, few primates are susceptible to HBV infection. However, ethical constraints, high cost, and large size limit the use of primates as suitable animal models. Thus, in vivo testing of therapies that target HBV has been hampered by the lack of an appropriate in vivo research model. To address this, mouse model systems of HBV are being developed and several are used for studying HBV in vivo. In this review, we summarize the currently available mouse models, including HBV transgenic mice, hydrodynamic injection-mediated HBV replicon delivery systems, adeno-associated virus-mediated HBV replicon delivery systems, and human liver chimeric mouse models. These developed (or being developed) mouse model systems are promising and should be useful tools for studying HBV.
More than 250 million people worldwide carry hepatitis B virus (HBV). These people are prone to developing liver failure and diseases such as cirrhosis and hepatocellular carcinoma. Although vaccination and anti-HBV therapies are available, HBV infection remains a major global health concern because clearing the virus from chronic HBV carriers is difficult using current treatment methods.
HBV is a DNA virus with reverse transcriptional activity, belonging to the same family (Hepadnaviridae) as woodchuck hepatitis virus and duck hepatitis B virus (Figure 1). The infectious HBV virion, also known as the Dane particle, has a globular structure (approximately 42 nm in diameter) that contains the nucleocapsid [12]. The HBV capsid comprises 120 copies of core protein dimers [34] enclosed within an envelope, which consists of a lipid bilayer membrane derived from the host cell. Embedded in this bilayer are three viral surface proteins (small surface protein [SHB], middle surface protein [MHB], and large surface protein [LHB]). The nucleocapsid harbors a partially double-stranded HBV genome (approximately 3.2 kB) known as relaxed-circular DNA (rcDNA) and the viral polymerase (P protein), which is cross-linked covalently to the rcDNA [56].
A major cause of chronic HBV infection is covalently closed circular DNA (cccDNA), which forms a minichromosome in the host cell nucleus and serves as a template for viral transcription by host RNA polymerase [7]. Therefore, strategies targeting cccDNA may form the basis for developing novel antiviral agents. Recent studies show that interferon-α or APOBEC3 proteins reduce the amount of cccDNA [89]; however, this is not sufficient for complete elimination of cccDNA from host hepatocytes.
HBV infection and development of hepatitis are complex responses involving many factors. Thus, limited investigations have been undertaken using in vitro systems. The host range of HBV is narrow; therefore, few primates are susceptible to HBV infection. Among these, only chimpanzees are immunocompetent and fully susceptible to human HBV [1011]. They develop both acute and chronic HBV infection, along with hepatitis and immune response that are similar to those in humans [12]. However, ethical constraints, large size, and high cost make chimpanzees unsuitable animal models. Tupaias can be infected with HBV, but infection is mild and transient, and viral replication is limited [13]. In addition, these animals are large and hard to handle. Thus, small, easy to handle, and well-defined animal models such as mice are required. Several mouse models of HBV have been established, and novel mouse models that enable global assessment of the viral infection process and efficacy of antiviral agents are being developed continuously.
The HBV virion attaches to hepatocytes via weak interaction between the preS1 domain of the LHB protein on the HBV envelope and heparin sulfate proteoglycans (HSPGs) on the surface of hepatocytes [14]. The sodium taurocholate co-transporting polypeptide (NTCP) on the host cell surface, which is a receptor for HBV entry [15], allows the HBV virion to pass through the plasma membrane via clathrin-mediated endocytosis [16]. However, the detailed molecular mechanisms underlying the entry process are still not fully understood.
After endocytosis, the nucleocapsid is released from the viral envelope and transported to the nucleus. The underlying process is unclear; however, one possible mechanism is that the arginine-rich C-terminal domain of the core protein may provide a transport signal to a nuclear pore. Also, the nucleocapsid is degraded at the nuclear pore complex, whereupon it releases the rcDNA, which then enters the nucleus [17].
In the nucleus, rcDNA is transcribed to cccDNA. First, HBV polymerase is released from the 5' terminus of the minus rcDNA strand and the RNA primer is removed from the 5' terminus of the plus strand. Host DNA polymerase κ (POLK) and other DNA polymerases fill the gap in the plus strand [18]. The host DNA repair machinery, such as tyrosyl-DNA phosphodiesterase 2 (TDP2), plays a role in this process [19], although other as-yet-unknown host cellular factors are also involved. The cccDNA is maintained in the nucleus of host hepatocytes as a stable episome, which forms a minichromosome by associating with histones and nonhistone proteins [7].
