Abstract
The present study was carried out with the hypothesis that combination of canagliflozin and omega-3 fatty acid may have potential effect on insulin level, insulin resistance, cardiac biomarkers, inflammatory cytokines and histological studies in type 2 diabetes mellitus (DM). Type 2 DM was induced by injecting nicotinamide (120 mg/kg, i.p.) 15 min before STZ (60 mg/kg) injection. Canagliflozin (5 and 10 mg/kg) and omega-3 fatty acid (300 mg/kg) were given for 28 days after confirmation of diabetes. Biochemical estimations revealed elevated levels of glucose, insulin, HOMA-R and inflammatory cytokines in diabetic group. Daily dosing of alone canagliflozin and omega-3 fatty acid slightly reduced elevated levels of glucose, insulin, HOMA-R and inflammatory cytokines (IL-1β, IL-2, and TNFα), whereas canagliflozin and omega-3 fatty acid combination has reduced these biochemical parameters significantly when compared with diabetic group. Similarly in diabetic group the levels of cardiac biomarkers such as lipid profile, LDH, CKMB and troponin were significantly increased. Elevated levels of cardiac biomarkers were significantly reduced after daily dosing of alone canagliflozin and omega-3 fatty acid. Canagliflozin and omega-3 fatty acid combination has offered better improvement in cardiac biomarkers compared to alone canagliflozin and omega-3 fatty acid. Histopathological analysis also supported the above hypothesis that combination therapy (canagliflozin and omega-3 fatty acid) offered better protection against degenerative changes in β-cells of pancreas as compared to alone treatment with these drugs. Thus the present study revealed that canagliflozin and omega-3 fatty acid can be used as potential combination therapy in type 2 DM along with cardiac complication.
Diabetes mellitus (DM) was considered as an illness of low significance to world health a few decades ago, but now it is rising as a major threat for the health of human beings currently [1]. The International Diabetes Federation, Diabetes Atlas [2], have reported approximately 366 million people have diabetes in 2011 and by 2030 this will increase to 552 million. The prevalence of type 2 DM in Saudi Arabia is 27% in females and 34% in males according to recent study conducted among Saudi Community patients attending a primary health care clinic [3]. Prevalence of it is growing throughout the world and is attracting the attention of health community and researchers. Several epidemiological studies confirmed that it is 4th or 5th leading causes of death in developed countries. Previous studies showed that the possibility of developing cardiovascular disease (CVD) in diabetes is 2–3 times higher in men compared with 3–5 times higher in women [45]. Cardiovascular complication is the foremost cause of mortality in both types of DM [6]. It is considered to be one of the most worrying problems for the society and health care system. Type 2 DM often requires combination therapy with antihyperglycemic and antihypertensive agents as the disease progresses.
Cytokines are cluster of proteins (or glycoproteins) that are produced by the effector cells of the body. They are the category of signaling molecules that mediate cell to cell communication by autocrine, paracrine and endocrine in action that regulates inflammatory reactions. The inflammatory regulation of cytokines is elicited by pro-inflammatory and anti-inflammatory effector pathway. Cytokines are the key factors responsible for the occurrence of DM, which is characterized either as insulin resistance or insulin insufficiency [78]. Studies suggesting that the onset of effector pathway of cytokine network is involved in the development of diabetes a year before the onset [9]. The available records showed that inflammatory cytokines are the causative factor and play an important role in the occurrence of insulin resistance (IR) and cardiovascular complications in diabetes [10].
Fish and marine life are the most important natural sources of omega-3 fatty acids which belong to special class of polyunsaturated fatty acids (PUFA). Previous studies showed that omega-3 fatty acid has antioxidant and lipid lowering effect, suggesting a possible beneficial effect in managing type 2 DM and its complications [11]. Recently Eissa et al. [12] reported that the combination of omega-3 fatty acid and pioglitazone significantly improved insulin resistance in type 2 DM.
Canagliflozin represent a new class of drugs which inhibit sodium glucose co-transporter 2 (SGLT2) in renal tubule, reduces reabsorption of glucose in renal tubule and thus increases its urinary excretion [13]. SGLT2 inhibitors have other cardiometabolic effects such as natriuretic and mild osmotic diuretics, which contribute to reduction in blood pressure and total body weight. It is marketed as an adjunct therapy for adults with type 2 DM. Effect of canagliflozin on insulin secretion, insulin resistance, inflammatory cytokines and cardiovascular complications in type 2 DM remains to be investigated. Therefore, the present research work was designed with the hypothesis that combination of canagliflozin and omega-3 fatty acid has any beneficial effect on insulin secretion, insulin resistance, cardiac biomarkers and inflammatory cytokines in type 2 DM.
