Abstract
Bladder dysfunction is a common complication of diabetes mellitus (DM). However, there have been a few studies evaluating bladder smooth muscle contraction in DM in the presence of pharmacological inhibitors. In the present study, we compared the contractility of bladder smooth muscle from normal rats and DM rats. Furthermore, we utilized pharmacological inhibitors to delineate the mechanisms underlying bladder muscle differences between normal and DM rats. DM was established in 14 days after using a single injection of streptozotocin (65 mg/kg, intraperitoneal) in Sprague-Dawley rats. Bladder smooth muscle contraction was induced electrically using electrical field stimulation consisting of pulse trains at an amplitude of 40 V and pulse duration of 1 ms at frequencies of 2–10 Hz. In this study, the pharmacological inhibitors atropine (muscarinic receptor antagonist), U73122 (phospholipase C inhibitor), DPCPX (adenosine A1 receptor antagonist), udenafil (PDE5 inhibitor), prazosin (α1-receptor antagonist), verapamil (calcium channel blocker), and chelerythrine (protein kinase C inhibitor) were used to pretreat bladder smooth muscles. It was found that the contractility of bladder smooth muscles from DM rats was lower than that of normal rats. In addition, there were significant differences in percent change of contractility between normal and DM rats following pretreatment with prazosin, udenafil, verapamil, and U73122. In conclusion, we suggest that the decreased bladder muscle contractility in DM rats was a result of perturbations in PLC/IP3-mediated intracellular Ca2+ release and PDE5 activity.
Diabetes mellitus (DM) is a metabolic disorder characterized by defective insulin secretion and hyperglycemia. Numerous patients with diabetes develop associated complications, such as cardiovascular disease, diabetic neuropathy, and kidney disease [1234]. In addition, one of the main complications of diabetes affecting quality of life is impaired bladder function, which is experienced by 50% of diabetic patients [5]. The symptoms of diabetic bladder dysfunction include increased residual urine volume after voiding and impaired detrusor contractility [67]. Approximately 23% of diabetic patients have reduced bladder contractility [8].
The urinary bladder is an elastic organ that stores urine [9]. In the bladder, the smooth muscle of the detrusor can both contract and relax to control the excretion of urine [10]. Muscarinic receptors are principally responsible for mediating bladder contraction with M2 muscarinic receptors making up about 90% of the total muscarinic receptors in bladder smooth muscle in rats [1112]. Electrical field stimulation (EFS) can trigger the release of cholinergic neuronal mediators and induce contractions through muscarinic receptors in bladder smooth muscle [131415]. Specifically, EFS-induced contraction is mediated mainly by acetylcholine (Ach) release from parasympathetic nerves. There are several types of receptors present in rat bladders, including adrenergic and purinergic receptors [161718]. Nitric oxide synthase and phosphodiesterase type 5 (PDE5) are also involved in bladder contractility [1920]. Hydrogen sulfide (H2S) can also induce contraction of bladder smooth muscle and H2S synthetic enzymes are produced in the bladder [212223]. It has been reported that PDE5 inhibitors increase contractile force in normal rat bladders [20]. Nitric oxide (NO) activates guanylate cyclase, which results in increased levels of cyclic guanosine monophosphate (cGMP), thus leads to relaxation of smooth muscle. PDE5 inhibitors stimulate relaxation of bladder smooth muscle by inhibiting cGMP degradation by PDE5.
A decrease in bladder contractility has been reported in rats with diabetes mellitus (DM rats) [24], in which a hypocontractile state has been reported, especially in animals aged 12–20 weeks [25]. This decrease in bladder contractility occurs for a variety of reasons. One is a decrease in sensitivity to ACh in DM rats [2627]. Another is changes in calcium sensitivity that have been suggested to be related to changes in bladder contraction [2829]. In addition, NO production increases in hyperglycemia and diabetic cystopathy [3031]. Taken together, these data suggest that G protein-coupled receptors (GPCRs), intracellular signaling pathways, and NO production play roles in bladder contractility in DM rats.
