Abstract
Background
Enterococcus faecalis strains with low-level resistance to linezolid (an oxazolidinone antibiotic) have become common. No large-scale study has examined the underlying mechanisms in linezolid-resistant E. faecalis (LRE) strains. We investigated these mechanisms and molecular characteristics in Chongqing, China.
Methods
A total of 1,120 non-duplicated E. faecalis strains collected from August 2014 to June 2017 underwent drug susceptibility testing. LRE strains were screened for optrA, cfr, and mutations in the 23S rRNA and ribosomal proteins L3 and L4 by PCR amplification and sequencing. Multi-locus sequence typing (MLST) and pulsed-field gel electrophoresis (PFGE) were used for epidemiological analysis.
Results
All 43 low-level LRE strains (minimum inhibitory concentration: 8–16 mg/L) harbored optrA; cfr and 23S rRNA mutations were not detected. Novel mutations in the ribosomal proteins L3 and L4—one deletion (Q103del) and four substitutions (S113L, T35A, I98V, and N79D)—were identified. Novel amino acid substitutions at positions E60K, G197D, and T285P of the OptrA protein were observed. MLST revealed 20 types of LRE strains; the most common type was ST16 (32.6%). PFGE showed 14 strains of ST16 with unique banding patterns. Eight novel sequence types (ST823 to ST830) and one allele (gki95) were identified for the first time in China.
Conclusions
optrA plays an important role in linezolid resistance and may serve as a marker for resistance screening. Since the L3 and L4 mutations did not simultaneously occur in the same strain, they play a negligible role in linezolid resistance. Epidemiological investigation suggested that the LRE cases were sporadic.
Linezolid is the main oxazolidinone antibiotic in clinical use for treating serious infections caused by multidrug-resistant gram-positive organisms [1]. It inhibits bacterial growth by overlapping binding sites at the ribosomal peptidyl transferase center and preventing the formation of the initiation complex necessary for protein synthesis [1]. Point mutations in the 23S ribosomal RNA, the target site of linezolid, are the most prevalent mechanism in linezolid-resistant Enterococcus faecalis (LRE) isolates [2]. The main types of mutations include G2576T, G2447U, and G2504A [34]. Alterations in the conserved domains of ribosomal proteins L3 and L4, encoded by rplC and rplD, respectively, have been reported to be a less important mechanism associated with linezolid resistance (minimum inhibitory concentrations [MICs] of 4–8 mg/L) in enterococci [5].
Recently, a highly effective and transferable form of resistance related to the RNA methyltransferase chloramphenicol/florfenicol resistance (cfr) gene has been associated with linezolid resistance in Enterococcus isolates [6]. A second transferable resistance gene, optrA, from E. faecalis E349, described as a plasmid-borne ATP-binding cassette (ABC) transporter, has also been identified as a mediator of novel efflux-mediated mechanism of oxazolidinone and phenicol resistance [7]. This ABC-type transporter mediates resistance to oxazolidinones (linezolid and tedizolid) and amide alcohols (chloramphenicol and florfenicol) through ribosomal protection and could be readily transferred among enterococci [789].
However, these mechanisms cannot explicitly explain some non-susceptible or low-level linezolid-resistant enterococci strains (MICs of 4–16 mg/L) identified in various antimicrobial resistance surveillance programs [5101112]. At present, there is no consensus on the definition of low-level linezolid-resistance in Enterococcus spp., while an MIC range of 8–16 mg/L has been recommended [13]. A high level of drug resistance is initially defined as MIC>64 mg/L [13]. Low-level resistance may serve as a stepping-stone for developing higher levels of resistance, which may lead to the evolution of hospital “superbugs” [14].
We investigated the molecular characteristics and mechanisms of low-level LRE isolates from the First Affiliated Hospital of Chongqing Medical University in Chongqing, southwest China.
