Abstract
Post-traumatic stress disorder (PTSD) is a trauma-induced psychiatric disorder characterized by impaired fear extermination, hyperarousal, and anxiety that may involve the release of monoamines in the fear circuit. The reported pharmacological properties of tetramethylpyrazine (TMP) include anti-cancer, anti-diabetic, anti-atherosclerotic, and neuropsychiatric activities. However, the anxiolytic-like effects of TMP and its mechanism of action in PTSD are unclear. This study measured several anxiety-related behavioral responses to examine the effects of TMP on symptoms of anxiety in rats after single prolonged stress (SPS) exposure by reversing the serotonin (5-HT) and hypothalamic-pituitary-adrenal (HPA) axis dysfunction. Rats were given TMP (10, 20, or 40 mg/kg, i.p.) for 14 days after SPS exposure. Administration of TMP significantly reduced grooming behavior, increased the time spent and number of visits to the open arm in the elevated plus maze test, and significantly increased the number of central zone crossings in the open field test. TMP administration significantly reduced the freezing response to contextual fear conditioning and significantly restored the neurochemical abnormalities and the SPS-induced decrease in 5-HT tissue levels in the prefrontal cortex and hippocampus. The increased 5-HT concentration during TMP treatment might be partially attribute to the tryptophan and 5-hydroxyindoleacetic acid mRNA level expression in the hippocampus of rats with PTSD. These findings support a role for reducing the altered serotonergic transmission in rats with PTSD. TMP simultaneously attenuated the HPA axis dysfunction. Therefore, TMP may be useful for developing an agent for treating psychiatric disorders, such those observed in patients with PTSD.
Post-traumatic stress disorder (PTSD) is a chronic stress-induced psychiatric condition that develops immediately or a long time after exposure to a traumatic, life-threatening event [12]. The characteristic signs of PTSD include hyperarousal, insensibility, fear, and nightmares [34]. The onset of PTSD often precedes and increases the risk for the subsequent development of anxiety disorders, and PTSD enhances susceptibility to anxiety [5]. Altered serotonin (5-HT) levels, a core neurochemical feature of PTSD, contribute to multiple PTSD-related behaviors, including hypervigilance, impulsivity, aggression, depression, and anxiety [6]. Central 5-HT appears to have a positive effect on fear conditioning [7]. Many studies have also shown that hyperarousal and dysregulation of the hypothalamic-pituitary-adrenal (HPA) axis are closely associated with the development of PTSD, which produces marked changes in affective behavior that are indicative of or consistent with anxiety-like symptoms [89].
The single prolonged stress (SPS), which models a traumatic, stressful event, has been used widely because it has high validity when examining trauma-induced learning or anxiety [1011]. Rats exposed to SPS show enhanced dysregulation of the HPA axis [1213], increased fear conditioning, and increased anxiety-like behavior on the elevated plus maze (EPM) test [11]. These responses imitate the clinical symptoms observed in patients with PTSD [10].
Tetramethylpyrazine (TMP) is one of the most important active ingredients in the Asian traditional medicine herb Ligusticum wallichii Franch [14]. TMP can pass through the blood-brain barrier and is widely used in the treatment of neurovascular and cardiovascular diseases [151617]. For example, TMP exerts neuroprotective, antioxidant, anti-apoptotic, and anti-inflammatory effects in central nervous system-related disorders, such as forebrain ischemia, Parkinson's disease, and Alzheimer's disease [181920]. Some studies have shown that TMP protects against ischemic brain damage and promotes cell proliferation [21]. TMP also protects learning and memory functions and rescues cholinergic dysfunction in D-galactose-lesioned mice [22], a chronic cerebral hypoperfusion model [23], and rats given streptozotocin injections [24]. It has multiple functions, including the prevention of oxidative stress-induced neuronal death, calcium channel blockade, protection against kainite-induced excitotoxicity, and enhanced mitochondrial biosynthesis [252627]. TMP increases the expression of Bcl-2, but decreases that Bax, in the spinal cord, thereby exerting an anti-apoptotic effect [2829]. Therefore, we hypothesized that TMP would protect against the development of anxiety-like behaviors, which represent the symptoms of PTSD, in a rat model of PTSD using SPS.
Therefore, this study used the EPM test, open field test (OFT), and contextual fear conditioning and fear extinction to investigate the effects of TMP on the anxiety-related behaviors in rats exposed to SPS. We also examined how the behavioral effects were associated with the underlying mechanism of the serotonergic system in the brain. To investigate the possible mechanisms underlying the anti-PTSD-like effects of TMP further, we also studied HPA axis activation after chronic TMP treatment.
Six-week-old adult male Sprague-Dawley rats (Samtako Animal Co., Seoul, Korea), weighing 220–250 g, were used in all of the experiments. The rats were housed in a limited access rodent facility with up to five rats per polycarbonate cage. The rats were sustained on a 12-h light/dark cycle (lights on at 8:00 am, lights off at 8:00 pm) under a controlled temperature (23±5℃) and relative humidity (55±5%) conditions. All of the animals adapted to this condition during the 7 days after their arrival. All of the methods were approved by the Animal Care and Use Committee of Kyung Hee University [KHUASP(SE)-15-115]. All of the procedures were executed according to the Guide for the Care and Use of Laboratory Animals issued by the Korea National Institute of Health.
After exposure to SPS, TMP (10, 20, and 40 mg/kg, body weight, Sigma-Aldrich Chemical Co., St. Louis, MO, USA) and fluoxetine (10 mg/kg, FLX, fluoxetine hydrochloride; Sigma), which was used as the positive control, were intraperitoneally (i.p.) injected at a volume of 1 ml/kg for 14 days. The standard doses of TMP and FLX in the rat and considering the long-term treatment used in the present study was based on previous study [20]. In total, six to seven animals were allotted to each group. TMP and FLX were dissolved in 0.9% saline before use. As a vehicle control, animals in the saline-treated (SAL) group were intraperitoneally given the equivalent volumes in saline without SPS exposure. Another group was pretreated with TMP (40 mg/kg, i.p., TMP group) without SPS exposure. The entire experimental schedules of SPS and behavioral examinations are shown in Fig. 1.
Rats were subjected to SPS according to a slightly modified version of the procedure described by Enman's groups [130]. Briefly, rats were controlled for 2 h by a holder and then were subjected to a forced swimming condition for 20 min. The rats were dried and allowed to recuperate for 15 min, and then were moved to a separate chamber where they were exposed to isoflurane until loss of consciousness (<1 min). Isoflurane exposure was conducted by placing a cotton ball soaked with isoflurane into an open conical tube and placed into the chamber (2–4 rats simultaneously). Rats were housed in pairs undisturbed for the next 7 days, with the exception of cage changes. All further experimental procedures began after the 7-day isolation period. Control animals were weighed daily and housed in pairs.
