Abstract
Purpose
The cyclin-dependent kinase 1 (Cdk1) and cyclin B complex performs important roles in the transition from the G2 to M phase in the cell cycle through removal of inhibitory phosphates on Cdk1, and Cdc25B, which is a dual-specific phosphatase, mediates these dephosphorylation events. However, measuring Cdc25B activity by existing methods is hampered by inadequate nonspecific substrates and the need to use a radiolabeled isotope. The present study aimed to develop an improved method with which to properly measure Cdc25B activity using a novel nonradioisotopic assay and Cdc25B overexpression cell lines.
Materials and Methods
A nonradioisotopic Cdk1 kinase assay, based on Western blotting for retinoblastoma protein and histone H1, was used to analyze Cdc25B activity. Also, stable Cdc25B2 and Cdc25B3 overexpression HeLa cell lines were constructed using the tetracycline-regulated expression system and were applied as a tool for screening for inhibitors of Cdc25B.
Results
The present study developed and optimized a nonradioisotopic assay method to properly measure Cdc25B activity. Furthermore, we constructed stable Cdc25B2 and Cdc25B3 overexpression HeLa cell lines for the establishment of a strong assay system with which to evaluate the specificity of Cdc25B inhibitors under conditions similar to the intracellular environment. These methods were confirmed as useful tools for measuring Cdc25B activity.
The cell division cycle 25 (Cdc25) family, which consists of Cdc25A, Cdc25B, and Cdc25C, is a highly conserved family of dual specificity phosphatases that activate a few cyclin-dependent kinase (CDK) complexes, which in turn regulate progression through cell cycle.1 The cyclin-dependent kinase 1 (Cdk1 or Cdc2) and cyclin B protein complex, Cdk1 and cyclin A complex, Cdk2 and cyclin A complex, and Cdk2 and cyclin E complex play important roles in cell cycle regulation.2 Of these, the Cdk1 and cyclin B complex is known to be a key regulator in cell cycle transition from the G2 to M phase by dephosphorylation of the 14th threonine and 15th tyrosine residues of Cdk1, which is mediated by Cdc25B.345 Cdc25B phosphatases are also key components of checkpoint pathways that become activated in the event of DNA damage.1 Recent studies have confirmed that cell cycle regulators are closely related to cancer. Cdc25 overexpression has been reported in several types of human cancers on analysis of patient tissues and cells.6 The overexpression of Cdc25B in patient tissues has been reported in esophageal cancer, colon cancer, blood cancer, and lung cancer. Cdc25B has been predicted to be a target of cancer drugs.7
However, methods for measuring Cdc25B activity and the screening assays for drugs targeting Cdc25B are difficult to use and have some problems. The most common method for measuring the activity of the Cdc25B phosphatase uses p-nitrophenyl phosphate or o-methylfluorescein phosphate as a substrate.8910 Since these substrates are nonspecific, the activity of Cdc25B is often interpreted incorrectly.11 Another method using γ-32P-radiolabeling of histone H1 by Cdk1, which is the most important physiological substrate of Cdc25B,121314 improves substrate specificity, but is dangerous and inconvenient to use because of the radiolabeled isotope. Therefore, an improved method to analyze Cdc25B activity is needed that can satisfy both higher substrate specificity and ease of use. In this study, we developed a nonradioisotopic Cdk1 kinase assay method to properly measure Cdc25B activity. The method uses Western blotting to assess physiological substrates of Cdk1, such as retinoblastoma protein (Rb)1516 and histone H1, to overcome the inconvenience of use and limited accessibility of the existing experimental tools. We specifically used antibodies recognizing phosphorylation of the Ser795 residue of Rb or the phospho-Thr-Pro motif of histone H1.
Our general dephosphorylation method uses partial Cdc25B or GST-fused Cdc25B produced in Escherichia coli,17 which could lead to an inadequate or non-specific reaction in comparison to full-length Cdc25B (with post-translational modifications) produced in human cells. Thus, Cdc25B overexpression in human cells is required to produce full-length and fully post-translationally modified Cdc25B, as Cdc25B has been reported to be slightly expressed at the protein level. However, HeLa cells, which are the most frequently used human cell line for cancer research, exhibit a lethal phenotype if Cdc25B is constitutively expressed.18 Therefore, in this study, Cdc25B overexpression HeLa cell lines were constructed using an inducible expression system of Cdc25B2 or Cdc25B3, the major forms of the five splice forms, under the control of tetracycline to avoid the lethal phenotype of Cdc25. In addition, we developed in vitro dephosphorylation assay as a specific and simple tool for screening for Cdc25B inhibitors under conditions similar to the intracellular environment using cell-free extracts of the overexpression cell lines.
Consequently, we developed a new assay system that will replace general methods in terms of specificity and ease of use in screening for Cdc25B inhibitors as anti-cancer drugs. In addition, it is expected that Cdc25B2 or Cdc25B3 overexpression cell lines can be utilized as a useful tool for screening subtype selectivity of Cdc25B small interfering RNA (siRNA) candidates and to better understand the function of Cdc25B in physiological conditions.
Compound 5 (CPD5), a synthetic vitamin K analog [2-(2-mercaptoethanol)-3-methyl-1,4-naphthoquinone], was used as a Cdc25 inhibitor.12
To construct stable HeLa cell lines overexpressing Cdc25B2 or Cdc25B3, the tetracycline-regulated expression system was used to make a system with higher levels of induced expression than those obtained with other regulated mammalian expression systems. To obtain CDC25B2 or CDC25B3 genes, the pGEX-KG/Cdc25B2 and pGEX-KG/Cdc25B3 plasmids, which are GST-fusion expression vectors, were digested with Hind III and BamHI. The blunted Hind III/BamHI fragments containing CDC25B2 or CDC25B3 were inserted into pcDNA4/TO digested with EcoRV/BamHI. Insert orientation and integrity were confirmed by restriction mapping and sequencing.
pcDNA4/TO with either the CDC25B2 or CDC25B3 gene was transfected into the T-RExTM-HeLa cell line (Gibco-BRL, Grand Island, NY, USA) by the Fugene transfection protocol (Roche Molecular Biochemical, Mannheim, Germany). After 3 weeks, colonies resistant to zeocin (50 μg/mL) were selected and confirmed to be HeLa cell line overexpressing Cdc25B2 or Cdc25B3 through RT-PCR and Western blotting. The cells were stimulated by adding tetracycline (1 μg/mL) for 24 hours to induce Cdc25B2 or Cdc25B3 protein expression.
