Abstract
Purpose
Hydrogen sulfide (H2S) is an endogenous gaseous molecule with important physiological roles. It is synthesized from cysteine by cystathionine γ-lyase (CGL) and cystathionine β-synthase (CBS). The present study examined the benefits of exogenous H2S on renal ischemia reperfusion (IR) injury, as well as the effects of CGL or CBS inhibition. Furthermore, we elucidated the mechanism underlying the action of H2S in the kidneys.
Materials and Methods
Thirty male Sprague-Dawley rats were randomly assigned to five groups: a sham, renal IR control, sodium hydrosulfide (NaHS) treatment, H2S donor, and CGL or CBS inhibitor administration group. Levels of blood urea nitrogen (BUN), serum creatinine (Cr), renal tissue malondialdehyde (MDA), and superoxide dismutase (SOD) were estimated. Histological changes, apoptosis, and expression of mitogen-activated protein kinase (MAPK) family members (extracellular signal-regulated kinase, c-Jun N-terminal kinase, and p38) were also evaluated.
Results
NaHS attenuated serum BUN and Cr levels, as well as histological damage caused by renal IR injury. Administration of NaHS also reduced oxidative stress as evident from decreased MDA, preserved SOD, and reduced apoptotic cells. Additionally, NaHS prevented renal IR-induced MAPK phosphorylation. The CGL or CBS group showed increased MAPK family activity; however, there was no significant difference in the IR control group.
Renal ischemia reperfusion (IR) injury is an important clinical condition and causes significant morbidity and mortality during clinical procedures, such as kidney transplantation, partial nephrectomy, and hemorrhagic shock. Among various pathophysiological mechanisms of IR injury, oxidative stress plays a key role in inducing cellular and tissue damage. Excessive production of reactive oxygen species (ROS) and suppression of anti-oxidant defense system have been found to contribute to IR insult in many organs. Furthermore, ROS can activate the mitogen-activated protein kinase (MAPK) pathway, which plays a pivotal role in signal transduction during IR injury.12 Three distinct subgroups of MAPK, including extracellular signal-regulated kinase (ERK), c-Jun N-terminal kinase (JNK), and p38, are involved in cell recovery, survival, and death during IR injury.34
Gaseous transmitters have been implicated in the regulation of multiple pathophysiological functions in mammalian tissues.5 Along with nitric oxide (NO) and carbon monoxide (CO), hydrogen sulfide (H2S) has been characterized as a gaseous bioactive substance in various body systems. Recently, H2S has emerged as an endogenous molecule with important physiological roles. The cytoprotective or beneficial effects of the H2S donor sodium hydrosulfide (NaHS) against myocardial infarction, gastric ulcer, hypertension, and IR injury have been observed in several animal models.678
H2S is synthesized from cysteine by cystathionine γ-lyase (CGL), cystathionine β-synthase (CBS), and 3-mercaptopyruvate sulfur transferase (3-MST). Although all of these enzymes are required for H2S production, CGL and CBS are the major enzymes.9 These two enzymes are expressed in a variety of tissues and organs, including the kidney, liver, central nervous system, and vascular system.1011 The kidney is the important organ for endogenous H2S production by CGL and CBS.12 Inhibition of CGL and CBS, using D,L-propargylglycine (PAG) and amino-oxyacetic acid (AOAA), respectively, is a common strategy for investigating the effect of endogenous H2S on a disease.
In this study, we investigated whether exogenous H2S protects against renal IR injury, and the effects of inhibition of CGL or CBS were assessed to determine the relative contribution of both enzymes on H2S production in renal IR injury. Moreover, we intended to elucidate the underlying mechanism of action of H2S in the kidneys through the MAPK family.
All experiments were performed on male Sprague-Dawley rats (280–300 g), which were obtained from Central Laboratories (Seoul, Korea). All protocols were approved by the Institutional Animal Care and Use Committee at Kyungpook National University (2015-0029), Daegu, Korea.