The EPM test was carried out according to a method described previously [30]. Briefly, the rats were transferred to the EPM, which consisted of a 4-armed wooden platform in the shape of a plus sign. The apparatus was painted with black enamel and was raised 50 cm above the floor. All arms were 50 cm in length, 10 cm in width, and joined in the center to create a 10 cm2 center platform. Two arms facing away from each other were protected, whereas the remaining two arms remained open. At the start of each experiment, the rat could move freely for 5 min. The video footage of these sessions was scored. The ratio of the total time spent in the open and closed arms were used to measure anxiety. The anxiety index was calculated as follows:
Anxiety index values range from 0 to 1 where an increase in the index expresses increased anxiety-like behavior.
The rats completed the OFT before the EPM. The OFT was performed according to a previously described method [31]. The open field area consisted of an enclosed square area made of dark opaque Plexiglas (60 × 60 cm) surrounded by walls (30 cm in height). All of the locomotor activities (animal movements) were measured with a computerized video-tracking system using the S-MART program (PanLab Co., Barcelona, Spain). The locomotor activity was evaluated in terms of total distance traveled in the container, and exploratory activity was evaluated based on measurements of the total number of line crossings during 5 min. Painted white lines divided the area into 16 squares (15×15 cm each). Time spent, and number of zone crossings in the central and peripheral zones were observed. The number of rearing events for each rat was also recorded to analyze locomotor activity in the OFT.
A separate group of rats that did not undergo the EPM testing (n=4–5 for each group) was tested for contextual fear conditioning and extinction, after the SPS procedure. Contextual fear conditioning tests took place over 3 days, and followed the procedures according to a method described previously [32]. Briefly, on the acquisition day, rats were placed in a foot shock chamber (30 cm long×26 cm wide×22 cm high) with an overhead camera, and were allowed to explore freely for 2 min. After 2 min, a total of 10 electric shocks (0.75 mA, 2 s duration) were delivered at intervals of 74 s through the testing chamber floor. The rats then remained in the chamber for an additional 5 min with no shocks delivered. On test days 1–2, rats were placed for 8 min in same chambers as on acquisition day, with no shocks delivered, to determine the extent of contextual fear learning and extinction of contextual fear conditioning. This behavior was chosen because it was previously shown to induce a re-experiencing of the aversive event and to facilitate behavioral sensitization. The percentage of freezing responses was calculated by dividing the freezing time by the total time. Freezing behavior was defined as complete immobility except for minor movements required for respiration.
After the 15 days of rest designed to allow the development of PTSD, corticosterone (CORT), corticotropin-releasing hormone (CRH), and adrenocorticotroic hormone (ACTH) in the plasma, and 5-HT concentration in the brain tissue were assayed according to a previously described procedure [31]. Four rats from each group were deeply anesthetized through inhalation with isoflurane (1.2%), and were sacrificed one day after behavioral testing. The plasma was quickly collected via the abdominal aorta, and the medial prefrontal cortex (mPFC), hippocampus (HPC), striatum (STR), and amygdala (AMYG) were rapidly removed from the rat brain in a randomized order. Special care was taken to avoid pre-sacrifice stress and the rats were killed rapidly. The CORT, CRH, ACTH, and 5HT concentration were evaluated by a competitive enzyme-linked immunoassays (ELISAs) using a CORT antibody (Novus Biologicals, LLC., Littleton, CO, USA), a CRH antibody (Novus Biologicals), a ACTH antibody (Novus Biologicals), and a 5-HT antibody (Abcam, Cambridge, MA, USA) according to the manufacturer's protocols.
The expression of tryptophan (TRP), 5-hydroxyindoleacetic acid (5-HIAA), and vesicular monoamine transporter-2 (VMAT-2) mRNA expression were measured by RT-PCR according to a method described previously [31]. In brief, total RNA was extracted from the HPC of each rat using TRIzol reagent (Life Technologies, Carlsbad, CA, USA) according to the manufacturer's instructions. cDNA was synthesized from 2 µg total RNA using reverse transcriptase (Takara Bio, Otsu, Japan) with random hexamers (COSMO Genetech, Seoul, Korea). Then it was amplified at 60℃ for 28 cycles for TRP, at 60℃ for 30 cycles for 5-HIAA, and at 56℃ for 35 cycles for VMAT-2 using Taq DNA polymerase (Takara, Kyoto, Japan) on a thermal cycler (MJ Research, Watertown, MA, USA). Data were normalized against glyceraldehyde 3-phosphate dehydrogenase (GADPH) expression in the corresponding sample.
All of the measurements were performed by an independent investigator blind to the experimental conditions, and the results are expressed as mean±standard error of the mean. Differences within or between normally distributed data were analyzed with analysis of variance (ANOVA) with SPSS (version 13.0; SPSS, Inc., Chicago, IL, USA) and Tukey's post hoc tests. Between-subjects two-way ANOVA was used to analyze the effects of TMP treatment and time. In all of the analyses, differences were considered statistically significant at p-value <0.05.
Rats exhibited an obvious anxiety phenotype characterized by reduced open-arm exploration during the EPM test. Statistical analyses of the behavioral results showed that the percentage of time spent in the open arms of the maze significantly differed among the 7 groups [F(6,39)=5.035, p<0.01] (Fig. 2). The ANOVA also revealed a significant effect of the number of open arm entries among the 7 groups [F(6,39)=5.049, p<0.01]. Post-hoc comparisons indicated that the percentage of time spent and the number of entries in the open arms of the maze decreased significantly in the PTSD group compared with the control group (p<0.05 and p<0.01). However, rats in the PTSD+TMP40 group showed significant restoration of the time spent and the number of entries in the open arms of the maze compared with that of the PTSD group (p<0.05). The percentage of time spent and the number of entries in the closed arms of the maze did not differ significantly among the seven groups [F(6,39)=1.466, p=0.230 and F(6.39)=1.948, p=0.116]. TMP administration after SPS elicited anxiogenic or anxiolytic behavior. These findings indicated that increased time spent and number of entries in the open arms of the maze in the PTSD+TMP40 group was comparable to the exploratory behavior in the PTSD+FLX group. Overall, the anxiety index, calculated based on the number of visits to and time spent in the open and closed arms, also differed among the 7 groups of rats, with lower values in the TMP-treated rats (p<0.01). Anxiety reduction, indicated by open arm exploration in the EPM, was defined as an increase in the numbers of entries into the open arms relative to total entries into either open or closed arm, and an increase in the proportion of time spent in the open arms relative to total spending time in either open or closed arm. Also, unprotected head dips were defined as peering over the edge of an open arm with head, neck, and shoulders. Administering TMP significantly increased the frequency of unprotected head dips compared with that in the PTSD group, although this result was only marginally significant. Grooming behavior has been reported to be a response to novelty or other stressors and might vary in accordance with stress intensity. However, the duration of grooming behavior was reversed by 40 mg/kg TMP when administered after SPS exposure (p<0.05).