T-RExTM-HeLa cells stably express the Tet repressor, and these cells were cultured in MEM supplemented with blasticidin (6 μg/mL), 10% tetracycline-reduced fetal bovine serum (HyClone, Logan, UT, USA), 2 mM glutamine, 0.1 mM non-essential amino acids, 1.0 mM sodium pyruvate, penicillin (100 U/mL), and streptomycin (100 μg/mL) in a 5% CO2 humidified atmosphere.
HeLa cells were maintained in RPMI medium (Life Technology, Gaithersburg, MD, USA) supplemented with 10% fetal bovine serum and antibiotics at 37℃. For kinase assay experiments and cell cycle analysis, HeLa cells were treated with 250 nM nocodazole (Sigma, St. Louis, MO, USA) to induce G2/M cell cycle arrest, 10 μM CPD5 as a Cdc25B inhibitor, or 0.1% DMSO as vehicle for 24 hours.
The Cdc25B overexpression HeLa cells were arrested in the G2 phase by the addition of 800 nM etoposide for 20 to 24 hours prior to induction. G2 phase-arrested cells were washed with PBS and then incubated in fresh medium in the presence or absence of tetracycline for 16 hours.
All cells, both attached and floating, were harvested and washed with PBS. The cells were then fixed in 70% ethanol at −20℃ overnight and stained with propidium iodide (50 μg/mL) and RNase A (1 mg/mL) for cell cycle analysis using the FACScan system (Becton Dickinson, Mountain View, CA, USA).
Total protein extracts were prepared with cell lysis buffer (Cell Signaling Technology, Beverly, MA, USA) according to the manufacturer's instructions, and the concentrations were quantified using the BCA protein assay kit (Pierce, Rockford, IL, USA).
The general Western blot protocols used in this study were described in Molecular Cloning: A Laboratory Manual, 2nd edition.19 The primary antibodies used included anti-Cdk1 (Santa Cruz Biotechnology, Santa Cruz, CA, USA), anti-pTyr15 Cdk1 (Sigma), anti-cyclin B (Santa Cruz Biotechnology), anti-pSer795 Rb (Cell Signaling Technology), and anti-pThr/Pro motif-recognizing antibodies (Cell Signaling Technology). Antibody signals were detected using horseradish peroxidase-conjugated secondary antibodies and enhanced chemiluminescence (luminol) according to the manufacturer's instructions (Santa Cruz Biotechnology).
Total RNA was prepared with RNA extraction columns (Qiagen, West Sussex, UK), and RNA concentrations in each sample were determined spectrophotometrically. Using 2 μg of total RNA as a template and oligo-dT primers (Invitrogen, San Diego, CA, USA), RT-PCR was performed with Superscript II RNase H reverse transcriptase (Invitrogen).
Total protein extracts (100 μg) prepared from HeLa cells treated with 250 nM nocodazole were immunoprecipitated using an anti-cyclin B antibody to obtain Cdk1 immunoprecipitates (IP). The Cdk1 IP or five units of purified Cdk1 (NEB, Beverly, MA, USA) were incubated in 20 μL of kinase buffer containing 50 mM Tris-HCl pH 7.5, 10 mM MgCl2, 1 mM EGTA, 2 mM DTT, 1 μg of Rb (Cell Signaling Technology) or 2 μg of histone H1 (Roche, Meylan, France) as substrate, and 500 μM ATP at 30℃ for 30 minutes. The reaction was terminated by adding 5× SDS sample loading buffer, boiled for 5 minutes, and separated by SDS-PAGE. The proteins in the gel were transferred to a nitrocellulose membrane and probed with anti-pSer795 Rb antibody (Cell Signaling Technology) or anti-pThr/Pro motifrecognizing antibody (Cell Signaling Technology). The signals were detected by chemiluminescence and analyzed with a FluorChemTM 8800 image analyzer (Alpha-Innotech, San Leandro, CA, USA).
Phospho-Cdk1 IP was prepared by immunoprecipitation with a monoclonal Cdk1 antibody from HeLa cell extracts treated with CPD5. Cdc25B2 or Cdc25B3 overexpression HeLa cell lysates were prepared using cell lysis buffer (Cell Signaling Technology) without phosphatase inhibitors and were cleared of endogenous Cdk1 and cyclin B proteins by the immuno-depletion method. The Cdk1- and cyclin B-depleted lysate was incubated with high phospho-Cdk1 IP in phosphatase buffer with or without compounds for 60 minutes at 37℃. The dephosphorylation reaction was terminated by adding 5× SDS sample loading buffer. Western blotting for the detection of dephosphorylated Cdk1 was performed with anti-pTyr15 Cdk1 and anti-Cdk1 antibodies.
In-gel digestion was performed according to Applied Biosystems' instructions. The Coomassie blue-stained bands were cut out and washed with 50% acetonitrile/25 mM ammonium bicarbonate (pH 8.0) solution three times. Gels were dehydrated by soaking in 100% acetonitrile and dried in a vacuum centrifuge. The gel pieces were then incubated in 20 μL of digestion buffer containing 25 mM ammonium bicarbonate (pH 8.0) and 10 μg/mL of trypsin (Promega, Madison, WI, USA) in an ice-cold bath. After 30 minutes, the supernatant was removed and replaced with 10 μL of 25 mM ammonium bicarbonate buffer. Thereafter, the gel was incubated at 37℃ for 16 hours. Peptides were extracted twice with 30 μL of 50% acetonitrile/5% TFA solution for 60 minutes and dried in a vacuum centrifuge. Three microliters of the matrix (α-cyano–4 hydroxycinnamic acid) dissolved in 100% acetonitrile/0.1% TFA solution was mixed with the extracted peptides. Then, the peptide mixture (1 μL) was deposited on a MALDI plate and dried at room temperature. The peptide spectra were detected with MALDI-TOF Voyager DE-STR (Applied Biosystems, Framingham, CA, USA). The general procedure related to MALDI-TOF was carried out according to Applied Biosystems' instructions, and the peptide peaks were analyzed by searching the ProteinProspector MS-FIT database.
Four siRNAs were chemically synthesized by Invitrogen. HeLa cells and Cdc25B overexpression HeLa cells were cultured at 37℃. The cells were plated at approximately 70% confluence for 16 hours before transfection. Lipofectamine (Invitrogen)-mediated transient co-transfection of siRNAs was performed as described by the manufacturer for adherent cell lines. Cells were incubated in the transfection mixture for 6 hours with 100 nM siRNA1, 100 nM siRNA2, 50 nM siRNA3, or 50 nM siRNA4. After transfection, the cells were further cultured in antibiotic-free media for 30 hours, and total protein extracts were prepared as previously described. Specifically, Cdc25B overexpression HeLa cells were stimulated by adding tetracycline (1 μg/mL) to induce Cdc25B2 or Cdc25B3 protein production after transfection.
The inhibition of cell proliferation was measured by MTT [3-(4,5-dimethylthiazol-2-yl)-2,5-diphenyltetrazolium bromide] assay. MTT was added to the cells that were transfected with siRNA for 4 hours, and absorbance was measured at 540 nm.