Using specific pharmacological inhibitors, the mechanisms underlying changes in bladder smooth muscle contraction in DM rats were identified. The pharmacological inhibitors used in this study were the muscarinic receptor antagonist atropine, the phospholipase C (PLC) inhibitor U73122, the adenosine A1 receptor antagonist DPCPX, the PDE5 inhibitor udenafil, the α1-receptor antagonist prazosin, the smooth muscle relaxer NaHS, the calcium channel blocker verapamil, and the protein kinase C (PKC) inhibitor chelerythrine. Contractions were induced electrically using electrical field stimulation (EFS) and were compared between normal rats and rats with streptozotocin (STZ)-induced DM. Therefore, the aim of the present study was to investigate the role of GPCRs, intracellular signaling pathways, and NO production in bladder smooth muscle contractility in DM rats.
Male Sprague-Dawley (SD) rats weighing 250–280 g were supplied by Samtako Bio (Osan, South Korea). The animals were housed in groups in cages with wire-net floors in a temperatureand humidity-controlled room (24–25℃ and 70–75%, respectively) with a 12-h light-dark cycle. Rats were fed a normal laboratory diet supplied by Samtako Bio (Osan, South Korea) and fasted for 24 h prior to the experiment. The experiments were performed in accordance with the guidelines and approval of the Institutional Animal Care Use Committee of Chung-Ang University (IACUC 2017-00072).
Udenafil, atropine, prazosin, NaHS, verapamil, U73122, DPCPX, chelerythrine, and STZ were all purchased from Sigma (St. Louis, MO, USA). The Krebs buffer consisted of 133 mM NaCl, 4.7 mM KCl, 2.5 mM CaCl2, 1.35 mM NaH2PO4, 0.6 mM MgSO4, 16.3 mM NaHCO3, and 7.8 mM dextrose. Unless otherwise noted, it was purchased from Sigma (St. Louis, MO, USA).
STZ is a chemical that is particularly toxic to insulin-producing beta cells in the pancreas and is often used in medical research in animal models of hyperglycemia and diabetes. Experimental diabetes was induced in overnight-fasted rats using a single dose (65 mg/kg) of STZ (Sigma). STZ was dissolved in normal saline and then administered intraperitoneally to the rats. The blood glucose levels of rats were checked two days after STZ administration using a CareSens II glucose meter from i-SENS (Seoul, South Korea). Rats with blood glucose levels higher than 300 mg/dl were considered to have DM.
Two weeks after STZ administration, the rats were sacrificed using CO2. The bladders were removed and placed in Krebs solution. The bladders were then sliced longitudinally into equal strips (2 mm×7 mm). A mixture of oxygen and CO2 gas was supplied continuously to the strips. Transversely-oriented muscle strips were taken from the rat bladders. The strips were then cut into 2–3 minor strips and silk ligatures were tied to both ends. The muscle strips were then mounted in separate 1 ml muscle chambers with one wire fixed to the bottom of the muscle chamber and the other was attached to a force transducer (FT03 Grass Instruments Co., Quincy, MA, USA). Changes in isometric force were recorded on a polygraph (Grass model 79, Quincy, MA, USA). The muscle strips were initially stretched using 1 g to generate conditions for optimal force development and were equilibrated for over 60 min while continuously being perfused with oxygenated Krebs solution.
The bladder strips were stimulated with 10 s pulse trains 40 V in amplitude with pulse durations of 1 ms at frequencies of 2-10 Hz using a stimulator (Model S 88, Grass Instruments) through platinum wire electrodes placed longitudinally on either side of the strips. After a stable resting tone of muscle strips was obtained, the frequency-response relationship (2–10 Hz) was studied, where the strips were washed five times and allowed to equilibrate for 30 mins after EFS to permit the strips to completely recover.
The contraction response to EFS is expressed as percentage (%) versus control. Data are expressed as mean±standard error of the mean. Statistically significant differences among the groups were identified using two-way repeated measures ANOVA. A p-value of less than 0.05 indicated statistical significance.
Representative tracings of tension in normal and DM rats are presented in Figs. 1A and B. Contractions were induced using EFS (2–10 Hz). The average bladder contractilities upon EFS were compared between the normal and DM rat curves and analyzed using two-way ANOVA. In DM rat muscle, there was a significant decrease in EFS-induced contractility compared to that in normal rat muscle.