A total of 1,120 non-duplicated E. faecalis strains were retrospectively obtained in the First Affiliated Hospital of Chongqing Medical University from August 2014 to June 2017. The strains were from different samples, such as urine, secretions, blood drainage tubes, seroperitoneum, purulence, bile, and puncture fluid, and were initially identified using the VITEK2 compact and API systems (BioMérieux, Lyon, France). Antimicrobial susceptibility to linezolid, clindamycin, dalfopristin, tetracycline, erythromycin, ciprofloxacin, moxifloxacin, levofloxacin, vancomycin, ampicillin, penicillin, tigecycline, streptomycin, and gentamicin was determined using AST-GP67 cards (BioMérieux). Results were interpreted according to the CLSI guidelines [15]. The MICs of the LRE strains screened as described above were confirmed using the broth microdilution method, following the CLSI guidelines [16]. The MIC used in this study was 8–16 mg/L. The reference strain E. faecalis ATCC 29212 served as a quality control for the broth microdilution method. Since the experiment did not involve animals, it did not require the approval of the Institutional Review Board.
The genomic DNA of each LRE isolate was extracted using the HiPure Bacterial DNA Kit (Magen, Guangzhou, China), according to the manufacturer's instructions and stored at −20℃ until use. The possible mechanisms of linezolid resistance, were screened by PCR using previously described primers and conditions: the 23S rRNA domain [17], ribosomal protein (L3 and L4) domain [6], the methyltransferase gene cfr [18], and ABC-type transporter gene optrA [7]. All positive PCR products were sequenced and blasted against the wild-type sequences from E. faecalis ATCC 29212 (GenBank Accession No. CP008816.1) and the complete optrA gene sequence from plasmid pE349 (GenBank Accession No. NG_048023.1). Nucleotide and deduced amino acid (AA) sequences were aligned using the multiple alignment algorithm in the MegAlign package (version 7.1.0; DNASTAR, Madison, WI) with Clustal W [19].
Multi-locus sequence typing (MLST) was performed by amplifying seven relatively conserved E. faecalis housekeeping genes (gdh, gyd, pstS, gki, aroE, xpt, and yqiL) [20] according to the database methodology and guidelines available at http://pubmlst.org/efaecalis/ [20]. For a more detailed analysis of the different phylogeny, we used PHYLOViZ to generate goeBURST diagrams of the typed isolates. The clonal relatedness between sequence types (STs) was analyzed by PHYLOViZ against the entire E. faecalis database (http://www.phyloviz.net) [21]. All identified novel STs and alleles were submitted to the PubMLST database. In addition, strains with the most common ST were subjected to further pulsed-field gel electrophoresis (PFGE) analysis, as previously described [18], which allows for more comprehensive evolutionary comparisons.
Among the 1,120 strains of Enterococcus faecalis, resistance was observed against linezolid (3.8%), clindamycin (3.8%), dalfopristin (3.8%), tetracycline (3.6%), erythromycin (3.5%), ciprofloxacin (2.3%), moxifloxacin (1.9%), and levofloxacin (2.2%). All strains were susceptible to vancomycin, ampicillin, penicillin, and tigecycline. Further, 2.1% and 2.3% exhibited high-level resistance to streptomycin and gentamicin, respectively. In total, 43 LRE strains (all exhibiting low-level resistance) were isolated with MICs of 8–16 mg/L, and 35 of these strains showed a multidrug-resistant phenotype (non-susceptibility to at least one agent in three antimicrobial categories) [22] (Table 1).
All 43 LRE strains were positive for the optrA gene. Mutations in domain V of the 23S rRNA genes as well as the presence of the methyltransferase cfr gene were not identified in any of the strains. Comparison of the OptrA amino acid sequences of the 43 LRE strains with that of the original OptrA from E. faecalis E349 (designated as the wild-type) revealed that 19 strains contained three novel point mutations at positions E60K, G197D, and T285P. A novel mutation in the rplC gene was observed in four LRE strains; the C333T substitution resulted in an S113L change in the L3 deduced amino acid sequence. Mutation analysis of the L4 protein from 31 LRE strains identified the T35A, I98V, and N79D novel substitutions and the Q103 novel deletion (Tables 1 and 2).
MLST showed that the 43 LRE strains could be classified into different STs (See Supplemental Data Table S1). Nine LRE strains exhibited eight novel E. faecalis sequence types (ST823 to ST830), and a new gki95 allele was also observed (and submitted to the PubMLST database) for the first time in China. GoeBURST analysis illustrated that none of the 43 strains exhibited clonal aggregation based on the available global datasets, suggesting that the infected cases presented were sporadic. Although MLST indicated that Strains 2, 3, 5, 8, 12, 13, 15, 21, 22, 27, 28, 33, 34, and 43 were all ST16, PFGE showed that they were of different types with 14 unique banding patterns (Lanes 1–14 represent the above-mentioned strains, respectively), indicating that MLST has a lower discriminatory ability than PFGE (Fig. 1).