The OFT was used to assess the exploratory behavior and locomotion of the rats who underwent the SPS procedure (Fig. 3). Rats exposed to SPS spent significantly less time in the central zone and correspondingly more time in the peripheral zone compared to the saline (SAL) group (p<0.05). There was also a significant decrease in the number of central zone crossings following the SPS procedure (p<0.01). Our results indicated that SPS-treated rats developed exploratory activities that were closely associated with the anxiety-like behaviors observed in the OFT. However, the TMP-treated rats (40 mg/kg) displayed a significant increase in the number of central zone crossings compared with rats in the PTSD group (p<0.05), indicating that the anxiety-like behaviors of the PTSD+TMP40 group were similar to those of the PTSD+FLX group. A one-way ANOVA was performed, and PTSD-related differences were discovered in locomotor activity (motor function) and the total number of rearing (exploratory activities) in the OFT. A significant difference in locomotor activity was observed between saline-treated rats, the PTSD group, and the TMP-treated groups [F(6,39)=3.858, p<0.01] and the total times reared [F(6,39)=5.251, p<0.01]. Rats exposed to SPS had significantly decreased moving distance and rearing frequency in the open field compared with the SAL group (p<0.01). Administering TMP significantly increased the moving distance compared with that in the PTSD group, although this result was only marginally significant. However, rats in the PTSD+TMP40 group showed a significant restoration of the rearing frequency in the open field compared with that in the PTSD group (p<0.05).
The effects of TMP administration on the contextual freezing behavior of rats are shown in Fig. 4. Unconditioned freezing duration in response to foot shock was not different between groups [F(5,35)=0.235, p=0.547]. In contrast, two way ANOVA across the three testing sessions revealed a significant main effect of treatment [F(5,35)=10.45, p<0.01], a significant main effect of time [F(5,35)=29.54, p=0.745] and a significant interaction between treatment and time [F(5,35)=2.450, p<0.05] for freezing behavior. In the contextual freezing measurement, the freezing time significantly increased after exposure to SPS (p<0.01 on days 1 and 2). The percentage of time spent displaying freezing behavior was significantly decreased in the group treated with 40 mg/kg TMP on days 1 and 2, respectively (p<0.05). These results indicate that a persistent fear response to the original context was associated with the traumatic events that occurred there and that repeated TMP treatment ameliorated the context-dependent freezing behavior in the rats.
Fig. 5 shows differences in the regional levels of 5-HT among the group. The post-hoc test results indicated a significant decrease in 5-HT levels in the mPFC of the PTSD groups compared to the SAL (p<0.01). Daily administration of TMP significantly increased the SPS-induced decrease in the 5-HT concentration in the mPFC compared with the PTSD group (p<0.05). The post-hoc test results indicated a significant decrease in 5-HT levels in the HPC of the PTSD groups compared to the SAL (p<0.01). Daily administration of TMP significantly increased the SPS-induced decrease in the 5-HT concentration in the HPC compared with the PTSD group (p<0.05).
After treatment with TMP, the levels of 5-HT in the STR were significantly reversed, by 211.94% of that in the PTSD group, although this result was only marginally significant. After treatment with TMP, the levels of 5-HT in the AMYG were significantly reversed, by 214.12% of that in the PTSD group, although this result was only marginally significant. Additionally, 5-HT concentrations in the brain regions of rats receiving 10 mg/kg FLX were similar to those of rats receiving 40 mg/kg TMP.
To examine the effect of TMP on the expression of TRP, 5-HIAA, and VMAT-2 mRNAs in rat hippocampus injured by SPS, the mRNAs expression of TRP, 5-HIAA, and VMAT-2 was analyzed by RT-PCR (Fig. 6). The mRNA level of TRP in the PTSD group decreased significantly compared with that in the SAL group (p<0.01), the expression levels in the PTSD group were similar to those in the SAL group after treatment with 40 mg/kg TMP (p<0.05). The mRNAs level of 5-HIAA and VMAT-2 in the PTSD group decreased significantly compared with that in the SAL group (p<0.01 and p<0.05). The expression levels of 5-HIAA mRNA in the PTSD group was significantly restored to a level similar to those in the SAL group after treatment with 40 mg/kg TMP (p<0.05). The expression levels of VMAT-2 mRNA in the PTSD group was significantly restored by 40 mg/kg TMP, although this result was only marginally significant. This indicates that TRP and 5-HIAA mRNAs expression levels in the hippocampus of rats receiving 40 mg/kg TMP were similar to those of rats receiving 10 mg/kg FLX.
ELISA analysis showed that rats who underwent SPS exposure had significantly increased plasma CORT concentration (317.42%) compared to rats in the SAL group (p<0.01; Fig. 7). However, the administration of TMP decreased the SPS-induced increase in plasma CORT levels as compared with the PTSD group (p<0.05). ELISA analysis showed that rats who underwent SPS exposure had increased plasma CRH concentration (246.87%) compared to rats in the SAL group, although this result was only minimally statistically significant. However, the administration of TMP decreased the SPS-induced increase in plasma CRH levels as compared with the PTSD group, although this result was only minimally statistically significant. ELISA analysis showed that rats who underwent SPS exposure had significantly increased plasma ACTH concentration (282.63%) compared to rats in the SAL group (p<0.01). However, the administration of TMP decreased the SPS-induced increase in plasma ACTH levels as compared with the PTSD group (p<0.05). The results suggested that the anti-PTSD-like effects of TMP were associated with decreased HPA axis stress hormone levels. Thus, the SPS procedure induced anxiety-like symptoms in rats, which was used to develop a PTSD model. Inhibition of the increase in plasma CORT, CRH and ACTH levels by TMP also provides a basis for the inference that SPS induces memory impairment in rats.
This study, evaluated the anti-PTSD-like effects and possible mechanisms of action of TMP using the SPS model. Administration of TMP after SPS exposure significantly increased the time spent in and the number of entries into the open arms in the EPM, reduced the anxiety index, and decreased grooming behavior. Administration of TMP after SPS also significantly reduced the anxiety-like behaviors, as indicated by an increase in the number of central zone crossings during the OFT. TMP administration significantly decreased the freezing response to contextual fear conditioning, and it appeared to act as an anxiolytic by preventing the reduction in the brain 5-HT levels. We also found that the anti-PTSD-like effects of TMP were associated with the prevention of HPA axis dysfunction. These results could lead to the development of novel therapeutics for the treatment of PTSD.