The rats were randomly assigned to one of five groups (n=5 per group). Group 1 was the sham group (sham); the rats underwent a sham operation without the renal IR procedure. Group 2 was the renal IR control group (IR control); the rats underwent the renal IR procedure. Group 3 was the H2S donor administration group (NaHS); the rats received 100 µmol/kg NaHS by intraperitoneal (i.p.) injection 30 min before the renal IR procedure. Group 4 was the AOAA administration group (AOAA); the rats received 10 mg/kg AOAA by i.p. injection 30 min before the renal IR procedure. Group 5 was the PAG administration group (PAG); the rats received 50 mg/kg of PAG by i.p. injection 30 min before the renal IR procedure. The dose of each drug was selected based on previous studies.131415 Rats were anesthetized with ketamine (60 mg/kg, i.p.) and xylene (10 mg/kg, i.p.) prior to the renal IR procedure. To perform the renal IR procedure, first, the right nephrectomy was carried out. Next, the left kidney vasculature was clamped for 30 min using a nontraumatic vascular clamp. The clamp was then removed to allow reperfusion. After reperfusion for 24 h, blood samples were collected from the heart and centrifuged at 3000 rpm for 15 min for analysis of blood urea nitrogen (BUN) and creatinine (Cr) levels. A portion of the harvested renal tissues was stored in liquid nitrogen at −80℃ for the analysis of malondialdehyde (MDA), superoxide dismutase (SOD), and MAPK family protein expression. The remaining tissues were processed for histopathological examination.
To assess renal functions, serum BUN and Cr levels were analyzed using the colorimetric assay according to the manufacturer's protocols (Asan Pharmacy, Seoul, Korea). MDA level in the renal tissue was quantified using thiobarbituric acid reactive substances, and the absorbance of the reaction mixture was measured at 535 nm. The levels were expressed as nmol/g of tissue, according to a standard curve.16 SOD activity in the renal tissue was assessed using the pyrogallol autoxidation method, and the absorbance was measured at 420 nm.17 SOD activity was calculated as U/mg of protein.
Renal tissues were homogenized using a glass homogenizer (Kontes Glass Co, Vineland, NJ, USA) in RIPA buffer (Thermo Scientific, Waltham, MA, USA) containing protease and a phosphatase inhibitor cocktail (Thermo Scientific). The samples were centrifuged at 12500 rpm for 20 min at 4℃ followed by separation of the supernatant. Total protein was quantified using the Bradford assay (Thermo Scientific). The samples were mixed with loading buffer solution [60 mM Tris-HCl, 25% glycerol, 2% sodium dodecyl sulfate (SDS), 14.4 mM 2-mercaptoethanol, and 0.1% bromophenol blue], separated on a 10% SDS polyacrylamide gel, and transferred to a nitrocellulose membrane (Whatman GmbH, Dassel, Germany). The membrane was blocked with 1% bovine serum albumin in Tris-buffered saline containing 0.1% Tween-20 (TBS-T) for 1 h at room temperature. Following washes, the blots were incubated overnight at 4℃ with antibodies against p-JNK, p-ERK, p-p38 (diluted 1:1000; Santa Cruz, CA, USA), and β-actin (diluted 1:5000; Cell Signaling, Beverly, MA, USA). Membranes were then incubated with horseradish peroxidase-conjugated secondary antibody (diluted 1:1000; Cell Signaling Technology), developed using the enhanced chemiluminescence substrate kit (Advansta, Menlo Park, CA, USA), and exposed onto Agfa medical X-ray film (Agfa-Gevaert Group, Mortsel, Belgium). The signal intensity was quantified using the NIH Image J 1.47v software (National Institutes of Health, Bethesda, MD, USA).
Renal tissues were immediately perfused in 10% phosphate-buffered formalin, embedded in paraffin, sectioned to a 4-micrometer thickness, and stained with periodic acid Schiff (PAS). The sections were evaluated under a light microscope at 200× magnification, and five fields per slide were scored by tubular cell form, luminal state, and cast formation in a blind fashion. They were then graded on a scale of 0 to 3 (0: normal; 1: minimal; 2: moderate; 3: severe) by a renal pathologist in an unbiased fashion.18
The terminal deoxynucleotidyl transferase dUTP nick-end labeling (TUNEL) assay was performed as an index of cell injury using a detection kit (Fluorescein, Roche, Mannheim, Germany) according to the manufacturer's instructions. In brief, kidney sections were incubated with TUNEL reagent mixture, followed by washing with phosphate-buffered saline. 4,6-diamidino-2-phenylindole (DAPI) was used for nuclei staining. Images of TUNEL staining were collected from the outer medulla of kidneys using a microscope at 400× magnification (Leica DM2500). TUNEL-positive cells were counted in an unbiased fashion in five random fields per kidney sample under a light microscope with 400× magnification.
Data analysis was performed using SPSS software (Version 18.0 for Windows; SPSS Inc., Chicago, IL, USA). Data are expressed as a mean±SEM. The Mann-Whitney U test was used to assess the appropriateness of renal IR injury between the sham group and the IR control group. After performing the Kruskal-Wallis test to detect differences among the four groups, we applied the Mann-Whitney U post hoc test, each with Bonferroni correction, for the three pairwise comparison of the renal IR group with the other treatment groups. Differences between the groups were considered significant at p<0.05.