As shown in Fig. 2, pretreatment with the muscarinic receptor antagonist atropine (10−6 M) inhibited EFS-induced contraction of bladder smooth muscle from both normal and DM rats. The percentages of contraction were 62% in the normal group and 58% in the DM group compared to that of the controls.
The effect of α1-adrenoceptor antagonist prazosin (10−6 M) on EFS-induced contraction of bladder smooth muscle is shown in Fig. 3. Rat bladder smooth muscle was pretreated with prazosin and contractility was measured. After pretreatment, there was a significant difference between contraction of muscles from normal and DM rats in response to EFS, where the percent contraction compared to the control was higher for DM rat muscle than for normal rat muscle.
As shown in Fig. 4, there was no difference between the normal and DM rat muscles when the muscle strips were treated with the adenosine A1 receptor antagonist DPCPX (10−6 M). In addition, there was no difference between the controls. Experiments examining the roles of GPCR-mediated signaling pathways revealed a significantly different role for a1 adrenoceptors in contraction responses between normal and DM rat muscles.
The effect of the calcium channel blocker verapamil (10−6 M) on bladder smooth muscle contraction is shown in Fig. 5A. Following treatment with verapamil, contractions in response to EFS decreased in both normal and DM rat muscles. The magnitude of this decrease in the contractions was larger in normal rat muscles than in DM rat muscles.
The effect of the PLC inhibitor U73122 (10−6 M) on bladder smooth muscle contraction is shown in Fig. 5B. Following treatment with U73122, there was a significant difference between DM and normal rat EFS-induced muscle contraction, where the percent contraction in DM rat muscles was lower than that in normal rat muscles.
The effect of the PDE5 inhibitor udenafil (10−6 M) on bladder smooth muscle contraction is shown in Fig. 6A. A significant difference was observed between normal and DM rat muscles following udenafil treatment, where the DM rat muscles had a higher percent contraction than normal rat muscles.
As shown in Fig. 6B, there was no difference in EFS-induced contractions between normal and DM rat muscles when the smooth muscle relaxer NaHS (10−6 M) was used to treat the muscle strips. In addition, there were no significant differences between the controls.
As shown in Fig. 7, there were no differences in EFS-induced contraction between normal and DM rat muscles following treatment with the PKC inhibitor chelerythrine (10−6 M). In addition, there were no differences between the controls.
In these experiments examining intracellular signaling pathways, modulators of calcium channels, PLC, and PDE5 were found to have significantly different effects on normal and DM rat bladder smooth muscle contraction in response to EFS.
A summary of the changes in EFS-induced contraction of DM rat bladder muscle following treatment with different agents is presented in Fig. 8. GPCR- and NO-mediated intracellular signaling pathways are involved in changes in the contraction or relaxation of bladder smooth muscle. In DM rats, an alteration in GPCR-mediated PLC signaling affected IP3-dependent intracellular Ca2+ release and PDE5 activity affected contraction or relaxation of bladder muscle in DM rats.
Bladder dysfunction is one of the main complications occurring in DM [32]. A decrease in detrusor contractility is a common symptom of bladder dysfunction [7]. Many different factors influence bladder smooth muscle contraction. The present study was conducted to identify receptors and/or enzymes with roles in contraction of bladder smooth muscle in a rat model of DM [33].
In this study, atropine decreased contractility in normal and DM rat bladder muscles to a similar extent. By contrast, treatment with prazosin (an α1-receptor antagonist), udenafil (a PDE5 inhibitor), verapamil (a calcium channel blocker), and U73122 (a PLC inhibitor) yielded significant differences in contractions in response to EFS between normal and DM rat muscles.