The behavioral features induced by SPS include cognitive dysfunction [3334], disrupted extinction learning [3536], exaggerated startle responses [1], and heightened fear [37], which are related to the hyperarousal, avoidance, and clinical symptoms of intrusion seen in patients with PTSD [1]. Therefore, we used the SPS to assess anxiety-like behavioral effects.
Studies have reported that rats exposed to isoflurane for the SPS procedure showed a decreased freezing response to contextual fear conditioning and increased serum corticosterone levels [38]. The results of that study also revealed that the SPS procedure using isoflurane induced PTSD-like symptoms in rats, as evidenced by their exploratory behavior [38]. This facilitated the development of anxiety. It is ethically difficult to evaluate PTSD prospectively in humans. Animal models present an alternative approach to investigating the disorder. While animal models do not completely reflect the human psychological state, they can be used to assess aspects of this state. We used isoflurane as an alternative to ether in the SPS model. Some studies have shown that isoflurane blocks associative fear conditioning. Isoflurane given during training reduced conditional fear dose-dependently during the training. However, the authors also suggested that stresses imposed during anesthesia could lead to PTSD symptoms in the distant future [39]. Therefore, we chose SPS as the traumatic stress, because it is among the most reliable models of PTSD, characterized by high face validity and the core etiological factors of this disease, such as reduced sensorimotor gating of the startle response, anxiety-like behaviors, and enhanced negative feedback of the HPA axis.
Stressful life experiences are a risk factor for major depression or anxiety, and serotonergic dysfunction is obvious in individuals with these disorders [30]. The SPS procedure, an animal model of PTSD, decreased the proportion of time that rats spent in as well as the number of entries they made into the open arms on the EPM [40]. Our behavioral findings demonstrated the anxiolytic-like effects of TMP in a rodent model of anxiety. Administration of TMP after SPS significantly reduced the anxiety-like behaviors in the EPM test, as indicated by increased exploratory behavior and more entries into the open arms. Administration of TMP after SPS also significantly increased the number of central zone crossings in the OFT. These changes in anxiety-like behavior appeared to be specific and a result of locomotor activity because the length of the tracks in the OFT and EPM test were similar for all groups. In this study, grooming behavior also reflected better adaptation to novel stress in rats treated with TMP, consistent with previous EPM test results [41]. Therefore, because the behaviors in the EPM test and OFT are related to serotonergic system-associated psychological symptoms, our results suggest that TMP inhibits serotonergic system dysregulation.
A classic exploratory behavior measured in the OFT is rearing behavior [42]. Although initially thought to be highly correlated with activity, careful factor analyses of OFT data revealed that this relationship is more complicated [4344]. For example, the two types of rearing load onto two different factors; wall leaning loads only onto a factor related to locomotion/activity, while rearing also loads onto a factor related to emotional behavior, a marker related to stress and anxiety [45]. Interestingly, a range of stress models and modulators in rats potentially affect the overall rearing behavior [45]. Some studies of capsaicin-treated rats during the activity test reported that the number of episodes of rearing, repetitive standing with two forepaws up, was increased and rostral grooming decreased [46]. Therefore, increased rearing and decreased rostral grooming in capsaicin-treated rats may reveal an anxiolytic efficacy of oral capsaicin. Not only increased rearing but also increased locomotor and center zone activities were interpreted as reflecting reduced anxiety. However, some studies have reported that predator stressed rats traveled a significantly shorter total distance than that of control rats, while the rearing times remained slightly increased in the OFT [47]. Social isolation stress also shows slightly less rearing compared with that in control rats in the OFT [48]. Many studies have suggested that abnormal social interactions and increased anxiety behavior predict the onset of depression [47]. Some studies have suggested that rats subjected to 3 consecutive weeks of chronic unpredictable mild stress exhibited the behavioral deficits, that are symptoms of most human depressive states, including decreased sucrose preference, reduced crossing and rearing activity in OFT, and prolonged immobility time in the FST [47]. In this study, these behavioral changes in rats after exposure to SPS in various behavioral tests and the results of several anxiety indexes, such as the time spent and distance travelled in the central zone and locomotor activity, suggest that anxiety-like behavior was successfully established. Therefore, our results showed that TMP treatment alleviated anxiety-like behavior in SPS-treated rats, as indicated by their decreased rearing frequency in the OFT.
Furthermore, consistent with previous studies, our results indicate that the SPS model produced enhanced contextual freezing and anxiety-like behavior in the OFT, as well as stress-induced analgesia compared with that demonstrated by the control group [49]. The SPS-induced freezing behavior also supported the notion that PTSD is characterized by an exaggerated reaction, which would be appropriate for the original traumatic situation, to a mild stressor or to a reminder of the trauma [49]. Therefore, SPS exposure led to the acquisition of conditioned fear responses to trauma-related stimuli [50]. The data presented here support the idea that this PTSD model resembles the clinical symptoms of PTSD. Therefore, our results imply that TMP ameliorates fearful memories and the anxiety induced by traumatic stress.
Our results show that anxiety following SPS is consistent with an impaired serotonergic system in patients with PTSD. They also show reduced tissue 5-HT levels in the mPFC, STR, AMYG, and HPC of SPS rats, suggesting a reduction in serotonergic tone in the mesolimbic pathway. Therefore, PTSD symptoms are reexperienced due to dysfunction in or imbalance of 5-HT within the anxiety circuit areas of the mRFC, STR, AMYG, and HPC [75152]. We have shown that animals with PTSD treated with TMP had significantly increased 5-HT levels in the mRFC and HPC, and this may have inhibited the pathophysiology of PTSD. The TMP could be reverse by manipulating 5-HT [7]. Therefore, our results indicate that 5-HT, like FLX, restores the behavior and neurochemical alterations associated with anxiety-like symptoms by modulating the 5-HT system in the brain [53].