In this study, we aimed to develop a nonradioisotopic Cdk1 kinase assay method with which to properly measure Cdc25B activity using a Western blotting method targeting substrates of Cdk1, such as Rb and histone H1.202122 Two kinds of Cdk1 were prepared: one was commercially available, purified Cdk1 made by NEB, and the other was Cdk1 immunoprecipitated from HeLa cell extracts using anti-cyclin B antibody. The purified Cdk1 kinase or Cdk1 IP was incubated with Rb or histone H1 substrates. After the reaction was terminated, phosphorylation levels were detected by Western blot. The Rb and histone H1 substrates were phosphorylated by Cdk1 (Fig. 1A and B, lanes 1 and 5). In the presence of the Cdk1 kinase inhibitor olomoucine, the phosphorylation rate was dramatically decreased, as predicted (lanes 2 and 6). Unlike histone H1, Rb has never been used as a Cdk1 substrate before this study; therefore, we confirmed that the phospho-Ser795 site of Rb was phosphorylated by Cdk1 using MALDI-TOF with in-gel digestion (data not shown). These results indicated that Cdk1 kinase activity can be measured by this nonradioisotopic method using Cdk1 immunoprecipitated from cell extracts, as well as commercially available Cdk1.
To optimize the nonradioisotopic Cdk1 kinase assay, several factors that may influence results were examined. The amount of total protein from HeLa cell extracts to obtain Cdk1 IP, the amount of Rb or histone H1 substrate, and the duration of the reaction time were shown to be positively correlated with phosphorylation levels (Fig. 2). Exceeding a certain ATP concentration, however, did not affect the reaction. These results suggested that the nonradioisotopic method is applicable as an assay to measure Cdk1 activity.
To confirm if the Cdk1 kinase assay can measure Cdc25B activity, HeLa cells treated with DMSO as a negative control; with CPD5, a synthetic vitamin K analog [2-(2-mercaptoethanol)-3-methyl-1,4-naphthoquinone], as a Cdc25B inhibitor;12 or with nocodazole to induce G2/M cell cycle arrest by blocking tubulin polymerization were used. The cell-cycle state of each condition was confirmed by FACS analysis (Fig. 3A). The expression levels of Cdk1 and phosphorylated Cdk1 were detected by Western blot analysis of Cdk1 IP (Fig. 3B). As expected, nocodazole induced the dephosphorylation of Cdk1 during G2/M arrest, and CPD5 increased dramatically Cdk1 phosphorylation by inhibiting Cdc25B, compared to the negative control DMSO. Furthermore, with Cdk1 IP, we also conducted Cdk1 kinase assay to measure Cdc25B activity as described previously (Fig. 3C), and we found that Cdk1 kinase activity was inversely proportional to the phosphorylation level of Cdk1 protein, as predicted. In addition, we found similar results when the same test was carried out with in-house Cdc25B inhibitor candidates (data not shown). Therefore, these results suggested that this assay can be effectively used not only in screening for Cdk1 inhibitors, but also for developing Cdc25B inhibitors by treating cells directly therewith.
It has been reported that Cdc25B has oncogenic properties and is overexpressed in head and neck, colorectal, and breast cancer.6 However, among five splicing variants of Cdc25B, only Cdc25B2 and Cdc25B3 are slightly expressed at the protein level, whereas the other spliced forms have only been detected at the transcript level. In this study, because Cdc25B2 and Cdc25B3 are the major splice forms and have oncogenic properties, we constructed stable Cdc25B2 or Cdc25B3 overexpression HeLa cell lines under the control of tetracycline (to avoid the lethal phenotype caused by constitutive expression of Cdc25B protein) to establish a strong assay system with which to evaluate the specificity of Cdc25B inhibitors, such as small molecule inhibitors or siRNA. The inducible cell lines were selected, and mRNA and protein levels were confirmed by RT-PCR and Western blot, respectively (Fig. 4A and B). Biological activities were also confirmed through two assays: the release of cells arrested in G2 phase into G1 phase and increase of Cdk1 kinase activity (Fig. 4C and D). Cdc25B2 protein induced by tetracycline pushed G2/M-arrested cells into G1 phase, and the activity of Cdk1, which is the physiological substrate of Cdc25B, was higher than that induced in the absence of tetracycline. However, the biological activities were lower than expected, and we considered that the activity of overexpressed Cdc25B might be regulated by cellular feedback.
To better understand the function of Cdc25B2 and Cdc25B3 in physiological conditions, we performed two-dimensional gel electrophoresis and MALDI-TOF mass spectrometry experiments to identify differentially expressed or post-translationally modified (PTM) proteins in the stable cell lines. We found four differentially upregulated and four PTM spots (data not shown). Each protein spot was identified by peptide mass fingerprinting of MALDI-TOF and searching the ProteinProspector MS-Fit database. The four upregulated proteins were identified as vimentin, hnRNP K [transformation upregulated nuclear protein (TUNP)], heat shock cognate 71 kDa protein, and annexin II. Three out of the four hits for PTM proteins were all for alpha enolase, and one of the PTM proteins was identified as Transgelin 2 (Table 1). It is especially notable that vimentin and heat shock cognate 71 kDa protein were upregulated specifically in the Cdc25B3-induced cell line. These results may help to reveal the function of Cdc25B2 and Cdc25B3 in physiological conditions.
To directly screen for inhibitors of Cdc25B at the in vitro level, a dephosphorylation assay using Cdc25B overexpression cell lines was developed. This method can be used as a specific and simple screening tool for Cdc25B inhibitors under conditions similar to the intracellular environment by using cell-free extracts depleted of Cdk1 and cyclin B without purification of activated Cdc25B. The in vitro dephosphorylation reaction was performed as described in the Materials and Methods. As shown in Fig. 5, the dephosphorylation assay using phosphatase inhibitors (lane 4 in Fig. 5A and B) showed similar phosphorylation of Cdk1 compared to that of the control (lane 2 in Fig. 5A and B), suggesting that the inhibitors blocked Cdc25B activity. In addition, CPD5, a known inhibitor of Cdc25B, showed similar results (lane 6 in Fig. 5A), although the phosphorylation level was lower than that observed for the phosphatase inhibitors, suggesting that the results were obtained via the same pathway. Interestingly, CPD5 was ineffective at inhibiting Cdc25B3 activity (lane 6 in Fig. 5B) and specifically inhibited Cdc25B2. Taken together, these results demonstrated that the dephosphorylation assay developed in this study can be used not only as a useful tool for measuring Cdc25B activity but also as a tool for confirming the activity of specific splice variants of Cdc25B.