Induction of renal ischemia (30 min) followed by reperfusion (24 h) resulted in a significant elevation of serum Cr and BUN levels, compared to those in the sham group (p=0.008 for both). Administration of NaHS significantly attenuated this renal IR-induced elevation of serum Cr and BUN levels (p=0.024 for both). In the AOAA and PAG groups, serum Cr and BUN levels did not show any significant difference, compared to those in the IR control group (Fig. 1).
MDA levels in the IR control group were significantly increased, compared to those in the sham group (p=0.008). Administration of NaHS significantly attenuated the elevation of MDA levels caused by renal IR injury (p=0.024). SOD levels in the IR control group were significantly lower than those in the sham group (p=0.008). Administration of NaHS significantly preserved SOD levels, compared to those in the IR control group (p=0.024). SOD levels were also preserved in the AOAA and PAG groups, compared to those in the IR control group (p=0.024 for both) (Fig. 2).
Renal IR promoted phosphorylation of ERK, JNK, and p38 (p=0.008 for all). NaHS significantly inhibited renal IR-induced activation of MAPK pathways (p=0.024 for all). Phosphorylation of ERK was decreased in the PAG group, compared to that in the IR control group (p=0.024). Rats in the AOAA and PAG groups showed increased levels of phosphorylated ERK, JNK, and p38, similar to those observed in the renal IR control group (Fig. 3).
Analysis of tissue sections from the kidneys by PAS staining demonstrated a significant tissue damage in the renal IR control group (p=0.008). This was manifested by tubular dilatation, luminal congestion, and tubular cell atrophy. In the NaHS group, kidney structures appeared better, with mild tubular dilatation and luminal congestion (p=0.024). Kidney structures in the AOAA and PAG groups were also better, compared to those in the IR control group (for AOAA, p=0.048; for PAG, p=0.024) (Fig. 4).
Kidney sections from the IR control group showed increased TUNEL-positive cells, compared with those from the sham group (p=0.008). The degree of apoptosis in the NaHS group was significantly decreased, compared to that in the IR control group (p=0.024). Rats in the AOAA and PAG groups exhibited decreased TUNEL-positive cells, compared with those in the IR control group (for AOAA, p=0.024; for PAG, p=0.024) (Fig. 5).
This study demonstrates that exogenous H2S can attenuate renal IR injury. Its renoprotective effects were characterized not only by decreased serum BUN and Cr levels but also by attenuated histological damage that includes apoptosis caused by renal IR injury. Administration of NaHS in rats decreased MDA levels and preserved SOD levels, indicating reduced lipid peroxidation and preserved antioxidant enzymes, respectively. In addition, NaHS prevented renal IR-induced phosphorylation of MAPK family proteins (ERK, JNK, and p38). In the AOAA and PAG groups, renal function indices and MAPK phosphorylation levels did not change, compared to those in the renal IR control group, indicating that CGL or CBS inhibition had no effect on renal impairment of rats subjected to renal IR injury.
H2S has been identified as an important gaseous transmitter for molecular therapy in renal IR injury.1319 It was initially known as an environmentally toxic molecule; however, it is now recognized as a physiological molecule and third gasotransmitter, after NO and CO, in the mammalian body. Gasotransmitters exhibit various biological functions, including vasodilatation, angiogenesis, metabolic modulation, anti-oxidant activity, anti-inflammation, and anti-apoptotic activity, in various animal models.2021 Among many physiological and cytoprotective actions of H2S, one of the main mechanisms underlying H2S renoprotection is its anti-oxidative role. Several previous studies have demonstrated that H2S upregulates anti-oxidant defense, particularly under severe chronic conditions.2223 Moreover, H2S functions as a direct scavenger of ROS, thus preventing IR injury.2425 In this study, NaHS-treated rats exhibited significantly decreased serum BUN and Cr levels and improved renal tissue damage, compared to those in the IR control group. In addition, NaHS-treated rats exhibited decreased MDA levels, demonstrating its cytoprotective effect against oxidative stress. Increased SOD levels suggested that NaHS maintains the intracellular anti-oxidant capacity of kidney cells. Additionally, TUNEL-positive cells were decreased in the NaHS group, suggesting that NaHS offered a protective effect against renal tubular apoptosis. During IR injury, increased ROS initiates transition of mitochondrial permeability, which in turn induces pro-apoptotic molecules and stimulates the formation of various inflammatory cytokines.26 Therefore, decreased MDA and preserved SOD levels, along with reduced apoptosis, in renal cells might underlie the protective effect of NaHS on oxidative stress.