Our study demonstrated concomitant changes in the neural transmission of candidate catecholamines, in which 5-HT efflux in the HPC was reduced after 2 weeks in rats that underwent the SPS procedure. We then observed a more dynamic relationship in which area-dependent consistency existed between the release and reuptake of specific 5-HT after a traumatic experience. 5-HT regulates mood-related behaviors via different 5-HT receptors and subsequent signaling transduction pathways [6]. The SPS procedure decreased 5-HT efflux and TRP, 5-HIAA, and VMAT-2 mRNA levels in the HPC, indicating the possible association of presynaptic adaptation or plasticity [6]. TRP synthesizes 5-HT and is an important marker in the traumatic stress-induced response in the serotonergic system and in stress-related psychopathological conditions, including fear or anxiety [6]. Our results show that 5-HIAA decreased in the HPC, with an accompanying change in VMAT-2, which reflects presynaptic 5-HIAA activity, in rats subjected to the SPS procedure [2]. The SPS-altered increase in 5-HT levels also reflects neuronal adaptation in synaptic release rather than reuptake, because the accompanying reduction in VMAT-2 means that a decreased quantity of the neurotransmitter in vesicles is available for exocytosis [54]. The accompanying decreases in both VMAT-2 and 5-HA efflux in the HPC suggest that SPS alters the synaptic release of 5-HA [54]. Therefore, we deduced that SPS decreased the mRNA expression levels of TRP and 5-HIAA in the HPC, as well as the anxiety-like responses. TMP restored the hippocampal TRP and 5-HIAA expression levels, indicating that modulation of the 5-HT system plays a role in the anxiolytic effects of TMP. Thus, TMP acted like an anxiolytic, possibly by modifying the 5-HT system.
Furthermore, we observed an increase in the serum CORT, CRH, and ACTH levels compared with immediately before testing, indicating that SPS was sufficiently stressful [55]. Many studies have shown that SPS increased the serum CORT, CRH, and ACTH concentrations of rats, consistent with PTSD models [495657]. Accordingly, the forced maintenance of high CORT levels associated with animal models can affect anxiety-like symptoms under experimental conditions, and this might be relate to the progression or exacerbation of PTSD in humans [41]. Administration of TMP was associated with anxiolytic activity, suggesting that this therapy inhibited the HPA axis-associated psychological dysfunction induced by decreased serum CORT and ACTH levels, thereby normalizing the behavioral and neurochemical responses. Therefore, our results suggest that the effects of TMP was mediated by decreases in CORT and ACTH levels via the prevention of HPA axis dysfunction.
TMP has analgesic [58], anti-aging [59], antidiabetic [60], anti-inflammatory [61], anti-obesity [62], anti-oxidation [63], cholesterol-lowering [64], and neuroprotective [65] activities. Many studies continue to explore the physiochemical properties and biological activities of TMP and its structural derivatives, hoping to determine the medical potential of such compounds. As a traditional Asian medicine, TMP is generally considered safe for human consumption. In experimental animals, no apparent adverse effects were noted in rats treated for 6 weeks with daily doses of 20–40 mg/kg body weight of TMP, including weight loss, altered physical appearance, or behavioral changes [66]. Similarly, a TMP dose of 14.8 g/kg body weight did not induce any adverse effects [67]. In addition, many studies have suggested that TMP is rapidly absorbed and metabolized, mainly in its glucuronidated and sulfated forms, and then excreted in urine. TMP also exhibited superior pharmacological activities in vitro and in vivo [68]. Therefore, TMP is a potential candidate for treating different ailments due to its improved drug stability, increased bioavailability, and minimal side-effects.
Taken together, our data suggest that 5-HT is crucial after traumatic experiences or stress and that 5-HT hypofunction in the HPC appears to be useful for evaluation long-term adaptation following stress. Administration of TMP after SPS exposure significantly increased the time spent exploring the open arms on the EPM test, reduced the anxiety index, and decreased grooming behavior. Administration of TMP after SPS also significantly increased the number of central zone crossings during the OFT. Therefore, TMP was associated with anxiolytic-like effects in the OFT and EPM test, possibly by modifying the 5-HT system. These results suggest that 5-HT can ameliorate the neurochemical responses and psychological behaviors involved in anxiety. Our findings also indicate that TMP has a therapeutic effect on the PTSD-like stress response that was accompanied by modulation of the HPA axis. Therefore, 5-HT and the HPA axis may be alternative treatment routes for preventing the anxiety-like behaviors associated with PTSD. These results could lead to the development of novel therapeutics for treating PTSD.
Figures and Tables
Fig. 1
Experiments used to develop single prolonged stress (SPS)-induced anxiety-like behaviors, and tetramethylpyrazine (TMP) treatment in rats.
Different groups of rats (n=6 or 7 animals per group) were used for all experiments.
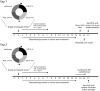
Fig. 2
Effects of TMP administration on the percentage of time spent in the open and closed arms, numbers of entries into the open and closed arms, anxiety index, number of unprotected head dips, and grooming behavior in the elevated plus maze (EPM) test of rats exposed to SPS.
*p<0.05, **p<0.01 vs. SAL group; #p<0.05, ##p<0.01 vs. PTSD group. ***p<0.001.
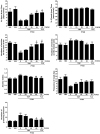
Fig. 3
Effects of TMP administration on locomotion and exploratory behavior in the open filed test (OFT) of rats exposed to SPS.
Changes in the time spent in the central and peripheral zones, numbers of crossing in the central and peripheral zones, locomotor activity, and number of rearing events in the open field test of rats exposed to SPS. *p<0.05, **p<0.01 vs. SAL group; #p<0.05, ##p<0.01 vs. PTSD group.
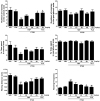
Fig. 4
Effects of TMP on freezing behavior from contextual fear conditioning testing after exposure to SPS in rats.
The percentage of time spent freezing was determined on acquisition, day 1, and day 2. **p<0.01 vs. SAL group; #p<0.05, ##p<0.01 vs. SPS group.
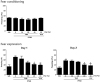
Fig. 5
Effects of TMP on the serotonin (5-HT) concentration in the brains of rats exposed to SPS.
*p<0.05, **p<0.01 vs. SAL group; #p<0.05, ##p<0.01 vs. PTSD group.
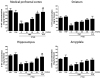
Fig. 6
Effects of TMP administration on the expression of tryptophan (TRP), 5-hydroxyindoleacetic acid (5-HIAA), and vesicular monoamine transporter-2 (VMAT-2) mRNA in rats during SPS-induced anxiety symptoms.
The polymerase chain reaction bands on an agarose gel and their relative intensities are indicated. The expression levels of TRH, 5-HIAA, and VMAT-2 mRNA were normalized to that of glyceraldehyde 3-phosphate dehydrogenase (GAPDH) mRNA as an internal control. *p<0.05, **p<0.01 vs. the SAL group, #p<0.05 vs. the PTSD group.
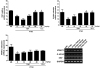
Fig. 7
Effects of TMP administration on the plasma corticosterone (CORT), corticotropin-releasing hormone (CRH), and adrenocorticotroic hormone (ACTH) levels in rats with SPS-induced anxiety-like symptoms analyzed by enzyme-linked immunosorbent assay.
**p<0.01 vs. the SAL group, #p<0.05 vs. the PTSD group.
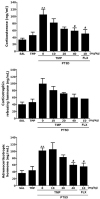
ACKNOWLEDGEMENTS
This research was supported by a Grant from the National Research Foundation of Korea funded by the Korean government (2016R1D1A1A09917012).
Notes
Author contributions B.L. and D.H.H. performed the conception and design. B.L. performed the carried out the experiments. B.L. and D.H.H. performed the acquisition of data. B.L. and D.H.H. performed the analysis and interpretation. B.L. performed the drafting the article. B.L. and D.H.H. performed the statistical analysis. I.S. and H.L. performed the study supervision.
References
1. Enman NM, Arthur K, Ward SJ, Perrine SA, Unterwald EM. Anhedonia, reduced cocaine reward, and dopamine dysfunction in a rat model of posttraumatic stress disorder. Biol Psychiatry. 2015; 78:871–879.