In previous studies, we have confirmed that it is not possible to evaluate the inhibitory activity of siRNA using the HeLa cell line because it was difficult to detect Cdc25B2 or Cdc25B3 at the protein level (data not shown). Therefore, herein, we studied whether Cdc25B overexpression cell lines could be used as a siRNA screening tool. As shown in Fig. 6A, four siRNAs were designed and constructed to mediate specific gene silencing through an interaction with the RNA-induced silencing complex. It has been reported that exon 4 of CDC25B2 consists of only a portion of the sequence of exon 4 of CDC25B3, and the remaining exon and intron sequences are the same.18 In the present study, the siRNA1 construct targeted a portion of the sequence in exon 4 of CDC25B; the siRNA2 construct targeted the intron between exons 4 and 5; and the siRNA3 and siRNA4 constructs targeted the intron between exons 5 and 6. Cdc25B mRNAs were prepared and measured by RT-PCR after 24 hours of transfection of each siRNA (data not shown), and Cdc25B protein was also extracted and measured by Western blot (Fig. 6B). Each siRNA selectively down-regulated both the mRNA and protein expression levels of Cdc25B. Among the constructs, siRNA1 selectively down-regulated Cdc25B3, rather than Cdc25B2. The other siRNA constructs down-regulated both Cdc25B2 and Cdc25B3 to different degrees. In addition, we performed MTT assays to analyze the effects of the siRNAs on the proliferation of Cdc25B overexpression cell lines. In result, we confirmed that all siRNAs inhibited cell proliferation, although to different degrees (data not shown).
In summary, we confirmed that the Cdc25B overexpression cell lines constructed in this study can be conveniently used as a powerful tool for screening inhibitors of Cdc25B phosphatase and subtype selectivity of Cdc25B siRNA candidates.
Cdc25B phosphatase plays an important role in cell cycle regulation by dephosphorylating its physiological substrate, Cdk1 (Cdc2)/cyclin B.1 Because Cdc25B is overexpressed in many human cancers,6 which leads to excessive activation of its physiological substrate, aberrant cell cycle progression, and poor clinical outcomes, Cdc25B has been actively pursued as an attractive target of cancer drugs for a long time. Thus, valid assay methods for evaluating the activity of Cdc25B are essential to avoid false positive or false negative results when screening anti-cancer drug candidates. However, measuring Cdc25B activity with existing methods is hampered by the use of inadequate substrates of Cdc25B, such as p-nitrophenyl phosphate and 3-O-methylfluorescein phosphate, and the use of nonnative forms of Cdc25B, such as recombinant C-terminal Cdc25B or GST-fused Cdc25B produced in E. coli, thereby yielding less than optimal results.891011 Other methods have also been reported to be inconvenient and have limited accessibility as an experimental tool due to γ-32P labeling of histone H1 by Cdk1, a physiological substrate of Cdc25B. Therefore, in this study, we developed a nonradioisotopic assay method based on Western blotting to properly measure Cdc25B activity using Cdk1. This Western blotting method is easily accessible and avoids the issues with existing methods. This assay is relatively easy and does not require purification of the native form of Cdc25B from human cells. Cdc25B activity can be measured after treating HeLa cells directly with Cdc25B inhibitors, performing immunoprecipitation of cell lysates and Western blotting of immunoprecipitated proteins. As shown in Fig. 2, we optimized the Cdk1 kinase assay by altering various factors, such as the amount of substrate, amount of enzyme, concentration of ATP, and reaction time.
Meanwhile, because Cdc25B can be slightly detected at only the protein level, in this study, we constructed Cdc25B2 and Cdc25B3 overexpression HeLa cell lines to establish a strong assay system for the evaluation of specific Cdc25B inhibitors. Cdc25B2 and Cdc25B3 have oncogenic properties and are the major components of the 5 splice forms of Cdc25B. We confirmed the overexpression of Cdc25B2 and Cdc25B3 through RT-PCR, Western blotting, and two assays for biological activity (Fig. 4). However, we found that the biological activities were lower than expected, considering the levels of RNA and protein expression. These results may be due to the complexity of various negative feedback systems for Cdc25B within cells. For example, MEK1 destabilizes Cdc25B by phosphorylating Ser249, leading to proteasomal degradation of Cdc25B. Activation of Jun N-terminal kinase (JNK) and p38 leads to phosphorylation of Ser101 of Cdc25B, thereby resulting in the rapid degradation of Cdc25B and cell cycle arrest. Centrosome-associated Chk1 negatively regulates Cdc25B through phosphorylation at Ser230 and Ser563. Additionally, 14-3-3 proteins bind with high affinity to the Ser323 residue located near the catalytic site of Cdc25B, disrupting Cdk1 substrate access to the catalytic site.3
As shown in Table 1, vimentin and heat shock cognate 71 kDa protein were only upregulated in the Cdc25B3-induced cell line. This result suggests that Cdc25B2 and Cdc25B3 are regulated by slightly different signaling pathways within the induced cells. Thus, these overexpressed cell lines may not only help to understand the mechanism of action of Cdc25B inhibitors, but also provide useful information for understanding differences in the signaling of Cdc25B variants. Recent studies have reported that the expression patterns of Cdc25B variants differ by cancer type. For example, Cdc25B3, rather than Cdc25B1 or Cdc25B2, was found to be overexpressed in most pancreatic cancer cases and to be associated with poor cancer prognosis.18 Meanwhile, however, overexpression of the Cdc25B2 variant was correlated with tumor grade in colorectal cancer23 and increased tumor progression in non-Hodgkin lymphoma cases.24 This information suggests that a Cdc25B inhibitor as an anticancer agent for a specific cancer type should target a specific Cdc25B variant that is overexpressed therein. In this study, we developed an in vitro dephosphorylation assay using cell-free extracts of Cdc25B overexpression cell lines and showed that the assay can be used to screen for Cdc25B inhibitors under conditions similar to the intracellular environment. The in vitro dephosphorylation assay shown in Fig. 5 indicated that CPD5 specifically inhibited Cdc25B2 but not Cdc25B3. In addition, we found that siRNA1 specifically inhibited Cdc25B3, as shown in Fig. 6B. Therefore, the assays developed herein can be used to discover inhibitors against certain Cdc25B variants.
To summarize the data presented herein, our results show proof of concept that these assay methods can be applied to screen for Cdc25B inhibitors as anticancer drugs. The newly developed system uses Western blotting and Cdc25B overexpression cell lines, with improved specificity and ease of use in comparison with older methods. We expect that this system can be utilized as a screening tool for subtype selectivity of Cdc25B inhibitors, such as small molecule inhibitors and siRNA. In near future, we will further optimize assay conditions that are applicable to high-throughput screening and conduct bioanalytical method validation studies for the development of new pharmaceuticals.
References
1. Boutros R, Lobjois V, Ducommun B. CDC25 phosphatases in cancer cells: key players? Good targets? Nat Rev Cancer. 2007; 7:495–507. PMID: 17568790.