Mutations in the central loop of domain V of the 23S rRNA gene have been considered the main mechanism of linezolid resistance among different clinical enterococci strains [2]. Since the first description of cfr-mediated linezolid resistance in an E. faecalis isolate (ST16) of human origin [6], few similar cases have been reported [7182324]. Our results did not reveal any 23S rRNA mutation sites; the presence of cfr seems to account for the high-level linezolid resistance by altering the drug target in linezolid-resistant strains [2526]. However, systematic surveillance showed that low-level LRE did not possess any known resistance mechanisms [2]. A few studies have examined low-level resistance together with the optrA gene [727].
The optrA gene was recently identified in low-level LRE strains lacking the cfr gene or mutations in the 23S ribosomal RNA and ribosomal protein L3 [282930]. Previously, we conducted a transcriptome analysis of LRE strains compared with a linezolid-susceptible strain; the results showed that the optrA gene was up-regulated in the LRE strains [31]. We have now presented several lines of evidence of a low-level linezolid resistance mechanism involving the optrA gene. Our data showed that all LRE strains harbored the optrA gene alone or in combination with mutations in ribosomal proteins L3 and L4. Several optrA gene sequence variants have been previously identified in isolates with the efflux phenotype, including the DK, ED, and KDP variants [273233]. In our study, the 43 LRE strains exhibited novel amino acid substitutions at positions of E60K, G197D, and T285P compared with E. faecalis E349. These mutation sites have been previously reported in China as those likely to elevate linezolid MICs in E. faecalis [2732]. Two important questions remaining are whether ABC transporter inhibitors are effective against optrA-positive strains and whether the presence of optrA can be verified by observing changes in MICs.
Another mechanism underlying linezolid resistance involves mutations in ribosomal proteins L3 and L4 [5343536]. Of the 43 LRE strains, 35 had mutations compared with E. faecalis ATCC-29212. Although these sequence changes resulted in amino acid changes, there were no differences in linezolid MICs among the 43 LRE strains. Previous studies have demonstrated the presence of mutations (N158S, F101L) in the L4 protein in linezolid-susceptible isolates, suggesting that some mutations may not be strongly associated with linezolid resistance [43236]. Since these mutations did not simultaneously occur in the same strain, they appear to play a negligible role in linezolid resistance. In addition, the possibility that several mechanisms contribute to decreasing linezolid sensitivity cannot be excluded.
MLST analysis revealed that the 43 LRE strains belonged to 20 STs, and ST16 was the most common type (14/43). The epidemiological investigation showed no clonal complex correlation between these isolates, indicating that the cases in our hospital were sporadic rather an outbreak. Furthermore, MLST demonstrated eight novel STs (ST823 to ST830) and one allele (gki95) identified for the first time in China. These low-level LRE isolates could not be attributed to clonal dissemination, as determined by MLST and PFGE analysis. Interestingly, isolates with differently related PFGE patterns had the same ST (ST16). This indicates that PFGE is more discriminatory than MLST for homology analysis of small areas in the short term, such as the examination of hospital or ward isolates.
This study is the first to collect a large number of LRE isolates and to explore the mechanisms underlying low-level linezolid resistance in southwest China. The high prevalence of the optrA gene in LRE strains indicates that it may serve as a useful marker for linezolid resistance screening. Further studies are required to determine the relationship between optrA variants and linezolid resistance and the transmission mechanism. Furthermore, routine and persistent screening for optrA gene risk factors associated with the rapid occurrence and dissemination of linezolid resistance is urgently required.
Acknowledgment
This work was supported by a grant from the National Natural Science Foundation of China (Grant No. 81572055).
References
1. Kloss P, Xiong L, Shinabarger DL, Mankin AS. Resistance mutations in 23 S rRNA identify the site of action of the protein synthesis inhibitor linezolid in the ribosomal peptidyl transferase center. J Mol Biol. 1999; 294:93–101. PMID: 10556031.