The cccDNA serves as a template for transcription of viral mRNAs by host cellular RNA polymerase 2 [2021]. There are four different HBV mRNAs, which differ in length. The longest mRNA, a 3.5 kB pregenomic RNA (pgRNA), encodes the polymerase protein and the precore/core protein. A 2.4 kB preS mRNA encodes the LHB and MHB proteins, and a 2.1 kB S mRNA encodes the SHB protein. In addition, a 0.7 kB X mRNA encodes the X protein. Transcription of these four transcripts initiates at distinct promoters, but all have overlapping 3' termini. Four promoters and two enhancers play roles in transcription: the core, PreS, S, and X promoters, and enhancers 1 and 2. All four transcripts have 5' cap structures and 3' poly-A tails.
pgRNA serves as a precursor for synthesis of HBV genomic DNA. Encapsidation is triggered by binding of HBV polymerase to the ε-stem loop structure of the pgRNA 5' terminal region in the cytoplasm [222324]. Because pgRNA is terminally redundant, the stem loop structure is also present at the 3' terminus; however, the polymerase also recognizes the 5' cap structure [25]. Therefore, only the 5' ε-stem loop can serve as an encapsidation signal. After binding the polymerase protein, core proteins are recruited for capsid assembly, and pgRNA and polymerase are encapsidated. Next, using pgRNA as a template, the minus strand of rcDNA is synthesized by the reverse transcriptase activity of HBV polymerase [26]. The N-terminal TP domain of HBV polymerase has a tyrosine (Y63) residue that acts as a protein primer for polymerization of minus-strand DNA [627]. After this step, most of the pgRNA template is degraded by the RNase H domain of HBV polymerase, leaving only a small fragment of RNA. The remaining RNA fragment is used as a primer for synthesis of plusstrand DNA [28], resulting in rcDNA synthesis in the nucleocapsid.
The nucleocapsid moves to the endoplasmic reticulum (ER), where the surface proteins (SHB, MHB, and LHB) are embedded into the membrane [2930]. Enveloped HBV (the Dane particle) is formed in the lumen of the ER and released from the cell through secretory pathways and budding. In addition, two kinds of subviral particle, spherical and filamentous, are secreted from the cell. These do not have a viral nucleocapsid and comprise the viral envelope itself.
Initially, transgenic mice expressing each viral protein were constructed [3132333435]. These mice were used to evaluate the virology and oncogenic potential of each viral protein. Thereafter, transgenic mice expressing the complete HBV replicon were generated; this model produces infectious virions [36] and made it possible to test the efficacy of antiviral agents such as HBV inhibitors, small interfering RNAs (siRNAs), and cytokines in vivo [37383940]. Since HBV transgenic mice are immune tolerant and HBV pathogenesis proceeds via host adaptive immune responses, the mice do not suffer hepatitis/liver injury or clear the virus. This means that HBV viral proteins or HBV itself is not cytopathic; in addition, HBV-specific cytotoxic T lymphocytes (CTLs) cause acute liver disease when adoptively transferred to these HBV transgenic mice [41]. Thus, studies examining the mechanism(s) underlying infection or cccDNA synthesis are not suited to this model. Since the murine hepatocytes do not express HBV-specific receptors, making intrahepatic infection or spread of HBV impossible, no cccDNA is formed within murine hepatocytes. The inability to clear HBV means that alternative immunocompetent mouse models are required.
This mouse model is based on immunocompetent mice; therefore, pathogenesis and host immune responses against HBV can be observed [4243]. The HBV replicons are transferred to the mouse liver by injecting a large volume of naked DNA into the tail vein. This DNA is transiently expressed by hepatocytes. Viral replication remains at high levels until Day 7 post-transfection, at which time it starts to decline as HBV-specific antiviral antibodies and CTLs begin to emerge; these are key effectors for viral clearance, which is supported by CD4+ T cells and natural killer cells [42]. This mouse model is used to test antiviral agents such as short hairpin RNAs (shRNAs) and HBV genomespecific guide RNA (gRNA)-mediated clustered regularly interspaced short palindromic repeats (CRISPR)/Cas9 systems [4445]. Recently, the model was used for research into recombinant cccDNA (rcccDNA), which is derived from hydrodynamic-injected HBV minicircle DNA and mimics cccDNA [46].
This model, which is based on viral transduction by intravenously injected adeno-associated virus (AAV), allows more efficient and homogeneous transduction of the liver than the hydrodynamic injection model [4247], in which only 5–10% of hepatocytes are transfected; indeed, more than half of murine hepatocytes in the AAV model express HBcAg [47]. In contrast to the hydrodynamic injection model, this model mimics chronic HBV infection [47]. AAV infection does not induce some types of immune response, and the murine immune system is tolerant to HBV antigens; therefore, HBV persistence is established in injected immunocompetent mice. Due to immune tolerance to HBV, this mouse model can be used to develop anti-HBV vaccines [4748].