To investigate the role of GPCRs in bladder contraction in DM, atropine, prazosin, and DPCPX were used as pharmacological tools. The α1-adrenergic receptors, especially the α1A-adrenoceptor subtype, are expressed in rat bladders. Agonists acting on α1-adrenoceptors enhance EFS-induced release of ACh in isolated rat bladders. It has also been reported that α1-adrenoceptor agonists produce contractions that are about 10–43% of those achieved using muscarinic stimulation [34]. In addition, an enhanced adrenoceptor receptor response has been observed in DM rat bladders [35]. These data suggest that α1-adrenoceptors may have a role in bladder contraction in DM.
Adenosine A1 receptors are expressed in the uroepithelium of the bladder [36]. DPCPX (8-cyclopentyl-1, 3-dipropylxanthine) acts as a selective antagonist of the adenosine A1 receptor [3738]. These adenosine A1 receptors can activate PLC, increasing cytoplasmic Ca2+ concentrations, as well as inhibit adenylyl cyclase [3940]. ATP can also induce contractions through activation of P2X purinergic receptors [41]. Adenosine-induced relaxation is thought to primarily occur through P1A1 receptors because treatment with an antagonist of the P1A1 receptor (DPCPX) decreases adenosine-induced relaxation in normal rat bladder [18]. In this study, prazosin had a significantly different effect on contraction in normal and DM rat muscles.
Udenafil is a PDE5 inhibitor used for the treatment of erectile dysfunction. Interestingly, mRNA encoding PDE5 has been found in rat bladders [42]. It has also been reported that treatment with vardenafil, another PDE5 inhibitor, increases the contractility of bladder smooth muscle, potentially through changes in cGMP that lead to increases in intracellular calcium levels and thus increase bladder contraction [43]. The present study also suggests that the cGMP pathway may be involved in the control of relaxation of bladder smooth muscle in DM.
The density of muscarinic receptors in rat bladders increases during the early stages of DM [44] and M3 muscarinic receptors cause bladder contraction through a PLC-independent mechanism [45]. The main pathway for muscarinic stimulation of contraction of bladder smooth muscle involves activation of PLC, which leads to the generation of inositol-1, 4, 5-trisphosphate (IP3) [10]. IP3 induces Ca2+ release and leads to bladder contraction [46]. Numerous studies have observed alterations in calcium channel sensitivity and calcium levels in DM rats. In particular, it has been shown that intracellular calcium levels are elevated in animal models of DM [282947].
NaHS induces relaxation of bladder smooth muscle and H2S synthetic enzymes have been shown to be expressed in the bladder. However, we found no significant difference between normal and DM rat bladder smooth muscle contraction following NaHS treatment.
Several studies have investigated the effect of DM on contraction of cardiac muscle and colonic smooth muscle. It has been reported that the cholinergic response and contractility of colonic smooth muscle are reduced in DM rats. The contractile response of the proximal colon following treatment with carbachol, an ACh receptor agonist, was also found to be significantly weaker in DM rats. [48]. Another study on DM using female GK rats found reduced contraction of left ventricular muscle [49].
Our study has several limitations. First, only male rats were used. Male rats have organs not present in female rats, such as the penis and prostate. These male-specific organs may have effects on bladder smooth muscle contraction that we did not take into account. Second, the effect of age was not studied as all the rats used in this study were of a similar age (10 weeks). In this regard, it has been reported that the contractility of rat muscle changes with age [25]. Third, a hypercontractile state was also observed in DM rats. There might be various other factors affecting contraction of bladder smooth muscle. Accordingly, additional experiments will be required to address these limitations.
In conclusion, the present study found that bladder contractility was decreased in DM rats. Although atropine did not inhibit contraction of DM rat muscles, the effects of a PLC inhibitor, a calcium channel blocker, and a PDE5 inhibitor were significantly different between normal and DM rat muscles. Overall, decreased contractility of DM rat muscles was a result of alterations in PDE5 and PLC activity, including changes in the PLC signaling pathway that mediates IP3-induced intracellular Ca2+ release.