2. Mendes RE, Deshpande LM, Jones RN. Linezolid update: stable in vitro activity following more than a decade of clinical use and summary of associated resistance mechanisms. Drug Resist Updat. 2014; 17:1–12. PMID: 24880801.
3. Bonilla H, Huband MD, Seidel J, Schmidt H, Lescoe MK, McCurdy SP, et al. Multicity outbreak of linezolid-resistant Staphylococcus epidermidis associated with clonal spread of a cfr-containing strain. Clin Infect Dis. 2010; 51:796–800. PMID: 20726771.
4. Wong A, Reddy SP, Smyth DS, Aguero-Rosenfeld ME, Sakoulas G, Robinson DA. Polyphyletic emergence of linezolid-resistant staphylococci in the United States. Antimicrob Agents Chemother. 2010; 54:742–748. PMID: 19933808.
5. Chen H, Wu W, Ni M, Liu Y, Zhang J, Xia F, et al. Linezolid-resistant clinical isolates of enterococci and Staphylococcus cohnii from a multicentre study in China: molecular epidemiology and resistance mechanisms. Int J Antimicrob Agents. 2013; 42:317–321. PMID: 23880167.
6. Diaz L, Kiratisin P, Mendes RE, Panesso D, Singh KV, Arias CA. Transferable plasmid-mediated resistance to linezolid due to cfr in a human clinical isolate of Enterococcus faecalis. Antimicrob Agents Chemother. 2012; 56:3917–3922. PMID: 22491691.
7. Wang Y, Lv Y, Cai J, Schwarz S, Cui L, Hu Z, et al. A novel gene, optrA, that confers transferable resistance to oxazolidinones and phenicols and its presence in Enterococcus faecalis and Enterococcus faecium of human and animal origin. J Antimicrob Chemother. 2015; 70:2182–2190. PMID: 25977397.
8. Cai J, Wang Y, Schwarz S, Zhang G, Chen S, Gu D, et al. High detection rate of the oxazolidinone resistance gene optrA in Enterococcus faecalis isolated from a Chinese anorectal surgery ward. Int J Antimicrob Agents. 2016; 48:757–759. PMID: 27751624.
9. Sharkey LK, Edwards TA, O'Neill AJ. ABC-F proteins mediate antibiotic resistance through ribosomal protection. MBio. 2016; 7:e01975-15. PMID: 27006457.
10. Jones RN, Ross JE, Bell JM, Utsuki U, Fumiaki I, Kobayashi I, et al. Zyvox Annual Appraisal of Potency and Spectrum program: linezolid surveillance program results for 2008. Diagn Microbiol Infect Dis. 2009; 65:404–413. PMID: 19913683.
11. Ross JE, Farrell DJ, Mendes RE, Sader HS, Jones RN. Eight-year (2002-2009) summary of the linezolid (Zyvox® Annual Appraisal of Potency and Spectrum; ZAAPS) program in European countries. J Chemother. 2011; 23:71–76. PMID: 21571621.
12. Patel SN, Memari N, Shahinas D, Toye B, Jamieson FB, Farrell DJ. Linezolid resistance in Enterococcus faecium isolated in Ontario, Canada. Diagn Microbiol Infect Dis. 2013; 77:350–353. PMID: 24095643.
13. Cho SY, Kim HM, Chung DR, Kim SH, Huh HJ, Kang CI, et al. Resistance mechanisms and clinical characteristics of linezolid-resistant Enterococcus faecium isolates: a single-centre study in South Korea. J Glob Antimicrob Resist. 2018; 12:44–47. PMID: 28941790.
14. Baquero F. Low-level antibacterial resistance: a gateway to clinical resistance. Drug Resist Updat. 2001; 4:93–105. PMID: 11512526.
15. CLSI. Performance standards for antimicrobial susceptibility testing. M02-A12, M07-A10, and M11-A8. M100-table 2D. Wayne, PA: Clinical and Laboratory Standards Institute;2017.
16. CLSI. Methods for dilution antimicrobial tests for bacteria that grow aerobically. Approved standard. 10th ed. CLSI M07-A10. 10th ed. Wayne, PA: Clinical and Laboratory Standards Institute;2015.