Since mice are not a natural host for HBV, it is not possible to study the entire infection process (from viral entry to cccDNA synthesis and intrahepatic spread) in transgenic or HBV replicon-delivered mice. Therefore, a human liver chimeric mouse model is required.
The first such model was the trimera mouse model, which was established by transplanting HBV-infected human liver fragments under the kidney capsule of lethally irradiated immunodeficient mice [49]. This mouse model has been used to test several antiviral agents; however, since transplanted xenogeneic human hepatocytes do not remain intact for a long time, other models have been developed in which human liver cells are stably engrafted.
The most widely used human liver chimeric mouse model is the albumin-urokinase-type plasminogen activator (alb-uPA)/severe combined immunodeficiency (SCID) mouse [50]. When driven by a liver-specific albumin promoter, uPA is overexpressed and results in subacute liver failure [51]. After backcrossing alb-uPA mice with immunodeficient mice (e.g., SCID or recombination activating gene 2 [RAG2] knockout mice), human hepatocytes are introduced via intra-splenic injection. Next, the small numbers of hepatocytes that reach the injured liver proliferate and repopulate it. The engrafted human hepatocytes are then maintained stably [52]. However, this mouse model has several drawbacks, including infertility, potential fatal bleeding, and kidney disorders [5354]. An alternative human liver chimeric mouse model is the triple knockout FAH-/-RAG2-/- IL2RG-/- (FRG) mouse [55]. This model is generated by crossing fumarylacetoacetate hydrolase (FAH) knockout mice with double immunodeficient RAG2-/-interleukin 2 receptor γ chain (IL2RG)-/- mice. FAH is an enzyme essential for tyrosine metabolism; knocking out FAH leads to accumulation of fumarylacetoacetate in hepatocytes. This compound is toxic to hepatocytes and causes injury [56]. Accumulation of toxic metabolites can be prevented by administration of 2-(2-nitro-4-trifluoro-methylbenzoyl)-1,3-cyclohexanedione (NTBC) prior to transplantation. NTBC is withdrawn upon intra-splenic injection of human hepatocytes. The injected human hepatocytes then repopulate the mouse liver and persist over a period of 6 months [57]. Because human liver chimeric mice are susceptible to HBV infection and cccDNA is formed in transplanted hepatocytes, these models can be used to study viral infection, spread, and the nature of cccDNA. However, immunodeficiency makes these mice unsuitable for study of immune responses induced by HBV or vaccine development.
Here, we discuss the current knowledge base regarding the HBV life cycle and available mouse models (Figure 2 and Table 1). Each step of HBV infection can be targeted by compounds such as mycludex B (an entry inhibitor), APOBEC3 protein or CRISPR/Cas9 (cccDNA inhibitors), siRNAs (viral mRNA inhibitors), entecavir or tenofovir (reverse transcription inhibitors), and BAY 41-4109 (a core protein assembly inhibitor) [9373840444558596061]. However, complete elimination of cccDNA, a key treatment for chronic hepatitis B patients, has not yet been achieved. Development of transgenic and hydrodynamic injection or AAV transduction-mediated replicon delivery model mice allows elucidation of the mechanism(s) underlying the viral life cycle. Also, human liver chimeric mouse models allow examination of viral infection pathways, cccDNA formation, and their sequelae. However, these human liver chimeric mice are immunodeficient. Therefore, to overcome this limitation, immunocompetent human liver chimeric mouse models equipped with human livers and human immune systems have been developed. Examples include albumin promoter-driven-FK506-binding protein-caspase 8 fusion protein (AFC8)-CD34+ human hematopoietic stem cell (hu HSC)/hepatocyte progenitor (Hep) mice and HLA-A2 (A2)/non-obese diabetic SCID IL2RG-/- (NSG)-hu HSC/Hep mice [6263]. However, these mice do not harbor a complete human immune system. Studies of immunopathogenesis, development of novel vaccines, and more comprehensive analyses are feasible if robust dual humanized chimeric mouse models can be generated. This will be one of the most important contributions to HBV research.
Figures and Tables
Table 1
Comparison of the different mouse models
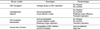
Acknowledgments
This work was supported by the Ministry of Health & Welfare, Republic of Korea (grant number: HI16C1074), and by the Basic Science Research Program through the National Research Foundation of Korea (NRF), funded by the Ministry of Education (grant number: NRF-2017R1E1A1A01074299).
References
1. Ryu W-S. Molecular virology of human pathogenic viruses. Amsterdam: Academic Press;2017. p. 247–260.
2. Dane DS, Cameron CH, Briggs M. Virus-like particles in serum of patients with Australia-antigen-associated hepatitis. Lancet. 1970; 1(7649):695–698.