2. Yu L, Orlandi L, Wang P, Orr MS, Senderowicz AM, Sausville EA, et al. UCN-01 abrogates G2 arrest through a Cdc2-dependent pathway that is associated with inactivation of the Wee1Hu kinase and activation of the Cdc25C phosphatase. J Biol Chem. 1998; 273:33455–33464. PMID: 9837924.


3. Sur S, Agrawal DK. Phosphatases and kinases regulating CDC25 activity in the cell cycle: clinical implications of CDC25 overexpression and potential treatment strategies. Mol Cell Biochem. 2016; 416:33–46. PMID: 27038604.


4. Gabrielli BG, De Souza CP, Tonks ID, Clark JM, Hayward NK, Ellem KA. Cytoplasmic accumulation of cdc25B phosphatase in mitosis triggers centrosomal microtubule nucleation in HeLa cells. J Cell Sci. 1996; 109(Pt 5):1081–1093. PMID: 8743955.


5. Lammer C, Wagerer S, Saffrich R, Mertens D, Ansorge W, Hoffmann I. The cdc25B phosphatase is essential for the G2/M phase transition in human cells. J Cell Sci. 1998; 111(Pt 16):2445–2453. PMID: 9683638.


6. Galaktionov K, Lee AK, Eckstein J, Draetta G, Meckler J, Loda M, et al. CDC25 phosphatases as potential human oncogenes. Science. 1995; 269:1575–1577. PMID: 7667636.


7. Takemasa I, Yamamoto H, Sekimoto M, Ohue M, Noura S, Miyake Y, et al. Overexpression of CDC25B phosphatase as a novel marker of poor prognosis of human colorectal carcinoma. Cancer Res. 2000; 60:3043–3050. PMID: 10850455.
8. Honda R, Ohba Y, Nagata A, Okayama H, Yasuda H. Dephosphorylation of human p34cdc2 kinase on both Thr-14 and Tyr-15 by human cdc25B phosphatase. FEBS Lett. 1993; 318:331–334. PMID: 8440392.
9. Gottlin EB, Xu X, Epstein DM, Burke SP, Eckstein JW, Ballou DP, et al. Kinetic analysis of the catalytic domain of human cdc25B. J Biol Chem. 1996; 271:27445–27449. PMID: 8910325.


10. McCain DF, Catrina IE, Hengge AC, Zhang ZY. The catalytic mechanism of Cdc25A phosphatase. J Biol Chem. 2002; 277:11190–11200. PMID: 11805096.


11. Parks JM, Hu H, Rudolph J, Yang W. Mechanism of Cdc25B phosphatase with the small molecule substrate p-nitrophenyl phosphate from QM/MM-MFEP calculations. J Phys Chem B. 2009; 113:5217–5224. PMID: 19301836.


12. Tamura K, Southwick EC, Kerns J, Rosi K, Carr BI, Wilcox C, et al. Cdc25 inhibition and cell cycle arrest by a synthetic thioalkyl vitamin K analogue. Cancer Res. 2000; 60:1317–1325. PMID: 10728693.
13. Hoffmann I, Clarke PR, Marcote MJ, Karsenti E, Draetta G. Phosphorylation and activation of human cdc25-C by cdc2--cyclin B and its involvement in the self-amplification of MPF at mitosis. EMBO J. 1993; 12:53–63. PMID: 8428594.