Figures and Tables
Fig. 1
Representative traces and tension comparison for electrical field stimulation-induced contraction of bladder smooth muscle from normal and diabetic rats.
(A) Electrical field stimulation (EFS)-induced contraction. The left and right sides are NM and DM rats, respectively. (B) Average tension of EFS contraction for muscles from normal (NM) and rats with diabetes mellitus (DM rats). (A, B) EFS stimulation was administered at 2, 4, 6, 8, and 10 Hz. Each point represents the mean±standard error of the mean (SEM), where n=13. *Indicates statistically significant (p<0.05) when evaluated by Student's t-test and ### indicates statistically significant (p<0.001) when evaluated by two-way ANOVA.
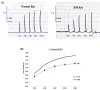
Fig. 2
Changes in contraction of smooth bladder muscles from normal and DM rats following atropine treatment.
EFS-induced contractions of muscles from normal and DM rats. Atropine was added at 10−6 M. EFS was administered using 2, 4, 6, 8, and 10 Hz. Each point represents the mean±SEM, where n=6.
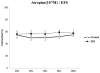
Fig. 3
Changes in contraction of bladder smooth muscles from normal and DM rats following prazosin treatment.
EFS-induced contractions of muscles from normal and DM rats. Prazosin was added at 10−6 M. EFS was administered at 2, 4, 6, 8, and 10 Hz. Each point represents the mean±SEM, where n=6. ### indicates statistically significant (p<0.001) based on two-way ANOVA.
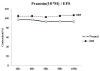
Fig. 4
Changes in contraction of bladder smooth muscles from normal and DM rats following DPCPX treatment.
EFS-induced contractions in muscles from normal and DM rats. DPCPX was added at 10−6 M. EFS was administered at 2, 4, 6, 8, and 10 Hz. Each point represents the mean±SEM, where n=6.
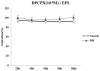
Fig. 5
Changes in contractions of bladder smooth muscles from normal and DM rats following verapamil and U73122 treatment.
(A) EFS-induced contractions following 10−6 M verapamil treatment. (B) EFS-induced contractions following 10−6 M U73122 treatment. EFS was administered at 2, 4, 6, 8, and 10 Hz. Each point represents the mean±SEM, where n=6. ## and ### indicate statistical significance (p<0.01 and p<0.001, respectively) based on two-way ANOVA.
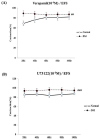
Fig. 6
Changes in contractions of bladder smooth muscles from normal and DM rats following udenafil and NaHS treatment.
(A) EFS-induced contractions following 10−6 M udenafil treatment. (B) EFS-induced contractions following 10−6 M NaHS treatment. EFS was administered at 2, 4, 6, 8, and 10 Hz. Each point represents the mean±SEM, where n=6. ### indicates statistically significant (p<0.001) based on two-way ANOVA.
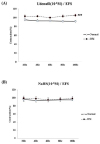
Fig. 7
Changes in contractions of bladder smooth muscles from normal and DM rats following chelerythrine treatment.
EFS-induced contractions following addition of 10−6 M chelerythrine. EFS was administered at 2, 4, 6, 8, and 10 Hz. Each point represents the mean±SEM, where n=6.
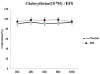
Fig. 8
Summary of mechanistic changes in smooth muscle bladder contraction in DM rats.
Both G protein-coupled receptor- and nitric oxide-mediated intracellular signaling pathways are involved in changes in the contraction or relaxation of bladder smooth muscle. Grey symbols (
) indicate signaling pathway differences between normal and DM rats. Phospholipase C generates the intracellular second messenger IP3 from the membrane lipid PIP2. IP3 then induces Ca2+ release and the released Ca2+ binds to calmodulin. Ca2+/calmodulin activates MLCK, which phosphorylates myosin light chain, thus leading to contraction. In bladder smooth muscles from DM rats, changes in G protein-coupled receptor-mediated phospholipase C signaling affects IP3-dependent Ca2+ release and changes in PDE5 affect contraction or relaxation. These factors are expected to result in a decrease in bladder contractility. DM, diabetes mellitus; EFS, electrical field stimulation; PIP2, phosphatidylinositol 4,5-bisphosphate; PLC, phospholipase C; DAG, diacylglycerol; IP3, inositol trisphosphate; CaM, calmodulin; MLCK, myosin light chain kinase; MLC-P, myosin light chain phosphatase; NO, nitric oxide; PKG, protein kinase G; PDE5, phosphodiesterase 5.