17. Bourgeois-Nicolaos N, Massias L, Couson B, Butel MJ, Andremont A, Doucet-Populaire F. Dose dependence of emergence of resistance to linezolid in Enterococcus faecalis in vivo. J Infect Dis. 2007; 195:1480–1488. PMID: 17436228.
18. Wang L, He Y, Xia Y, Wang H, Liang S. Investigation of mechanism and molecular epidemiology of linezolid-resistant Enterococcus faecalis in China. Infect Genet Evol. 2014; 26:14–19. PMID: 24815727.
19. Clewley JP, Arnold C. MEGALIGN. The multiple alignment module of Lasergene. Methods Mol Biol. 1997; 70:119–129. PMID: 9089607.
20. Ruiz-Garbajosa P, Bonten MJ, Robinson DA, Top J, Nallapareddy SR, Torres C, et al. Multilocus sequence typing scheme for Enterococcus faecalis reveals hospital-adapted genetic complexes in a background of high rates of recombination. J Clin Microbiol. 2006; 44:2220–2228. PMID: 16757624.
21. Francisco AP, Vaz C, Monteiro PT, Melo-Cristino J, Ramirez M, Carriço JA. PHYLOViZ: phylogenetic inference and data visualization for sequence based typing methods. BMC Bioinformatics. 2012; 13:87. PMID: 22568821.
22. Magiorakos AP, Srinivasan A, Carey RB, Carmeli Y, Falagas ME, Giske CG, et al. Multidrug-resistant, extensively drug-resistant and pandrug-resistant bacteria: an international expert proposal for interim standard definitions for acquired resistance. Clin Microbiol Infect. 2012; 18:268–281. PMID: 21793988.
23. Liu Y, Wang Y, Schwarz S, Wang S, Chen L, Wu C, et al. Investigation of a multiresistance gene cfr that fails to mediate resistance to phenicols and oxazolidinones in Enterococcus faecalis. J Antimicrob Chemother. 2014; 69:892–898. PMID: 24272266.
24. Li B, Ma CL, Yu X, Sun Y, Li MM, Ye JZ, et al. Investigation of mechanisms and molecular epidemiology of linezolid nonsusceptible Enterococcus faecalis isolated from a teaching hospital in China. J Microbiol Immunol Infect. 2016; 49:595–599. PMID: 26210761.
25. Ikonomidis A, Grapsa A, Pavlioglou C, Demiri A, Batarli A, Panopoulou M. Accumulation of multiple mutations in linezolid-resistant Staphylococcus epidermidis causing bloodstream infections; in silico analysis of L3 amino acid substitutions that might confer high-level linezolid resistance. J Chemother. 2016; 28:465–468. PMID: 27077930.
26. Locke JB, Hilgers M, Shaw KJ. Mutations in ribosomal protein L3 are associated with oxazolidinone resistance in staphylococci of clinical origin. Antimicrob Agents Chemother. 2009; 53:5275–5278. PMID: 19805557.
27. Cai J, Wang Y, Schwarz S, Lv H, Li Y, Liao K, et al. Enterococcal isolates carrying the novel oxazolidinone resistance gene optrA from hospitals in Zhejiang, Guangdong, and Henan, China, 2010-2014. Clin Microbiol Infect. 2015; 21:1095.e1–1095.e4.
28. Flamm RK, Mendes RE, Hogan PA, Streit JM, Ross JE, Jones RN. Linezolid surveillance results for the United States (LEADER Surveillance Program 2014). Antimicrob Agents Chemother. 2016; 60:2273–2280. PMID: 26833165.
29. Cavaco LM, Bernal JF, Zankari E, Léon M, Hendriksen RS, Perez-Gutierrez E, et al. Detection of linezolid resistance due to the optrA gene in Enterococcus faecalis from poultry meat from the American continent (Colombia). J Antimicrob Chemother. 2017; 72:678–683. PMID: 27999039.
30. Tamang MD, Moon DC, Kim SR, Kang HY, Lee K, Nam HM, et al. Detection of novel oxazolidinone and phenicol resistance gene optrA in enterococcal isolates from food animals and animal carcasses. Vet Microbiol. 2017; 201:252–256. PMID: 28284617.