3. Böttcher B, Wynne SA, Crowther RA. Determination of the fold of the core protein of hepatitis B virus by electron cryomicroscopy. Nature. 1997; 386(6620):88–91.


4. Crowther RA, Kiselev NA, Böttcher B, Berriman JA, Borisova GP, Ose V, Pumpens P. Three-dimensional structure of hepatitis B virus core particles determined by electron cryomicroscopy. Cell. 1994; 77(6):943–950.


5. Gerlich WH, Robinson WS. Hepatitis B virus contains protein attached to the 5' terminus of its complete DNA strand. Cell. 1980; 21(3):801–809.


6. Bartenschlager R, Schaller H. The amino-terminal domain of the hepadnaviral P-gene encodes the terminal protein (genome-linked protein) believed to prime reverse transcription. EMBO J. 1988; 7(13):4185–4192.


7. Bock CT, Schwinn S, Locarnini S, Fyfe J, Manns MP, Trautwein C, Zentgraf H. Structural organization of the hepatitis B virus minichromosome. J Mol Biol. 2001; 307(1):183–196.


8. Belloni L, Allweiss L, Guerrieri F, Pediconi N, Volz T, Pollicino T, Petersen J, Raimondo G, Dandri M, Levrero M. IFN-alpha inhibits HBV transcription and replication in cell culture and in humanized mice by targeting the epigenetic regulation of the nuclear cccDNA minichromosome. J Clin Invest. 2012; 122(2):529–537.
9. Lucifora J, Xia Y, Reisinger F, Zhang K, Stadler D, Cheng X, Sprinzl MF, Koppensteiner H, Makowska Z, Volz T, Remouchamps C, Chou WM, Thasler WE, Huser N, Durantel D, Liang TJ, Munk C, Heim MH, Browning JL, Dejardin E, Dandri M, Schindler M, Heikenwalder M, Protzer U. Specific and nonhepatotoxic degradation of nuclear hepatitis B virus cccDNA. Science. 2014; 343(6176):1221–1228.


10. Barker LF, Chisari FV, McGrath PP, Dalgard DW, Kirschstein RL, Almeida JD, Edington TS, Sharp DG, Peterson MR. Transmission of type B viral hepatitis to chimpanzees. J Infect Dis. 1973; 127(6):648–662.


11. Maynard JE, Berquist KR, Krushak DH, Purcell RH. Experimental infection of chimpanzees with the virus of hepatitis B. Nature. 1972; 237(5357):514–515.


12. Wieland SF. The chimpanzee model for hepatitis B virus infection. Cold Spring Harb Perspect Med. 2015; 5(6):pii: a021469.
13. Walter E, Keist R, Niederost B, Pult I, Blum HE. Hepatitis B virus infection of tupaia hepatocytes in vitro and in vivo. Hepatology. 1996; 24(1):1–5.


14. Schulze A, Gripon P, Urban S. Hepatitis B virus infection initiates with a large surface protein-dependent binding to heparan sulfate proteoglycans. Hepatology. 2007; 46(6):1759–1768.