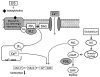
ACKNOWLEDGEMENTS
This research was supported by the Basic Science Research Program through the National Research Foundation of Korea and funded by the Ministry of Education, Science and Technology [Grant 2016R1D1A1A09918019] and the Chung-Ang University Graduate Research Scholarship in 2017.
References
1. Pecoits-Filho R, Abensur H, Betônico CC, Machado AD, Parente EB, Queiroz M, Salles JE, Titan S, Vencio S. Interactions between kidney disease and diabetes: dangerous liaisons. Diabetol Metab Syndr. 2016; 8:50.


2. Weissman AJ. Intensive diabetes treatment and cardiovascular disease. N Engl J Med. 2006; 354:1751–1752. author reply 1751-1752.


3. Amaral N, Okonko DO. Metabolic abnormalities of the heart in type II diabetes. Diab Vasc Dis Res. 2015; 12:239–248.


4. Tuttle KR, Bakris GL, Bilous RW, Chiang JL, de Boer IH, Goldstein-Fuchs J, Hirsch IB, Kalantar-Zadeh K, Narva AS, Navaneethan SD, Neumiller JJ, Patel UD, Ratner RE, Whaley-Connell AT, Molitch ME. Diabetic kidney disease: a report from an ADA Consensus Conference. Am J Kidney Dis. 2014; 64:510–533.


5. Van Den Eeden SK, Sarma AV, Rutledge BN, Cleary PA, Kusek JW, Nyberg LM, McVary KT, Wessells H. Diabetes Control and Complications Trial/Epidemiology of Diabetes Research Group. Effect of intensive glycemic control and diabetes complications on lower urinary tract symptoms in men with type 1 diabetes: Diabetes Control and Complications Trial/Epidemiology of Diabetes Interventions and Complications (DCCT/EDIC) study. Diabetes Care. 2009; 32:664–670.


6. Ioanid CP, Noica N, Pop T. Incidence and diagnostic aspects of the bladder disorders in diabetics. Eur Urol. 1981; 7:211–214.


7. Bradley WE. Diagnosis of urinary bladder dysfunction in diabetes mellitus. Ann Intern Med. 1980; 92:323–326.


8. Kaplan SA, Te AE, Blaivas JG. Urodynamic findings in patients with diabetic cystopathy. J Urol. 1995; 153:342–344.


10. Andersson KE, Arner A. Urinary bladder contraction and relaxation: physiology and pathophysiology. Physiol Rev. 2004; 84:935–986.


11. Wang P, Luthin GR, Ruggieri MR. Muscarinic acetylcholine receptor subtypes mediating urinary bladder contractility and coupling to GTP binding proteins. J Pharmacol Exp Ther. 1995; 273:959–966.
12. Park SY, Je HD, Shim JH, Sohn UD. Characteristics of spontaneous contraction in the circular smooth muscles of cat ileum. Arch Pharm Res. 2010; 33:159–165.


13. Sjögren C, Andersson KE, Husted S, Mattiasson A, Moller-Madsen B. Atropine resistance of transmurally stimulated isolated human bladder muscle. J Urol. 1982; 128:1368–1371.