14. Blomberg I, Hoffmann I. Ectopic expression of Cdc25A accelerates the G(1)/S transition and leads to premature activation of cyclin E- and cyclin A-dependent kinases. Mol Cell Biol. 1999; 19:6183–6194. PMID: 10454565.
15. Rubin SM. Deciphering the retinoblastoma protein phosphorylation code. Trends Biochem Sci. 2013; 38:12–19. PMID: 23218751.


16. Blewett A. Cell division: CDK1 in the driving seat. Nat Rev Mol Cell Biol. 2007; 8:755–756.
17. Cossy J, Belotti D, Brisson M, Skoko JJ, Wipf P, Lazo JS. Biological evaluation of newly synthesized quinoline-5,8-quinones as Cdc25B inhibitors. Bioorg Med Chem. 2006; 14:6283–6287. PMID: 16782352.


18. Forrest AR, McCormack AK, DeSouza CP, Sinnamon JM, Tonks ID, Hayward NK, et al. Multiple splicing variants of cdc25B regulate G2/M progression. Biochem Biophys Res Commun. 1999; 260:510–515. PMID: 10403798.


19. Sambrook J, Fritsch EF, Maniatis T. Molecular cloning: a laboratory manual. 2nd ed. Cold Spring Harbor (NY): Cold Spring Harbor Laboratory Press;1989.
20. Morgan DL. The cell cycle: principles of control. London: New Science Press;2007.
21. Münger K, Howley PM. Human papillomavirus immortalization and transformation functions. Virus Res. 2002; 89:213–228. PMID: 12445661.


22. Freedman BS, Heald R. Functional comparison of H1 histones in Xenopus reveals isoform-specific regulation by Cdk1 and RanGTP. Curr Biol. 2010; 20:1048–1052. PMID: 20471264.


23. Talvinen K, Tuikkala J, Grönroos J, Huhtinen H, Kronqvist P, Aittokallio T, et al. Biochemical and clinical approaches in evaluating the prognosis of colon cancer. Anticancer Res. 2006; 26:4745–4751. PMID: 17214335.
24. Hernández S, Hernández L, Bea S, Pinyol M, Nayach I, Bellosillo B, et al. cdc25a and the splicing variant cdc25b2, but not cdc25B1, -B3 or -C, are over-expressed in aggressive human non-Hodgkin's lymphomas. Int J Cancer. 2000; 89:148–152. PMID: 10754492.


25. Garzon RJ, Zehner ZE. Multiple silencer elements are involved in regulating the chicken vimentin gene. Mol Cell Biol. 1994; 14:934–943. PMID: 8289833.


26. Leader M, Collins M, Patel J, Henry K. Vimentin: an evaluation of its role as a tumour marker. Histopathology. 1987; 11:63–72. PMID: 2435649.


27. Bomsztyk K, Denisenko O, Ostrowski J. hnRNP K: one protein multiple processes. Bioessays. 2004; 26:629–638. PMID: 15170860.


28. Mayer MP, Bukau B. Hsp70 chaperones: cellular functions and molecular mechanism. Cell Mol Life Sci. 2005; 62:670–684. PMID: 15770419.


29. Wang X, Wang Q, Lin H, Li S, Sun L, Yang Y. HSP72 and gp96 in gastroenterological cancers. Clin Chim Acta. 2013; 417:73–79. PMID: 23266770.


30. Song Y, Luo Q, Long H, Hu Z, Que T, Zhang X, et al. Alpha-enolase as a potential cancer prognostic marker promotes cell growth, migration, and invasion in glioma. Mol Cancer. 2014; 13:65. PMID: 24650096.


31. Zhou W, Capello M, Fredolini C, Piemonti L, Liotta LA, Novelli F, et al. Mass spectrometry analysis of the post-translational modifications of alpha-enolase from pancreatic ductal adenocarcinoma cells. J Proteome Res. 2010; 9:2929–2936. PMID: 20433201.
32. Sharma MC, Sharma M. The role of annexin II in angiogenesis and tumor progression: a potential therapeutic target. Curr Pharm Des. 2007; 13:3568–3575. PMID: 18220793.


33. Thweatt R, Lumpkin CK Jr, Goldstein S. A novel gene encoding a smooth muscle protein is overexpressed in senescent human fibroblasts. Biochem Biophys Res Commun. 1992; 187:1–7. PMID: 1520290.


Fig. 1
A nonradioisotopic assay method to measure Cdk1 kinase activity. We used two kinds of Cdk1 kinases, one was Cdk1 IP and the other was commercially available Cdk1. The Cdk1 IP was prepared from HeLa cell extracts treated with 250 nM nocodazole, and the commercial Cdk1 kinase was purchased. These two kinds of Cdk1 were used to measure Cdk1 kinase activity in the presence (lanes 2, 6) or absence (lanes 1, 3, 4, 5, 7, 8) of 1 mM olomoucine (a Cdk1 kinase inhibitor) using Western blotting with 1 μg of Rb (A) or 2 μg of histone H1 (B) as a substrate. The Cdk1 kinase activity was determined by phosphorylation levels of each substrate using the anti-pSer795 Rb antibody or anti-pThr/Pro motif-recognizing antibody, respectively. Cdk1, cyclin-dependent kinase 1; Rb, retinoblastoma protein; IP, immunoprecipitates.