Three catalytic enzymes, CGL, CBS, and 3-MST, are associated with the production of endogenous H2S. CGL and CBS are primarily located on renal proximal tubules, and these are the main enzymes for H2S generation in the kidney.27 Kidney IR injury induces tubular epithelial cell damage, indicating that this might lead to impaired production of H2S. Han, et al.28 showed that the production of H2S was decreased in renal IR injury. Thus, H2S donor, NaHS, exerted the protective effects on the recovery from kidney damage.1328 CGL and CBS activities were reduced in various renal IR injury studies,1228 and inhibition of either enzyme by pharmacological inhibitors aggravated renal damage.1328 However, Roy, et al.29 showed that the inhibition of either of the enzymes (CGL or CBS) had no significant effect on the vascular system, whereas simultaneous inhibition of both the enzymes reduced H2S production. This is consistent with our results, which suggested that suppression of either CGL or CBS did not have any effect on renal IR. Therefore, we suspect that these two enzymes may have an interdependent regulation or that they may complement each other in maintaining the production of H2S. A recent study by Cortese-Krott, et al.30 demonstrated that the H2S and NO signaling pathways intertwine with coupled chemical reactions to generate three products, nitrosopersulfide, dinitrososulfide, and polysulfides, each with a specific chemical bioactivity. In this study, considering the response of both the inhibitors, we assume that some unknown interactions between H2S and other molecules, such as NO, an important mediator of IR injury, may affect the action of CGL or CBS inhibitors during renal IR injury. In addition, in the CGL or CBS group, there were fewer histological changes, compared to the IR injury control group, while there were no differences in the MDA levels. This discrepancy might be attributed to the nature of the reaction in histologic pathogenesis, such as inflammatory response, neutrophil activation, and angiogenesis. Meanwhile, anti-oxidant activity (SOD) was increased in the CGL or CBS group, which might have affect mild histological changes therein. Therefore, the exact role of these enzymes in renal IR injury is unclear, and further investigations will be needed in future.
Oxidative stress by IR injury can activate MAPK signaling. The MAPK signaling pathway play a central role in transferring extracellular signals to the nucleus.1 Among three MAPK subgroups, ERK is generally activated by growth factors and contributes to cell survival. Conversely, JNK and p38 are preferentially activated by environmental stress and contribute to cell death.3132 However, these roles are not always distinctive, and cell fate associated with MAPK pathways may be determined by the nature of the environmental stress, activation duration, cell type, or the activities of other signaling pathways.3334 The influence of MAPK on the cytoprotective effect of H2S has been extensively studied. The neuroprotective effect of H2S has been reported to be involved in the suppression of ERK activation.35 Further, activation of ERK, JNK, and p38 has been shown to be associated with chemical hypoxia-induced neuronal injury and with H2S-protected neuronal injuries via ROS-activated ERK and p38 MAPK signaling pathways.36 In addition, NaHS treatment has been found to attenuate the phosphorylation of ERK, JNK, and p38 in renal IR injury.18 Here, we confirm that renal ischemia for 30 min and reperfusion for 24 h results in activation of ERK, JNK, and p38. Above all, we have shown that these effects are reduced by exogenous H2S treatment.
The present study has certain limitations. Here, we focused only on oxidative stress in renal IR injury. In addition to oxidative stress, several other events, such as inflammatory responses and neutrophil activation, have also been implicated in the pathognomonic process. Furthermore, we tried to identify the relative contribution of the enzymes involved in H2S production by individual inhibition of AOAA or PAG; however, combined inhibition of both should also be investigated to reach a definite conclusion on H2S production.