14. Min CH, Min YS, Lee SJ, Sohn UD. The comparative effects of aminoglycoside antibiotics and muscle relaxants on electrical field stimulation response in rat bladder smooth muscle. Arch Pharm Res. 2016; 39:863–870.


15. Min CH, Wang Y, Bae J, Han JH, Sohn UD. The inhibitory mechanism of gentamicin on electrical field stimulation response in rat bladder smooth muscle. Korean J Physiol Pharmacol. 2015; 19:473–478.


16. Braverman AS, Tibb AS, Ruggieri MR Sr. M2 and M3 muscarinic receptor activation of urinary bladder contractile signal transduction. I. Normal rat bladder. J Pharmacol Exp Ther. 2006; 316:869–874.


17. Kullmann FA, Downs TR, Artim DE, Limberg BJ, Shah M, Contract D, de Groat WC, Rosenbaum JS. Urothelial beta-3 adrenergic receptors in the rat bladder. Neurourol Urodyn. 2011; 30:144–150.


18. Vesela R, Aronsson P, Tobin G. Functional and morphological examinations of P1A1 purinoceptors in the normal and inflamed urinary bladder of the rat. Auton Neurosci. 2011; 159:26–31.


19. Johansson R, Pandita RK, Poljakovic M, Garcia-Pascual A, De Vente J, Persson K. Activity and expression of nitric oxide synthase in the hypertrophied rat bladder and the effect of nitric oxide on bladder smooth muscle growth. J Urol. 2002; 168:2689–2694.


20. Matsumoto S, Hanai T, Uemura H. Chronic treatment with a PDE5 inhibitor increases contractile force of normal bladder in rats. Int Urol Nephrol. 2010; 42:53–56.


21. Dombkowski RA, Doellman MM, Head SK, Olson KR. Hydrogen sulfide mediates hypoxia-induced relaxation of trout urinary bladder smooth muscle. J Exp Biol. 2006; 209:3234–3240.


22. Patacchini R, Santicioli P, Giuliani S, Maggi CA. Pharmacological investigation of hydrogen sulfide (H2S) contractile activity in rat detrusor muscle. Eur J Pharmacol. 2005; 509:171–177.


23. Patacchini R, Santicioli P, Giuliani S, Maggi CA. Hydrogen sulfide (H2S) stimulates capsaicin-sensitive primary afferent neurons in the rat urinary bladder. Br J Pharmacol. 2004; 142:31–34.


24. Mustafa S, Ismael HN. Reactivity of diabetic urinary bladder to the cholinesterase inhibitor neostigmine. Urology. 2014; 84:1549.e1–1549.e5.


25. Daneshgari F, Liu G, Imrey PB. Time dependent changes in diabetic cystopathy in rats include compensated and decompensated bladder function. J Urol. 2006; 176:380–386.


26. Longhurst PA, Belis JA. Abnormalities of rat bladder contractility in streptozotocin-induced diabetes mellitus. J Pharmacol Exp Ther. 1986; 238:773–777.
27. Je HD, Park SY, Barber AL, Sohn UD. The inhibitory effect of rosiglitazone on agonist-induced or spontaneous regulation of contractility. Arch Pharm Res. 2007; 30:461–468.


28. Waring JV, Wendt IR. Effects of streptozotocin-induced diabetes mellitus on intracellular calcium and contraction of longitudinal smooth muscle from rat urinary bladder. J Urol. 2000; 163:323–330.


29. Belis JA, Curley RM, Wagner CH, Murty VN, Winter SJ, Rohner TJ Jr. Neurogenic function of the diabetic rat bladder: alteration by calcium channel effectors. Pharmacology. 1991; 43:273–281.


30. Poladia DP, Bauer JA. Early cell-specific changes in nitric oxide synthases, reactive nitrogen species formation, and ubiquitinylation during diabetes-related bladder remodeling. Diabetes Metab Res Rev. 2003; 19:313–319.