31. Hua RY, Xia Y, Wu WY, Yan J, Yang M. Whole transcriptome analysis reveals potential novel mechanisms of low-level linezolid resistance in Enterococcus faecalis. Gene. 2018; 647:143–149. PMID: 29325735.
32. Cui L, Wang Y, Lv Y, Wang S, Song Y, Li Y, et al. Nationwide surveillance of novel oxazolidinone resistance gene optrA in Enterococcus isolates in China from 2004 to 2014. Antimicrob Agents Chemother. 2016; 60:7490–7493. PMID: 27645239.
33. Li D, Wang Y, Schwarz S, Cai J, Fan R, Li J, et al. Co-location of the oxazolidinone resistance genes optrA and cfr on a multiresistance plasmid from Staphylococcus sciuri. J Antimicrob Chemother. 2016; 71:1474–1478. PMID: 26953332.
34. Long KS, Vester B. Resistance to linezolid caused by modifications at its binding site on the ribosome. Antimicrob Agents Chemother. 2012; 56:603–612. PMID: 22143525.
35. Mendes RE, Deshpande LM, Farrell DJ, Spanu T, Fadda G, Jones RN. Assessment of linezolid resistance mechanisms among Staphylococcus epidermidis causing bacteraemia in Rome, Italy. J Antimicrob Chemother. 2010; 65:2329–2335. PMID: 20841419.
36. Mendes RE, Flamm RK, Hogan PA, Ross JE, Jones RN. Summary of linezolid activity and resistance mechanisms detected during the 2012 LEADER surveillance program for the United States. Antimicrob Agents Chemother. 2014; 58:1243–1247. PMID: 24323470.
SUPPLEMENTARY MATERIAL
Supplemental Data Table S1
Epidemiological analysis of 43 linezolid-resistant Enterococcus faecalis strains using MLST
Fig. 1
Epidemiology analysis of low-level linezolid-resistant Enterococcus faecalis isolates by MLST and PFGE. (A) GoeBURST diagram for the MLST data set of 43 linezolid-resistant E. faecalis isolates. The eBURST diagram was calculated using PHYLOViZ with the goeBURST algorithm; it contains 20 unique STs for a total of 43 isolates in the database. Each circle represents one ST and the size represents the number of strains in each ST. (B) PFGE fingerprinting dendrogram and PFGE pattern of 14 linezolid-resistant ST16 E. faecalis strains