Albino Wistar rats (200–250 g) were purchased from the Medical Research Centre (MRC), Jazan University. The rats were brought from the MRC and housed in the animal room of College of Pharmacy for one week for acclimatization. They were housed under ideal laboratory condition with 12 h light/dark cycle, received standard basal diet, water ad-libitum and maintained at temperature of 25±2℃. The experimental study was performed as per the International guidelines for the care and use of laboratory animals [14].
Streptozotocin (STZ) and nicotinamide was procured from Sigma Chemicals USA. Soft gelatin capsule of omega-3 fatty acid was purchased from local Pharmacy, which is manufactured by Jamjoom Pharmaceuticals, Saudi Arabia. Canagliflozin (Invokana) tablet was also purchased from the same Pharmacy, which is manufactured by Janseen Research & Development, USA. All other chemicals and kits used in the experiment were procured from different manufacturers through certified local supplier.
Type 2 DM was induced by injecting nicotinamide (120 mg/kg, i.p) 15 min prior to the injection of STZ (60 mg/kg, i.p) [15]. Diabetes was confirmed by measuring glucose after 3 days (or 72 h) of STZ+nicotinamide injection. Those animals showed glucose level 200 mg/dl or more were considered to be diabetic and divided into different groups.
Forty two Wistar rats were divided randomly into 7 groups with each group having 6 animals. Group-I was normal control, given vehicle only (CMC, 1 mg/kg). Group-II was diabetic control, given STZ+nicotinamide. Group-III was diabetic treated, given only omega-3 fatty acid (300 mg/kg). Group-IV was diabetic treated, given lower dose of canagliflozin (5 mg/kg). Group-V was diabetic treated, given higher dose of canagliflozin (10 mg/kg). Group-VI was diabetic treated, given lower dose of canagliflozin (5 mg/kg) and omega-3 fatty acid (300 mg/kg). Group-VII was also diabetic treated, given higher dose of canagliflozin (10 mg/kg) and omega-3 fatty acid (300 mg/kg). Omega-3 fatty acid was taken from soft gelatine capsule with the help of syringe and administered orally using oral feeding needle. Canagliflozin tablet was crushed into powder and suspended in water using carboxymethylcellulose (CMC) as suspending agent. It was administered orally with the help of oral feeding needle in two different doses (5 and 10 mg/kg). Both drugs were given for 4 weeks (28 days) and on last day blood was withdrawn and serum was separated for biochemical estimations.
Glucose: Glucose was measured in serum by the method of glucose oxidase [16] using Crescent diagnostics kit, Jeddah, Saudi Arabia.
Insulin: The insulin was estimated by simple step sandwich ELISA technique. The free insulin is quantified by end point determination. The end point was estimated by the development of yellow colour which was measured at 450 nm by using Bio Tek EL X 800 ELISA reader. The sample concentration was calculated by extrapolating on the standard curve.
Insulin resistance was determined by Mathews et al. [17] with the help of HOMA-R equation:
HOMA-R=Glucose (mM) fasting×insulin (mU/L) fasting /22.5. Glucose conversion factor was used to convert mg/dl into mM (18 mg/dl=1 mM).
Serum was used for the determination of various lipid marker parameters such as triglycerides (TG) [18], total cholesterol (TCh) [19] and high density lipoprotein cholesterol (HDL-C) [20] using Crescent Diagnostics kits, KSA. The value of low density lipoprotein cholesterol (LDL-C) was calculated according to the Friedwald equation [21]. Lactate dehydrogenase (LDH) was estimated in serum by simple colorimetric method using Abcam's assay kit [22]. NAD is reduced to NADH by LDH, which further interacts with specific probe to produce colour. The development of colour formation was estimated at 450 nm. Creatine kinase MB was estimated by simple in vitro sandwich ELISA using Abcam's assay kit. Standards and test samples were analyzed by binding with immobilized antibody which captures CKMB present in the test sample. The development of colour formation was estimated at 450 nm using Bio Tek EL X 800 ELISA reader. Cardiac troponin I was quantitatively measured in serum by simple in vitro sandwich ELISA. This method utilizes an affinity tag antibody and a reporter conjugated antibody which immunocapture the standard and sample present in solution. The end reaction was measured by the development of colour formation which was estimated at 450 nm using Bio Tek EL X 800 ELISA reader.
IL1-β, IL-2 and TNFα were assessed by using simple sandwich ELISA technique using Abcam's assay kit. The development of yellow colour end point was considered as cytokine concentration. The end point was estimated by the development of yellow colour which was measured at 450 nm by using Bio Tek EL X 800 ELISA reader. The sample concentration was calculated by extrapolating on the standard curve.
The isolated pancreas was properly washed with ice cold saline and it was fixed in 10% formalin solution. The pancreas was embedded in paraffin and sectioned into 3–5 µm thick with the help of microtome. The sliced sections were stained with hematoxylin and eosin (H&E) and the degree of β-cell damage was evaluated using light microscope.