In summary, we have demonstrated that exogenous H2S mitigates renal damage caused by IR injury. In addition, its proposed beneficial effect is, in part, due to anti-oxidative stress mediated via modulation of MAPK signaling. Therefore, the present study suggests that exogenous H2S may be beneficial in a variety of conditions associated with renal IR injury.
Figures and Tables
Fig. 1
Changes in renal function. (A and B) The IR control group showed a significant increase in serum Cr and BUN levels, compared to those in the sham group. Rats treated with NaHS showed lower serum Cr and BUN levels, compared with those in the IR control group. The results are expressed as a mean±SEM (n=5 rats/group). *p<0.05 vs. the sham group, †p<0.05 vs. the IR control group. IR, ischemia reperfusion; Cr, creatinine; BUN, blood urea nitrogen; NaHS, sodium hydrosulfide; AOAA, amino-oxyacetic acid; PAG, D,L-propargylglycine.
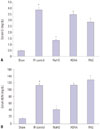
Fig. 2
Changes in renal oxidative stress. (A) The IR group showed a significant increase in MDA levels, compared to those in the sham group. MDA levels in the NaHS group were attenuated, compared with those in the IR control group. (B) The IR group showed a significant decrease in SOD levels, compared to those in the sham group. SOD levels in the NaHS group were preserved, compared with those in the IR group. SOD levels were also preserved in the AOAA and PAG groups, compared to those in the IR control group. The results are expressed as a mean±SEM (n=5 rats/group). *p<0.05 vs. the sham group, †p<0.05 vs. the IR control group. IR, ischemia reperfuion; MDA, malondialdehyde; SOD, superoxide dismutase; NaHS, sodium hydrosulfide; AOAA, amino-oxyacetic acid; PAG, D,L-propargylglycine.
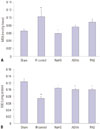
Fig. 3
Changes in renal MAPKs. Western blotting was performed to measure (A) phosphorylated ERK, (B) phosphorylated JNK, and (C) phosphorylated p38 expression levels in kidney cell homogenates. Renal IR injury promoted phosphorylation of ERK, JNK, and p38. NaHS significantly inhibited renal IR-induced activation of MAPK pathways. The results are expressed as a mean±SEM (n=5 rats/group). *p<0.05 vs. the sham group, †p<0.05 vs. the IR control group. MAPK, mitogen-activated protein kinase; ERK, extracellular signal-regulated kinase; JNK, c-Jun N-terminal kinase; IR, ischemia reperfusion; NaHS, sodium hydrosulfide; AOAA, amino-oxyacetic acid; PAG, D,L-propargylglycine.
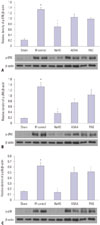
Fig. 4
Histopathological examination of the renal tissues from the experimental groups (A) and damage score (B). Rats in the IR control group exhibited tubular dilatation and luminal congestion (thick arrow) along with tubular cell atrophy (thin arrow). In the NaHS group, kidney structures were preserved, with mild tubular dilatation and luminal congestion. Kidney structures in the AOAA and PAG group appeared better, compared with those from the IR control group. Tissue sections were stained with periodic acid Schiff stain and visualized at magnification ×200. The results are expressed as a mean±SEM (n=5 rats/group). *p<0.05 vs. the sham group, †p<0.05 vs. the IR control group. IR, ischemia reperfusion; NaHS, sodium hydrosulfide; AOAA, amino-oxyacetic acid; PAG, D,L-propargylglycine.
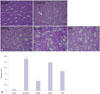
Fig. 5
Apoptosis evaluation (representative images and quantitative analyses of TUNEL-positive cells) in renal tissue from the experimental groups. (A and B) Kidney sections from the IR control group showed increased TUNEL-positive cells, compared with those from the sham group. The degree of apoptosis in the NaHS group was significantly decreased, compared with those in the IR control group. Rats in the AOAA and PAG group exhibited decreased TUNEL-positive cells, compared with those in the IR control group. The results are expressed as a mean±SEM (n=5 rats/group, magnification ×400). *p<0.05 vs. the sham group, †p<0.05 vs. the IR control group. TUNEL, transferase dUTP nick-end labeling; IR, ischemia reperfusion; NaHS, sodium hydrosulfide; AOAA, amino-oxyacetic acid; PAG, D,L-propargylglycine.
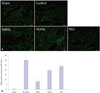
ACKNOWLEDGEMENTS
This work was supported by Biomedical Research Institute grant, Kyungpook National University Hospital (2015).
References
1. Son Y, Cheong YK, Kim NH, Chung HT, Kang DG, Pae HO. Mitogen-activated protein kinases and reactive oxygen species: how can ROS activate MAPK pathways? J Signal Transduct. 2011; 2011:792639.