15. Yan H, Zhong G, Xu G, He W, Jing Z, Gao Z, Huang Y, Qi Y, Peng B, Wang H, Fu L, Song M, Chen P, Gao W, Ren B, Sun Y, Cai T, Feng X, Sui J, Li W. Sodium taurocholate cotransporting polypeptide is a functional receptor for human hepatitis B and D virus. Elife. 2012; 1:e00049.


16. Huang HC, Chen CC, Chang WC, Tao MH, Huang C. Entry of hepatitis B virus into immortalized human primary hepatocytes by clathrin-dependent endocytosis. J Virol. 2012; 86(17):9443–9453.


17. Schmitz A, Schwarz A, Foss M, Zhou L, Rabe B, Hoellenriegel J, Stoeber M, Panté N, Kann M. Nucleoporin 153 arrests the nuclear import of hepatitis B virus capsids in the nuclear basket. PLoS Pathog. 2010; 6(1):e1000741.


18. Qi Y, Gao Z, Xu G, Peng B, Liu C, Yan H, Yao Q, Sun G, Liu Y, Tang D, Song Z, He W, Sun Y, Guo JT, Li W. DNA Polymerase kappa Is a Key Cellular Factor for the Formation of Covalently Closed Circular DNA of Hepatitis B Virus. PLoS Pathog. 2016; 12(10):e1005893.
19. Königer C, Wingert I, Marsmann M, Rösler C, Beck J, Nassal M. Involvement of the host DNA-repair enzyme TDP2 in formation of the covalently closed circular DNA persistence reservoir of hepatitis B viruses. Proc Natl Acad Sci U S A. 2014; 111(40):E4244–E4253.


20. Tuttleman JS, Pourcel C, Summers J. Formation of the pool of covalently closed circular viral DNA in hepadnavirus-infected cells. Cell. 1986; 47(3):451–460.


21. Rall LB, Standring DN, Laub O, Rutter WJ. Transcription of hepatitis B virus by RNA polymerase II. Mol Cell Biol. 1983; 3(10):1766–1773.


22. Nassal M, Rieger A. A bulged region of the hepatitis B virus RNA encapsidation signal contains the replication origin for discontinuous first-strand DNA synthesis. J Virol. 1996; 70(5):2764–2773.


23. Tavis JE, Perri S, Ganem D. Hepadnavirus reverse transcription initiates within the stem-loop of the RNA packaging signal and employs a novel strand transfer. J Virol. 1994; 68(6):3536–3543.


24. Wang GH, Seeger C. Novel mechanism for reverse transcription in hepatitis B viruses. J Virol. 1993; 67(11):6507–6512.


25. Jeong JK, Yoon GS, Ryu WS. Evidence that the 5'-end cap structure is essential for encapsidation of hepatitis B virus pregenomic RNA. J Virol. 2000; 74(12):5502–5508.


26. Summers J, Mason WS. Replication of the genome of a hepatitis B--like virus by reverse transcription of an RNA intermediate. Cell. 1982; 29(2):403–415.


27. Zoulim F, Seeger C. Reverse transcription in hepatitis B viruses is primed by a tyrosine residue of the polymerase. J Virol. 1994; 68(1):6–13.


28. Haines KM, Loeb DD. The sequence of the RNA primer and the DNA template influence the initiation of plus-strand DNA synthesis in hepatitis B virus. J Mol Biol. 2007; 370(3):471–480.


29. Eble BE, Lingappa VR, Ganem D. Hepatitis B surface antigen: an unusual secreted protein initially synthesized as a transmembrane polypeptide. Mol Cell Biol. 1986; 6(5):1454–1463.


30. Eble BE, Lingappa VR, Ganem D. The N-terminal (pre-S2) domain of a hepatitis B virus surface glycoprotein is translocated across membranes by downstream signal sequences. J Virol. 1990; 64(3):1414–1419.


31. Kim CM, Koike K, Saito I, Miyamura T, Jay G. HBx gene of hepatitis B virus induces liver cancer in transgenic mice. Nature. 1991; 351(6324):317–320.


32. Milich DR, Jones JE, Hughes JL, Price J, Raney AK, McLachlan A. Is a function of the secreted hepatitis B e antigen to induce immunologic tolerance in utero? Proc Natl Acad Sci U S A. 1990; 87(17):6599–6603.