Fig. 2
Optimization of several conditions for the Cdk1 kinase assay. Several key factors were examined to optimize the conditions of the Cdk1 kinase assay. The basic procedure of the reaction was described in the Materials and Methods section with the exception of the indicated protein amounts from HeLa cell extracts to obtain Cdk1 IP, substrate amounts, ATP concentrations, and reaction times using Rb or histone H1 as a substrate. All examined parameters were positively correlated with Cdk1 kinase activity, except for the excess concentration of ATP. Cdk1, cyclin-dependent kinase 1; Rb, retinoblastoma protein; IP, immunoprecipitates.
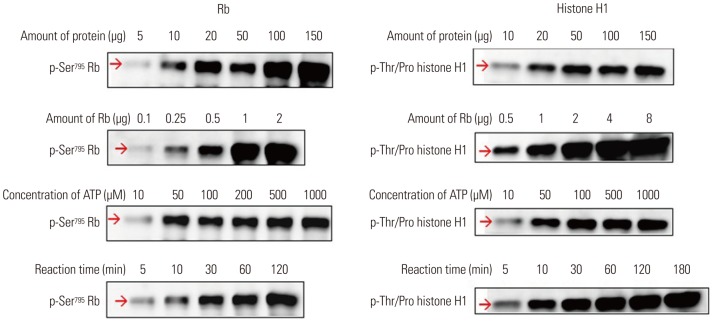
Fig. 3
Cdk1 kinase assay as a tool for assessing Cdc25B activity. To determine if the Cdk1 kinase assay can measure Cdc25B activity by treating cells directly with Cdc25B inhibitors, we performed the following tests: (A) FACS analysis results for HeLa cells treated with 0.1% DMSO as a negative control, 10 μM CPD5 as a Cdc25B inhibitor, or 250 nM nocodazole are shown in (A). Cdk1 IPs derived from HeLa cells treated with DMSO (lane 1), CPD5 (lane 2), or nocodazole (lane 3) were used to conduct Western blot analysis (B) and Cdk1 kinase assay (C). Total protein extractions, Western blotting, and Cdk1 kinase reactions were performed as described in the Materials and Methods. Cdk1, cyclin-dependent kinase 1; Rb, retinoblastoma protein; IP, immunoprecipitates; CPD5, compound 5.
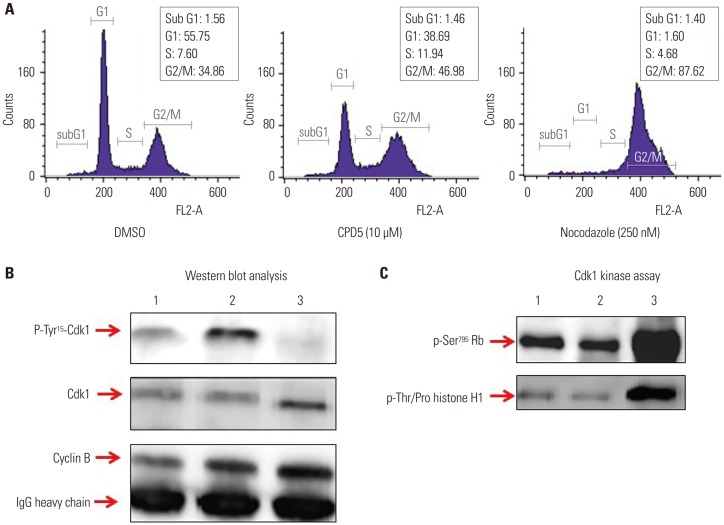
Fig. 4
Construction and characterization of Cdc25B2 and Cdc25B3 overexpression cell lines. We constructed stable Cdc25B2 or Cdc25B3 overexpression HeLa cell lines under the control of tetracycline to establish a strong assay system, and the cell lines were characterized by transcript levels (A), protein levels (B), and biological activity levels (C and D). (A) RT-PCR, (B) Western blotting. M: Size Marker, Lane 1: Cdc25B2 not induced cell line, Lane 2: Cdc25B2-induced cell line, Lane 3: Cdc25B3 not induced cell line, Lane 4: Cdc25B3-induced cell line. (C) Biological activity of the Cdc25B2 overexpression cell line was confirmed by FACS analysis. The cell line was arrested at G2 phase after 24 hours of treatment with 800 nM VP-16 (etoposide). After 16 hours of treatment with tetracycline, the cell cycle was analyzed by FACS. (D) Biological activity in the Cdc25B2 overexpression cell line was confirmed by the Cdk1 activity assay using Cdk1 IP. Lane 1: Cdc25B2-induced cell line, Lane 2: Cdc25B2 not induced cell line. Cdk1, cyclin-dependent kinase 1; Rb, retinoblastoma protein; IP, immunoprecipitates.
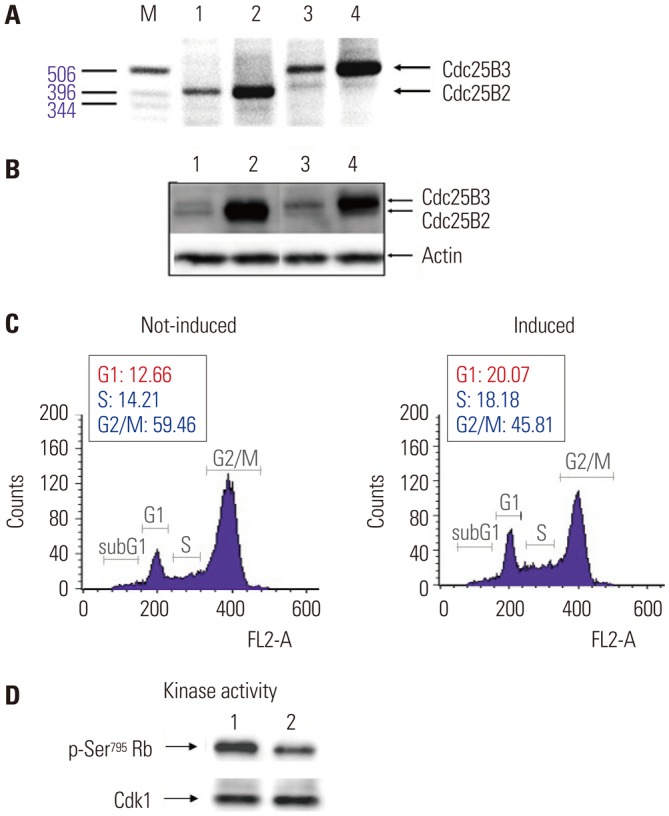
Fig. 5