2. Ferrandi C, Ballerio R, Gaillard P, Giachetti C, Carboni S, Vitte PA, et al. Inhibition of c-Jun N-terminal kinase decreases cardiomyocyte apoptosis and infarct size after myocardial ischemia and reperfusion in anaesthetized rats. Br J Pharmacol. 2004; 142:953–960.


3. Li J, Miller EJ, Ninomiya-Tsuji J, Russell RR 3rd, Young LH. AMP-activated protein kinase activates p38 mitogen-activated protein kinase by increasing recruitment of p38 MAPK to TAB1 in the ischemic heart. Circ Res. 2005; 97:872–879.


4. Yuan L, Wang J, Xiao H, Wu W, Wang Y, Liu X. MAPK signaling pathways regulate mitochondrial-mediated apoptosis induced by isoorientin in human hepatoblastoma cancer cells. Food Chem Toxicol. 2013; 53:62–68.


5. Wang R. Two's company, three's a crowd: can H2S be the third endogenous gaseous transmitter? FASEB J. 2002; 16:1792–1798.


6. Elrod JW, Calvert JW, Morrison J, Doeller JE, Kraus DW, Tao L, et al. Hydrogen sulfide attenuates myocardial ischemia-reperfusion injury by preservation of mitochondrial function. Proc Natl Acad Sci U S A. 2007; 104:15560–15565.


7. Guo C, Liang F, Shah Masood W, Yan X. Hydrogen sulfide protected gastric epithelial cell from ischemia/reperfusion injury by Keap1 s-sulfhydration, MAPK dependent anti-apoptosis and NF-κB dependent anti-inflammation pathway. Eur J Pharmacol. 2014; 725:70–78.


8. Yan H, Du JB, Tang CS. [Possible effects of endogenous hydrogen sulfide on aortic remodeling in hypertensive rats]. Beijing Da Xue Xue Bao Yi Xue Ban. 2004; 36:234–237.
9. Boehning D, Snyder SH. Novel neural modulators. Annu Rev Neurosci. 2003; 26:105–131.
10. Stipanuk MH, Beck PW. Characterization of the enzymic capacity for cysteine desulphhydration in liver and kidney of the rat. Biochem J. 1982; 206:267–277.


11. Wang R. Hydrogen sulfide: the third gasotransmitter in biology and medicine. Antioxid Redox Signal. 2010; 12:1061–1064.


12. Xu Z, Prathapasinghe G, Wu N, Hwang SY, Siow YL, Karmin O. Ischemia-reperfusion reduces cystathionine-beta-synthase-mediated hydrogen sulfide generation in the kidney. Am J Physiol Renal Physiol. 2009; 297:F27–F35.
13. Tripatara P, Patel NS, Collino M, Gallicchio M, Kieswich J, Castiglia S, et al. Generation of endogenous hydrogen sulfide by cystathionine gamma-lyase limits renal ischemia/reperfusion injury and dysfunction. Lab Invest. 2008; 88:1038–1048.


14. Kang K, Zhao M, Jiang H, Tan G, Pan S, Sun X. Role of hydrogen sulfide in hepatic ischemia-reperfusion-induced injury in rats. Liver Transpl. 2009; 15:1306–1314.