33. Milich DR, Jones JE, Hughes JL, Maruyama T, Price J, Melhado I, Jirik F. Extrathymic expression of the intracellular hepatitis B core antigen results in T cell tolerance in transgenic mice. J Immunol. 1994; 152(2):455–466.
34. Chisari FV, Filippi P, McLachlan A, Milich DR, Riggs M, Lee S, Palmiter RD, Pinkert CA, Brinster RL. Expression of hepatitis B virus large envelope polypeptide inhibits hepatitis B surface antigen secretion in transgenic mice. J Virol. 1986; 60(3):880–887.


35. Chisari FV, Pinkert CA, Milich DR, Filippi P, McLachlan A, Palmiter RD, Brinster RL. A transgenic mouse model of the chronic hepatitis B surface antigen carrier state. Science. 1985; 230(4730):1157–1160.


36. Guidotti LG, Matzke B, Schaller H, Chisari FV. High-level hepatitis B virus replication in transgenic mice. J Virol. 1995; 69(10):6158–6169.


37. Weber O, Schlemmer KH, Hartmann E, Hagelschuer I, Paessens A, Graef E, Deres K, Goldmann S, Niewoehner U, Stoltefuss J, Haebich D, Ruebsamen-Waigmann H, Wohlfeil S. Inhibition of human hepatitis B virus (HBV) by a novel non-nucleosidic compound in a transgenic mouse model. Antiviral Res. 2002; 54(2):69–78.


38. Klein C, Bock CT, Wedemeyer H, Wustefeld T, Locarnini S, Dienes HP, Kubicka S, Manns MP, Trautwein C. Inhibition of hepatitis B virus replication in vivo by nucleoside analogues and siRNA. Gastroenterology. 2003; 125(1):9–18.


39. Wieland SF, Guidotti LG, Chisari FV. Intrahepatic induction of alpha/beta interferon eliminates viral RNA-containing capsids in hepatitis B virus transgenic mice. J Virol. 2000; 74(9):4165–4173.


40. Julander JG, Colonno RJ, Sidwell RW, Morrey JD. Characterization of antiviral activity of entecavir in transgenic mice expressing hepatitis B virus. Antiviral Res. 2003; 59(3):155–161.


41. Moriyama T, Guilhot S, Klopchin K, Moss B, Pinkert CA, Palmiter RD, Brinster RL, Kanagawa O, Chisari FV. Immunobiology and pathogenesis of hepatocellular injury in hepatitis B virus transgenic mice. Science. 1990; 248(4953):361–364.


42. Yang PL, Althage A, Chung J, Chisari FV. Hydrodynamic injection of viral DNA: a mouse model of acute hepatitis B virus infection. Proc Natl Acad Sci U S A. 2002; 99(21):13825–13830.


43. Huang LR, Wu HL, Chen PJ, Chen DS. An immunocompetent mouse model for the tolerance of human chronic hepatitis B virus infection. Proc Natl Acad Sci U S A. 2006; 103(47):17862–17867.


44. McCaffrey AP, Nakai H, Pandey K, Huang Z, Salazar FH, Xu H, Wieland SF, Marion PL, Kay MA. Inhibition of hepatitis B virus in mice by RNA interference. Nat Biotechnol. 2003; 21(6):639–644.


45. Lin SR, Yang HC, Kuo YT, Liu CJ, Yang TY, Sung KC, Lin YY, Wang HY, Wang CC, Shen YC, Wu FY, Kao JH, Chen DS, Chen PJ. The CRISPR/Cas9 System Facilitates Clearance of the Intrahepatic HBV Templates In Vivo. Mol Ther Nucleic Acids. 2014; 3:e186.


46. Yan Z, Zeng J, Yu Y, Xiang K, Hu H, Zhou X, Gu L, Wang L, Zhao J, Young JAT, Gao L. HBVcircle: A novel tool to investigate hepatitis B virus covalently closed circular DNA. J Hepatol. 2017; 66(6):1149–1157.


47. Dion S, Bourgine M, Godon O, Levillayer F, Michel ML. Adeno-associated virus-mediated gene transfer leads to persistent hepatitis B virus replication in mice expressing HLA-A2 and HLA-DR1 molecules. J Virol. 2013; 87(10):5554–5563.