2. Wilson CB, McLaughlin LD, Ebenezer PJ, Nair AR, Francis J. Valproic acid effects in the hippocampus and prefrontal cortex in an animal model of post-traumatic stress disorder. Behav Brain Res. 2014; 268:72–80.


3. Ji LL, Tong L, Xu BK, Fu CH, Shu W, Peng JB, Wang ZY. Intra-hip pocampal administration of ZIP alleviates depressive and anxiety-like responses in an animal model of posttraumatic stress disorder. Behav Brain Funct. 2014; 10:28.
4. Rau V, DeCola JP, Fanselow MS. Stress-induced enhancement of fear learning: an animal model of posttraumatic stress disorder. Neurosci Biobehav Rev. 2005; 29:1207–1223.


5. Shin LM, Liberzon I. The neurocircuitry of fear, stress, and anxiety disorders. Neuropsychopharmacology. 2010; 35:169–191.


6. Wu ZM, Ni GL, Shao AM, Cui R. Genistein alleviates anxiety-like behaviors in post-traumatic stress disorder model through enhancing serotonergic transmission in the amygdala. Psychiatry Res. 2017; 255:287–291.


7. Lin CC, Tung CS, Liu YP. Escitalopram reversed the traumatic stress-induced depressed and anxiety-like symptoms but not the deficits of fear memory. Psychopharmacology (Berl). 2016; 233:1135–1146.


8. George SA, Stout SA, Tan M, Knox D, Liberzon I. Early handling attenuates enhancement of glucocorticoid receptors in the prefrontal cortex in an animal model of post-traumatic stress disorder. Biol Mood Anxiety Disord. 2013; 3:22–23.


9. Shea A, Walsh C, Macmillan H, Steiner M. Child maltreatment and HPA axis dysregulation: relationship to major depressive disorder and post traumatic stress disorder in females. Psychoneuroendocrinology. 2005; 30:162–178.


11. Yamamoto S, Morinobu S, Takei S, Fuchikami M, Matsuki A, Yamawaki S, Liberzon I. Single prolonged stress: toward an animal model of posttraumatic stress disorder. Depress Anxiety. 2009; 26:1110–1117.


12. Eskandarian S, Vafaei AA, Vaezi GH, Taherian F, Kashefi A, Rashidy-Pour A. Effects of systemic administration of oxytocin on contextual fear extinction in a rat model of post-traumatic stress disorder. Basic Clin Neurosci. 2013; 4:315–322.
13. Kohda K, Harada K, Kato K, Hoshino A, Motohashi J, Yamaji T, Morinobu S, Matsuoka N, Kato N. Glucocorticoid receptor activation is involved in producing abnormal phenotypes of single-prolonged stress rats: a putative post-traumatic stress disorder model. Neuroscience. 2007; 148:22–33.


14. Li H, Yang X, Shi W, Ma Z, Feng GK, Yin YL, Fan YX, Jiang J. Protective effects of tetramethylpyrazine on cerebrovascular regulations in rats with chronic alcoholic encephalopathy. Biomed Environ Sci. 2015; 28:691–695.
15. Cheng XR, Zhang L, Hu JJ, Sun L, Du GH. Neuroprotective effects of tetramethylpyrazine on hydrogen peroxide-induced apoptosis in PC12 cells. Cell Biol Int. 2007; 31:438–443.


16. Guan D, Su Y, Li Y, Wu C, Meng Y, Peng X, Cui Y. Tetramethylpyrazine inhibits CoCl2-induced neurotoxicity through enhancement of Nrf2/GCLc/GSH and suppression of HIF1a/NOX2/ROS pathways. J Neurochem. 2015; 134:551–565.
17. Wu W, Yu X, Luo XP, Yang SH, Zheng D. Tetramethylpyrazine protects against scopolamine-induced memory impairments in rats by reversing the cAMP/PKA/CREB pathway. Behav Brain Res. 2013; 253:212–216.


18. Ding Y, Hou X, Chen L, Li H, Tang Y, Zhou H, Zhao S, Zheng Y. Protective action of tetramethylpyrazine on the medulla oblongata in rats with chronic hypoxia. Auton Neurosci. 2013; 173:45–52.


19. Xu D, Chen H, Mak S, Hu S, Tsim KWK, Hu Y, Sun Y, Zhang G, Wang Y, Zhang Z, Han Y. Neuroprotection against glutamate-induced excitotoxicity and induction of neurite outgrowth by T-00, a novel multifunctional derivative of tetramethylpyrazine in neuronal cell models. Neurochem Int. 2016; 99:194–205.
20. Zhang H, Sun R, Liu XY, Shi XM, Wang WF, Yu LG, Guo XL. A tetramethylpyrazine piperazine derivate CXC137 prevents cell injury in SH-SY5Y cells and improves memory dysfunction of rats with vascular Dementia. Neurochem Res. 2014; 39:276–286.


21. Xiao X, Liu Y, Qi C, Qiu F, Chen X, Zhang J, Yang P. Neuroprotection and enhanced neurogenesis by tetramethylpyrazine in adult rat brain after focal ischemia. Neurol Res. 2010; 32:547–555.