31. Adela R, Nethi SK, Bagul PK, Barui AK, Mattapally S, Kuncha M, Patra CR, Reddy PN, Banerjee SK. Hyperglycaemia enhances nitric oxide production in diabetes: a study from South Indian patients. PLoS One. 2015; 10:e0125270.


33. Huang L, Chen D, Li S. Streptozotocin diabetes attenuates the effects of nondepolarizing neuromuscular relaxants on rat muscles. Korean J Physiol Pharmacol. 2014; 18:461–467.


34. Michel MC, Vrydag W. Alpha1-, alpha2- and beta-adrenoceptors in the urinary bladder, urethra and prostate. Br J Pharmacol. 2006; 147:Suppl 2. S88–S119.
35. Kudlacz EM, Gerald MC, Wallace LJ. Effects of diabetes and diuresis on contraction and relaxation mechanisms in rat urinary bladder. Diabetes. 1989; 38:278–284.


36. Yu W, Zacharia LC, Jackson EK, Apodaca G. Adenosine receptor expression and function in bladder uroepithelium. Am J Physiol Cell Physiol. 2006; 291:C254–C265.


37. Martinson EA, Johnson RA, Wells JN. Potent adenosine receptor antagonists that are selective for the A1 receptor subtype. Mol Pharmacol. 1987; 31:247–252.
38. Lohse MJ, Klotz KN, Lindenborn-Fotinos J, Reddington M, Schwabe U, Olsson RA. 8-Cyclopentyl-,3-dipropylxanthine (DPCPX)--a selective high affinity antagonist radioligand for A1 adenosine receptors. Naunyn Schmiedebergs Arch Pharmacol. 1987; 336:204–210.
39. Schulte G, Fredholm BB. Signalling from adenosine receptors to mitogen-activated protein kinases. Cell Signal. 2003; 15:813–827.


40. Bucheimer RE, Linden J. Purinergic regulation of epithelial transport. J Physiol. 2004; 555:311–321.


41. Inoue R, Brading AF. The properties of the ATP-induced depolarization and current in single cells isolated from the guinea-pig urinary bladder. Br J Pharmacol. 1990; 100:619–625.


42. Zhu X, Zhai K, Mi Y, Ji G. Expression and function of phosphodiesterases (PDEs) in the rat urinary bladder. BMC Urol. 2017; 17:54.


43. Rybalkin SD, Yan C, Bornfeldt KE, Beavo JA. Cyclic GMP phosphodiesterases and regulation of smooth muscle function. Circ Res. 2003; 93:280–291.


44. Tong YC, Chin WT, Cheng JT. Alterations in urinary bladder M2-muscarinic receptor protein and mRNA in 2-week streptozotocininduced diabetic rats. Neurosci Lett. 1999; 277:173–176.


45. Sand C, Michel MC. Bradykinin contracts rat urinary bladder largely independently of phospholipase C. J Pharmacol Exp Ther. 2014; 348:25–31.


46. Somlyo AP, Somlyo AV. Ca2+ sensitivity of smooth muscle and nonmuscle myosin II: modulated by G proteins, kinases, and myosin phosphatase. Physiol Rev. 2003; 83:1325–1358.


47. Levy J, Gavin JR 3rd, Sowers JR. Diabetes mellitus: a disease of abnormal cellular calcium metabolism. Am J Med. 1994; 96:260–273.


48. Kim SJ, Park JH, Song DK, Park KS, Lee JE, Kim ES, Cho KB, Jang BK, Chung WJ, Hwang JS, Kwon JG, Kim TW. Alterations of colonic contractility in long-term diabetic rat model. J Neurogastroenterol Motil. 2011; 17:372–380.


49. Iltis I, Kober F, Desrois M, Dalmasso C, Lan C, Portha B, Cozzone PJ, Bernard M. Defective myocardial blood flow and altered function of the left ventricle in type 2 diabetic rats: a noninvasive in vivo study using perfusion and cine magnetic resonance imaging. Invest Radiol. 2005; 40:19–26.