In vitro Cdk1 dephosphorylation assay using Cdc25B overexpression cell lines. To develop a simple screening tool for Cdc25B inhibitors under conditions similar to an intracellular environment at the in vitro level, we used cell-free extracts depleted of Cdk1 and cyclin B from Cdc25B2 or Cdc25B3 overexpression HeLa cells. (A and B) Phospho-Cdk1 as a substrate was prepared by immunoprecipitation with a monoclonal Cdk1 antibody from HeLa cell extracts treated with CPD5. The dephosphorylation reaction was performed as described in the Materials and Methods section. Cdk1 IPs were incubated with buffer only (lane 2), cell-free extract depleted of Cdk1 and cyclin B (lane 3) in the presence of 0.1% DMSO as a negative control (lane 5), phosphatase inhibitors (5 mM β-glycerophosphate and 0.1 mM Na3VO4) as a positive control (lane 4), or 10 μM CPD5 in the presence of 0.1% DMSO (lane 6). Lane 1 includes cell-free extract alone. Cdk1, cyclin-dependent kinase 1; IP, immunoprecipitates; CPD5, compound 5.
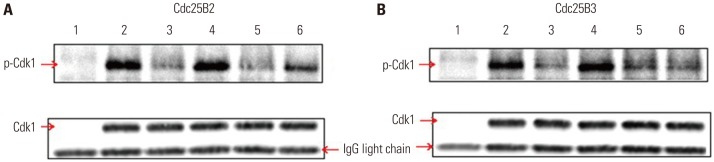
Fig. 6
siRNA screening assay using Cdc25B overexpression cell lines. To examine whether Cdc25B overexpression HeLa cell lines could be used as a siRNA screening tool, we performed the following: (A) The CDC25B2 and CDC25B3 genes are identical, except that exon 4 of CDC25B2 consists of only a portion of the sequence of exon 4 of CDC25B3. Four siRNAs were designed to inhibit Cdc25B2 or Cdc25B3 expression selectively, and each sequence is presented. (B) The inhibition effects of the siRNAs were measured by Western blotting. After siRNA transfection of Cdc25B2 or Cdc25B3 overexpression cell lines, the expression level of each protein was analyzed with anti-Cdc25B and anti-β actin antibodies. Lane 1: not induced cell line, Lane 2: induced cell line, Lane 3: induced cell line transfected with siRNA1, Lane 4: siRNA2, Lane 5: scrambled siRNA, Lane 6: siRNA3, Lane 7: siRNA4, Lane 8: scrambled siRNA. siRNA, small interfering RNA.
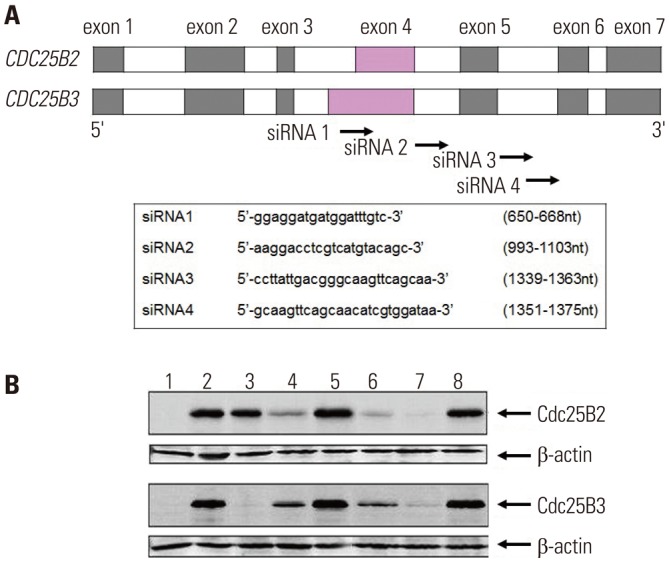
Table 1
Differentially Expressed or PTM Proteins in Cdc25B Overexpression Cell Lines
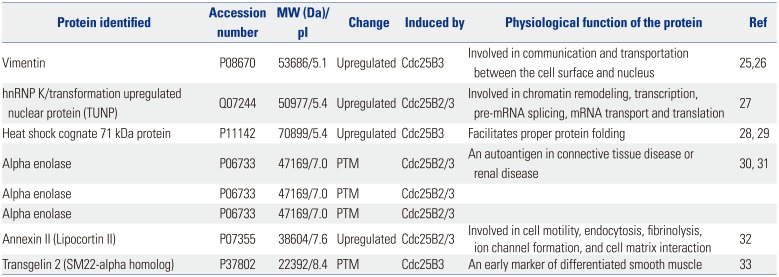
Protein identified | Accession number | MW (Da)/pI | Change | Induced by | Physiological function of the protein | Ref |
---|---|---|---|---|---|---|
Vimentin | P08670 | 53686/5.1 | Upregulated | Cdc25B3 | Involved in communication and transportation between the cell surface and nucleus | 2526 |
hnRNP K/transformation upregulated nuclear protein (TUNP) | Q07244 | 50977/5.4 | Upregulated | Cdc25B2/3 | Involved in chromatin remodeling, transcription, pre-mRNA splicing, mRNA transport and translation | 27 |
Heat shock cognate 71 kDa protein | P11142 | 70899/5.4 | Upregulated | Cdc25B3 | Facilitates proper protein folding | 2829 |
Alpha enolase | P06733 | 47169/7.0 | PTM | Cdc25B2/3 | An autoantigen in connective tissue disease or renal disease | 3031 |
Alpha enolase | P06733 | 47169/7.0 | PTM | Cdc25B2/3 | ||
Alpha enolase | P06733 | 47169/7.0 | PTM | Cdc25B2/3 | ||
Annexin II (Lipocortin II) | P07355 | 38604/7.6 | Upregulated | Cdc25B2/3 | Involved in cell motility, endocytosis, fibrinolysis, ion channel formation, and cell matrix interaction | 32 |
Transgelin 2 (SM22-alpha homolog) | P37802 | 22392/8.4 | PTM | Cdc25B3 | An early marker of differentiated smooth muscle | 33 |