22. Zhang C, Wang SZ, Zuo PP, Cui X, Cai J. Protective effect of tetramethylpyrazine on learning and memory function in D-galactose-lesioned mice. Chin Med Sci J. 2004; 19:180–184.
23. Zhang T, Gu J, Wu L, Li N, Sun Y, Yu P, Wang Y, Zhang G, Zhang Z. Neuroprotective and axonal outgrowth-promoting effects of tetramethylpyrazine nitrone in chronic cerebral hypoperfusion rats and primary hippocampal neurons exposed to hypoxia. Neuropharmacology. 2017; 118:137–147.


24. Lu F, Li X, Li W, Wei K, Yao Y, Zhang Q, Liang X, Zhang J. Tetramethylpyrazine reverses intracerebroventricular streptozotocin-induced memory deficits by inhibiting GSK-3b. Acta Biochim Biophys Sin (Shanghai). 2017; 49:722–728.
25. Li SY, Jia YH, Sun WG, Tang Y, An GS, Ni JH, Jia HT. Stabilization of mitochondrial function by tetramethylpyrazine protects against kainate-induced oxidative lesions in the rat hippocampus. Free Radic Biol Med. 2010; 48:597–608.


26. Liu HT, Du YG, He JL, Chen WJ, Li WM, Yang Z, Wang YX, Yu C. Tetramethylpyrazine inhibits production of nitric oxide and inducible nitric oxide synthase in lipopolysaccharide-induced N9 microglial cells through blockade of MAPK and PI3K/Akt signaling pathways, and suppression of intracellular reactive oxygen species. J Ethnopharmacol. 2010; 129:335–343.


27. Tan Z. Neural protection by naturopathic compounds-an example of tetramethylpyrazine from retina to brain. J Ocul Biol Dis Infor. 2009; 2:57–64.


28. Fan LH, Wang KZ, Cheng B, Wang CS, Dang XQ. Anti-apoptotic and neuroprotective effects of Tetramethylpyrazine following spinal cord ischemia in rabbits. BMC Neurosci. 2006; 7:48–56.


29. Shih YH, Wu SL, Chiou WF, Ku HH, Ko TL, Fu YS. Protective effects of tetramethylpyrazine on kainate-induced excitotoxicity in hippocampal culture. Neuroreport. 2002; 13:515–519.


30. Lee B, Sur B, Yeom M, Shim I, Lee H, Hahm DH. L-tetrahydropalmatine ameliorates development of anxiety and depression-related symptoms induced by single prolonged stress in rats. Biomol Ther (Seoul). 2014; 22:213–222.
31. Lee B, Sur B, Yeom M, Shim I, Lee H, Hahm DH. Effects of systemic administration of ibuprofen on stress response in a rat model of post-traumatic stress disorder. Korean J Physiol Pharmacol. 2016; 20:357–366.


32. Davies DR, Olson D, Meyer DL, Scholl JL, Watt MJ, Manzerra P, Renner KJ, Forster GL. Mild traumatic brain injury with social defeat stress alters anxiety, contextual fear extinction, and limbic monoamines in adult rats. Front Behav Neurosci. 2016; 10:71–80.


33. Eagle AL, Fitzpatrick CJ, Perrine SA. Single prolonged stress impairs social and object novelty recognition in rats. Behav Brain Res. 2013; 256:591–597.


34. Peng Y, Feng SF, Wang Q, Wang HN, Hou WG, Xiong L, Luo ZJ, Tan QR. Hyperbaric oxygen preconditioning ameliorates anxiety-like behavior and cognitive impairments via upregulation of thioredoxin reductases in stressed rats. Prog Neuropsychopharmacol Biol Psychiatry. 2010; 34:1018–1025.


35. Knox D, George SA, Fitzpatrick CJ, Rabinak CA, Maren S, Liberzon I. Single prolonged stress disrupts retention of extinguished fear in rats. Learn Mem. 2012; 19:43–49.


36. Yamamoto S, Morinobu S, Fuchikami M, Kurata A, Kozuru T, Yamawaki S. Effects of single prolonged stress and D-cycloserine on contextual fear extinction and hippocampal NMDA receptor expression in a rat model of PTSD. Neuropsychopharmacology. 2008; 33:2108–2116.


37. Khan S, Liberzon I. Topiramate attenuates exaggerated acoustic startle in an animal model of PTSD. Psychopharmacology (Berl). 2004; 172:225–229.


38. Hohlbaum K, Bert B, Dietze S, Palme R, Fink H, Thöne-Reineke C. Severity classification of repeated isoflurane anesthesia in C57BL/6JRj mice-Assessing the degree of distress. PLoS One. 2017; 12:e0179588.


39. Rau V, Oh I, Laster M, Eger EI 2nd, Fanselow MS. Isoflurane suppresses stress-enhanced fear learning in a rodent model of post-traumatic stress disorder. Anesthesiology. 2009; 110:487–495.


40. Shafia S, Vafaei AA, Samaei SA, Bandegi AR, Rafiei A, Valadan R, Hosseini-Khah Z, Mohammadkhani R, Rashidy-Pour A. Effects of moderate treadmill exercise and fluoxetine on behavioural and cognitive deficits, hypothalamic-pituitary-adrenal axis dysfunction and alternations in hippocampal BDNF and mRNA expression of apoptosis - related proteins in a rat model of post-traumatic stress disorder. Neurobiol Learn Mem. 2017; 139:165–178.


41. Serova LI, Laukova M, Alaluf LG, Sabban EL. Intranasal infusion of melanocortin receptor four (MC4R) antagonist to rats ameliorates development of depression and anxiety related symptoms induced by single prolonged stress. Behav Brain Res. 2013; 250:139–147.


42. Lever C, Burton S, O'Keefe J. Rearing on hind legs, environmental novelty, and the hippocampal formation. Rev Neurosci. 2006; 17:111–133.


43. Crusio WE. Genetic dissection of mouse exploratory behaviour. Behav Brain Res. 2001; 125:127–132.


44. Sturman O, Germain PL, Bohacek J. Exploratory rearing: a context- and stress-sensitive behavior recorded in the open-field test. Stress. 2018; 1–10.


45. Torres-Lista V, Parrado-Fernández C, Alvarez-Montón I, Frontiñán-Rubio J, Durán-Prado M, Peinado JR, Johansson B, Alcaín FJ, Giménez-Llort L. Neophobia, NQO1 and SIRT1 as premorbid and prodromal indicators of AD in 3xTg-AD mice. Behav Brain Res. 2014; 271:140–146.