48. Yang D, Liu L, Zhu D, Peng H, Su L, Fu YX, Zhang L. A mouse model for HBV immunotolerance and immunotherapy. Cell Mol Immunol. 2014; 11(1):71–78.


49. Ilan E, Burakova T, Dagan S, Nussbaum O, Lubin I, Eren R, Ben-Moshe O, Arazi J, Berr S, Neville L, Yuen L, Mansour TS, Gillard J, Eid A, Jurim O, Shouval D, Reisner Y, Galun E. The hepatitis B virus-trimera mouse: a model for human HBV infection and evaluation of anti-HBV therapeutic agents. Hepatology. 1999; 29(2):553–562.


50. Mercer DF, Schiller DE, Elliott JF, Douglas DN, Hao C, Rinfret A, Addison WR, Fischer KP, Churchill TA, Lakey JR, Tyrrell DL, Kneteman NM. Hepatitis C virus replication in mice with chimeric human livers. Nat Med. 2001; 7(8):927–933.


51. Sandgren EP, Palmiter RD, Heckel JL, Daugherty CC, Brinster RL, Degen JL. Complete hepatic regeneration after somatic deletion of an albumin-plasminogen activator transgene. Cell. 1991; 66(2):245–256.


52. Meuleman P, Libbrecht L, De Vos R, de Hemptinne B, Gevaert K, Vandekerckhove J, Roskams T, Leroux-Roels G. Morphological and biochemical characterization of a human liver in a uPA-SCID mouse chimera. Hepatology. 2005; 41(4):847–856.


53. Brezillon NM, DaSilva L, L'Hôte D, Bernex F, Piquet J, Binart N, Morosan S, Kremsdorf D. Rescue of fertility in homozygous mice for the urokinase plasminogen activator transgene by the transplantation of mouse hepatocytes. Cell Transplant. 2008; 17(7):803–812.


54. Heckel JL, Sandgren EP, Degen JL, Palmiter RD, Brinster RL. Neonatal bleeding in transgenic mice expressing urokinase-type plasminogen activator. Cell. 1990; 62(3):447–456.


55. Azuma H, Paulk N, Ranade A, Dorrell C, Al-Dhalimy M, Ellis E, Strom S, Kay MA, Finegold M, Grompe M. Robust expansion of human hepatocytes in Fah-/-/Rag2-/-/Il2rg-/- mice. Nat Biotechnol. 2007; 25(8):903–910.


56. Grompe M, al-Dhalimy M, Finegold M, Ou CN, Burlingame T, Kennaway NG, Soriano P. Loss of fumarylacetoacetate hydrolase is responsible for the neonatal hepatic dysfunction phenotype of lethal albino mice. Genes Dev. 1993; 7(12A):2298–2307.


57. Bissig KD, Wieland SF, Tran P, Isogawa M, Le TT, Chisari FV, Verma IM. Human liver chimeric mice provide a model for hepatitis B and C virus infection and treatment. J Clin Invest. 2010; 120(3):924–930.


58. Lai CL, Rosmawati M, Lao J, Van Vlierberghe H, Anderson FH, Thomas N, Dehertogh D. Entecavir is superior to lamivudine in reducing hepatitis B virus DNA in patients with chronic hepatitis B infection. Gastroenterology. 2002; 123(6):1831–1838.


59. Konishi M, Wu CH, Wu GY. Inhibition of HBV replication by siRNA in a stable HBV-producing cell line. Hepatology. 2003; 38(4):842–850.


60. Ristig MB, Crippin J, Aberg JA, Powderly WG, Lisker-Melman M, Kessels L, Tebas P. Tenofovir disoproxil fumarate therapy for chronic hepatitis B in human immunodeficiency virus/hepatitis B virus-coinfected individuals for whom interferon-alpha and lamivudine therapy have failed. J Infect Dis. 2002; 186(12):1844–1847.
61. Volz T, Allweiss L, Ben MBarek M, Warlich M, Lohse AW, Pollok JM, Alexandrov A, Urban S, Petersen J, Lutgehetmann M, Dandri M. The entry inhibitor Myrcludex-B efficiently blocks intrahepatic virus spreading in humanized mice previously infected with hepatitis B virus. J Hepatol. 2013; 58(5):861–867.