Abbreviations: ST, sequence type; MLST, multi-locus sequence typing; PFGE, pulsed field gel electrophoresis.
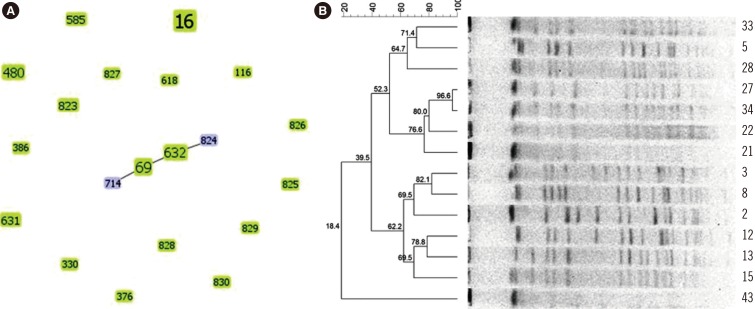
Table 1
General characteristics and resistance mechanisms of 43 low-level linezolid-resistant Enterococcus faecalis isolates
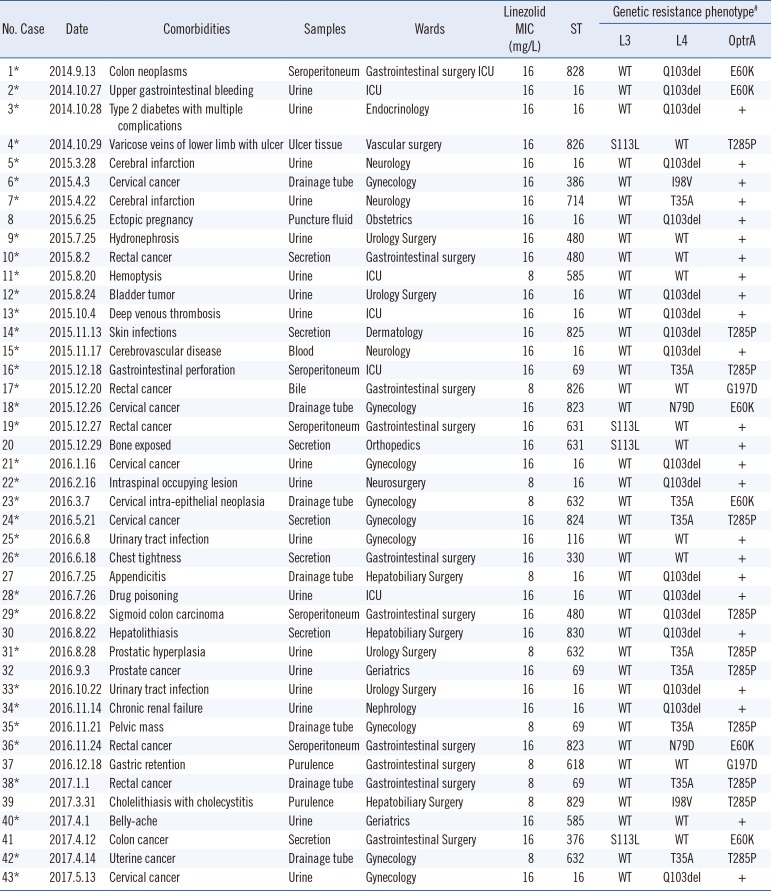
*Multi-drug resistant phenotype; #23S rRNA mutations and cfr gene were not detected.
Abbreviations: ICU, intensive care unit; MIC, minimum inhibitory concentration; ST, sequence type; rRNA, ribosomal RNA; WT, wild-type; T, Thr; A, Ala; P, Pro; I, Ile; V, Val; Q, Gln; S, Ser; L, Leu; E, Glu; K, Lys; N, Asn; D, Asp; G, Gly.
Table 2
OptrA protein polymorphisms detected in 43 optrA-positive linezolid-resistant Enterococcus faecalis strains
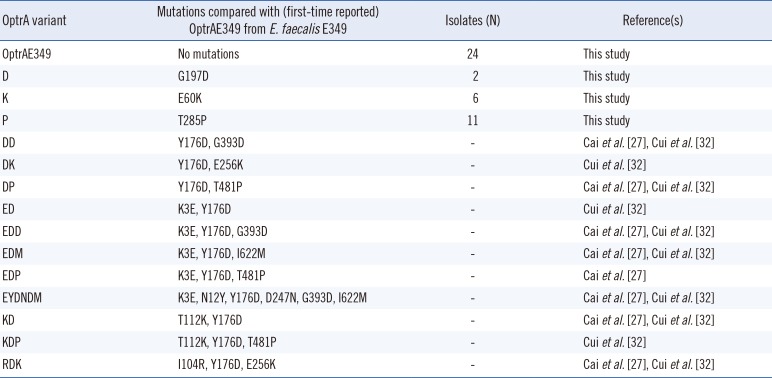
OptrA variant | Mutations compared with (first-time reported) OptrAE349 from E. faecalis E349 | Isolates (N) | Reference(s) |
---|---|---|---|
OptrAE349 | No mutations | 24 | This study |
D | G197D | 2 | This study |
K | E60K | 6 | This study |
P | T285P | 11 | This study |
DD | Y176D, G393D | - | Cai et al.[27], Cui et al.[32] |
DK | Y176D, E256K | - | Cui et al.[32] |
DP | Y176D, T481P | - | Cai et al.[27], Cui et al.[32] |
ED | K3E, Y176D | - | Cui et al.[32] |
EDD | K3E, Y176D, G393D | - | Cai et al.[27], Cui et al.[32] |
EDM | K3E, Y176D, I622M | - | Cai et al.[27], Cui et al.[32] |
EDP | K3E, Y176D, T481P | - | Cai et al.[27] |
EYDNDM | K3E, N12Y, Y176D, D247N, G393D, I622M | - | Cai et al.[27], Cui et al.[32] |
KD | T112K, Y176D | - | Cai et al.[27], Cui et al.[32] |
KDP | T112K, Y176D, T481P | - | Cui et al.[32] |
RDK | I104R, Y176D, E256K | - | Cai et al.[27], Cui et al.[32] |