46. Choi YJ, Kim JY, Jin WP, Kim YT, Lee JH, Jahng JW. Anxiolytic efficacy of repeated oral capsaicin in rats with partial aberration of oral sensory relay to brain. Arch Oral Biol. 2015; 60:989–997.


47. Chen LJ, Shen BQ, Liu DD, Li ST. The effects of early-life predator stress on anxiety- and depression-like behaviors of adult rats. Neural Plast. 2014; DOI: 10.1155/2014/163908.


48. Kabuki Y, Mizobe Y, Yamada S, Furuse M. Dietary l-tyrosine alleviates the behavioral alterations induced by social isolation stress in mice. Brain Res Bull. 2009; 80:389–396.


49. Kozlovsky N, Matar MA, Kaplan Z, Zohar J, Cohen H. The role of the galaninergic system in modulating stress-related responses in an animal model of posttraumatic stress disorder. Biol Psychiatry. 2009; 65:383–391.


50. Cryan JF, Holmes A. The ascent of mouse: advances in modelling human depression and anxiety. Nat Rev Drug Discov. 2005; 4:775–790.


51. Zhang XG, Zhang H, Liang XL, Liu Q, Wang HY, Cao B, Cao J, Liu S, Long YJ, Xie WY, Peng DZ. Epigenetic mechanism of maternal post-traumatic stress disorder in delayed rat offspring development: dysregulation of methylation and gene expression. Genet Mol Res. 2016; 15:1–10.


52. Wang HT, Han F, Shi YX. Activity of the 5-HT1A receptor is involved in the alteration of glucocorticoid receptor in hippocampus and corticotropin-releasing factor in hypothalamus in SPS rats. Int J Mol Med. 2009; 24:227–231.


53. Aykaç A, Aydın B, Cabadak H, Gören MZ. The change in muscarinic receptor subtypes in different brain regions of rats treated with fluoxetine or propranolol in a model of post-traumatic stress disorder. Behav Brain Res. 2012; 232:124–129.


54. Nikolaus S, Antke C, Beu M, Müller HW. Cortical GABA, striatal dopamine and midbrain serotonin as the key players in compulsive and anxiety disorders--results from in vivo imaging studies. Rev Neurosci. 2010; 21:119–139.


55. Castro JE, Diessler S, Varea E, Márquez C, Larsen MH, Cordero MI, Sandi C. Personality traits in rats predict vulnerability and resilience to developing stress-induced depression-like behaviors, HPA axis hyper-reactivity and brain changes in pERK1/2 activity. Psychoneuroendocrinology. 2012; 37:1209–1223.


56. Meyer EM, Long V, Fanselow MS, Spigelman I. Stress increases voluntary alcohol intake, but does not alter established drinking habits in a rat model of posttraumatic stress disorder. Alcohol Clin Exp Res. 2013; 37:566–574.


57. Roth MK, Bingham B, Shah A, Joshi A, Frazer A, Strong R, Morilak DA. Effects of chronic plus acute prolonged stress on measures of coping style, anxiety, and evoked HPA-axis reactivity. Neuropharmacology. 2012; 63:1118–1126.


58. Leng YF, Gao XM, Wang SX, Xing YH. Effects of tetramethylpyrazine on neuronal apoptosis in the superficial dorsal horn in a rat model of neuropathic pain. Am J Chin Med. 2012; 40:1229–1239.


59. Gao B, Lin X, Jing H, Fan J, Ji C, Jie Q, Zheng C, Wang D, Xu X, Hu Y, Lu W, Luo Z, Yang L. Local delivery of tetramethylpyrazine eliminates the senescent phenotype of bone marrow mesenchymal stromal cells and creates an anti-inflammatory and angiogenic environment in aging mice. Aging Cell. 2018; 17:e12741.


60. Yang QH, Liang Y, Xu Q, Zhang Y, Xiao L, Si LY. Protective effect of tetramethylpyrazine isolated from Ligusticum chuanxiong on nephropathy in rats with streptozotocin-induced diabetes. Phytomedicine. 2011; 18:1148–1152.


61. Kim M, Kim SO, Lee M, Lee JH, Jung WS, Moon SK, Kim YS, Cho KH, Ko CN, Lee EH. Tetramethylpyrazine, a natural alkaloid, attenuates pro-inflammatory mediators induced by amyloid b and interferon-g in rat brain microglia. Eur J Pharmacol. 2014; 740:504–511.
62. Zhang Y, Ren P, Kang Q, Liu W, Li S, Li P, Liu H, Shang J, Zhang L, Gong Y, Zhou M. Effect of tetramethylpyrazine on atherosclerosis and SCAP/SREBP-1c signaling pathway in ApoE-/- mice fed with a high-fat diet. Evid Based Complement Alternat Med. 2017; DOI: 10.1155/2017/3121989.
63. Zhao X, Jiang J, Yang G, Huang J, Yang G, He G, Chu Z, Hang T, Fan G. Profiling and preparation of metabolites from pyragrel in human urine by online solid-phase extraction coupled with high performance liquid chromatography tandem mass spectrometry followed by a macroporous resin-based purification approach. Molecules. 2017; 22:E494.


64. Wang GF, Shi CG, Sun MZ, Wang L, Wu SX, Wang HF, Xu ZQ, Chen DM. Tetramethylpyrazine attenuates atherosclerosis development and protects endothelial cells from ox-LDL. Cardiovasc Drugs Ther. 2013; 27:199–210.


65. Chen L, Cheng L, Wei X, Yuan Z, Wu Y, Wang S, Ren Z, Liu X, Liu H. Tetramethylpyrazine analogue CXC195 protects against dopaminergic neuronal apoptosis via activation of PI3K/Akt/GSK3β signaling pathway in 6-OHDA-induced Parkinson's disease mice. Neurochem Res. 2017; 42:1141–1150.


66. Jiang Y, Liu C, Chen W, Wang H, Wang C, Lin N. Tetramethylpyrazine enhances vascularization and prevents osteonecrosis in steroid-treated rats. Biomed Res Int. 2015; DOI: 10.1155/2015/315850.


67. Moon SK, Kim YS, Park SU, Jung WS, Ko CN, Cho KH, Bae HS. Effect of gastrodia elata BL water extract on human cerebral blood flow using transcranial doppler. Korean Oriental Medicine. 2005; 26:115–122.
68. Guo B, Xu D, Duan H, Du J, Zhang Z, Lee SM, Wang Y. Therapeutic effects of multifunctional tetramethylpyrazine nitrone on models of Parkinson’s disease in vitro and in vivo. Biol Pharm Bull. 2014; 37:274–285.