15. Wang P, Isaak CK, Siow YL, Karmin O. Downregulation of cystathionine β-synthase and cystathionine γ-lyase expression stimulates inflammation in kidney ischemia-reperfusion injury. Physiol Rep. 2014; 2:e12251.
16. Mihara M, Uchiyama M. Determination of malonaldehyde precursor in tissues by thiobarbituric acid test. Anal Biochem. 1978; 86:271–278.


17. Marklund S, Marklund G. Involvement of the superoxide anion radical in the autoxidation of pyrogallol and a convenient assay for superoxide dismutase. Eur J Biochem. 1974; 47:469–474.


18. Kim J, Park JW, Park KM. Increased superoxide formation induced by irradiation preconditioning triggers kidney resistance to ischemia-reperfusion injury in mice. Am J Physiol Renal Physiol. 2009; 296:F1202–F1211.


19. Bos EM, Leuvenink HG, Snijder PM, Kloosterhuis NJ, Hillebrands JL, Leemans JC, et al. Hydrogen sulfide-induced hypometabolism prevents renal ischemia/reperfusion injury. J Am Soc Nephrol. 2009; 20:1901–1905.


20. Sen N, Paul BD, Gadalla MM, Mustafa AK, Sen T, Xu R, et al. Hydrogen sulfide-linked sulfhydration of NF-κB mediates its antiapoptotic actions. Mol Cell. 2012; 45:13–24.


21. Papapetropoulos A, Pyriochou A, Altaany Z, Yang G, Marazioti A, Zhou Z, et al. Hydrogen sulfide is an endogenous stimulator of angiogenesis. Proc Natl Acad Sci U S A. 2009; 106:21972–21977.


22. Kimura Y, Dargusch R, Schubert D, Kimura H. Hydrogen sulfide protects HT22 neuronal cells from oxidative stress. Antioxid Redox Signal. 2006; 8:661–670.


23. Kimura Y, Kimura H. Hydrogen sulfide protects neurons from oxidative stress. FASEB J. 2004; 18:1165–1167.


25. Whiteman M, Armstrong JS, Chu SH, Jia-Ling S, Wong BS, Cheung NS, et al. The novel neuromodulator hydrogen sulfide: an endogenous peroxynitrite ‘scavenger’? J Neurochem. 2004; 90:765–768.


26. Circu ML, Aw TY. Reactive oxygen species, cellular redox systems, and apoptosis. Free Radic Biol Med. 2010; 48:749–762.


27. Tripatara P, Patel NS, Brancaleone V, Renshaw D, Rocha J, Sepodes B, et al. Characterisation of cystathionine gamma-lyase/hydrogen sulphide pathway in ischaemia/reperfusion injury of the mouse kidney: an in vivo study. Eur J Pharmacol. 2009; 606:205–209.


28. Han SJ, Kim JI, Park JW, Park KM. Hydrogen sulfide accelerates the recovery of kidney tubules after renal ischemia/reperfusion injury. Nephrol Dial Transplant. 2015; 30:1497–1506.


29. Roy A, Khan AH, Islam MT, Prieto MC, Majid DS. Interdependency of cystathione γ-lyase and cystathione β-synthase in hydrogen sulfide-induced blood pressure regulation in rats. Am J Hypertens. 2012; 25:74–81.


30. Cortese-Krott MM, Kuhnle GG, Dyson A, Fernandez BO, Grman M, DuMond JF, et al. Key bioactive reaction products of the NO/H2S interaction are S/N-hybrid species, polysulfides, and nitroxyl. Proc Natl Acad Sci U S A. 2015; 112:E4651–E4660.


31. Pearson G, Robinson F, Beers Gibson T, Xu BE, Karandikar M, Berman K, et al. Mitogen-activated protein (MAP) kinase pathways: regulation and physiological functions. Endocr Rev. 2001; 22:153–183.


32. Roux PP, Blenis J. ERK and p38 MAPK-activated protein kinases: a family of protein kinases with diverse biological functions. Microbiol Mol Biol Rev. 2004; 68:320–344.


33. Ballif BA, Blenis J. Molecular mechanisms mediating mammalian mitogen-activated protein kinase (MAPK) kinase (MEK)-MAPK cell survival signals. Cell Growth Differ. 2001; 12:397–408.
34. Lin A. Activation of the JNK signaling pathway: breaking the brake on apoptosis. Bioessays. 2003; 25:17–24.