The results were documented for each parameter after the experimental study and were analyzed statistically. Results of each group are represented as standard error means (S.E.M). The statistical method used to find the significant difference among group was ANOVA followed by Tukey's multiple comparison. A p value of less than 0.05 was considered to be significant.
Fig. 1 shows that treatment with STZ+nicotinamide significantly (p<0.001) increased the glucose level in group II (diabetic) compared to group I (normal). Administration of two doses of canagliflozin (5 and 10 mg/kg) and omega-3 fatty acid (300 mg/kg) for 28 days resulted in significant (p<0.001) decrease in glucose levels compared to group II (diabetic). The combination of canagliflozin and omega-3 fatty acid has also reduced the glucose levels significantly.
Result of canagliflozin and omega-3 fatty acid on insulin level is shown in Fig. 2. Insulin level was significantly (p<0.001) increased in group II (diabetic) after STZ+nicotinamide administration compared to group I (normal). No significant change in the insulin level was observed in the lower dose of canagliflozin (5 mg/kg). Treatment with higher dose of canagliflozin (10 mg/kg), omega-3 fatty acid (300 mg/kg) and its combination significantly (p<0.001) reduced the higher insulin levels compared to group II (diabetic).
Fig. 3 represents the value of HOMA-R, which is an insulin resistance index. There was significant (p<0.001) rise in HOMA-R level in group II (diabetic) compared to group I (normal). Administration of two doses of canagliflozin (5 & 10 mg/kg), omega-3 fatty acid (300 mg/kg) and their combinations significantly (p<0.001) prevented the rise in HOMA-R levels compared to group II (diabetic).
Table 1 illustrates the result of canagliflozin (5 and 10 mg/kg) and omega-3 fatty acid (300 mg/kg) on lipid profile. The levels of TCh, TG and LDL-C were significantly (p<0.001) elevated and HDL-C was significantly (p<0.001) decreased in STZ+nicotinamide treated group II (diabetic) compared to normal group. Two different doses of canagliflozin (5 and 10 mg/kg), omega-3 fatty acid (300 mg/kg) and their combinations resulted in significant (p<0.001) decrease in serum TCh, TG, LDK-C and LDL-C compared to diabetic group. In contrast significant increase in HDL-C level was observed after canagliflozin and omega-3 fatty acid treatment compared to diabetic group.
Assay of inflammatory cytokines such as IL-1β, IL-2, and TNFα are depicted in Table 2. STZ+nicotinamide administration to group II (diabetic) showed significant (p<0.001) upsurge in the levels of IL-1β, IL-2, and TNFα compared to group I (normal) rats. Treatment with canagliflozin (5 and 10 mg/kg), omega-3 fatty acid (300 mg/kg) and their combination significantly (p<0.001) restored the elevated levels of above mentioned cytokines compared to group II (diabetic).
STZ and nicotinamide treatment to group II (diabetic) has significantly (p<0.001) increased LDH and CKMB levels as compared to group I (normal). Administration of canagliflozin (5 and 10 mg/kg), omega-3 fatty acid (300 mg/kg) and its combination has reduced the levels of LDH and CKMB significantly (p<0.001) compared to group II (diabetic) which is presented in Figs. 4 and 5. High sensitive troponin I level was not detected in group I (normal). The level of troponin I in STZ and nicotinamide treated diabetic group was significantly (p<0.001) high compared with other treated groups. No significant change in the level of troponin I was seen after the treatment with only omega-3 fatty acid, whereas significant (p<0.001) reduction was observed after the administration of canagliflozin and omega-3 fatty acid combination compared to group II (diabetic) which is shown in Fig. 6.
The section of pancreas in group I (normal) showed normal architecture of pancreatic acini and β-cells of islet (Fig. 7A). Degenerative and necrotic changes in pancreatic acini and β-cells of pancreas were seen in group II (diabetic) with reduction in the size of β-cells (Fig. 7B). The degenerative and necrotic changes in the β-cells were not significantly improved after treatment with only omega-3 fatty acid (Fig. 7C), whereas these degenerative and necrotic changes in the β-cells of islet was slightly improved in type 2 diabetic rats treated with only canagliflozin (Figs. 7D and E). The combination of omega-3 fatty acid and canagliflozin treatment showed significant improvement in the degenerative and necrotic changes in pancreatic acini and regeneration of β-cells of islets (Figs. 7F and G) which revealed better protective effect.
Type 2 DM is a serious condition which has a direct effect on the individual and the whole society. It appear throughout the world, but a type of it i.e. type 2 DM is more common in developed countries. Its prevalence is growing throughout the world and is attracting the attention of health community and researchers. As we know, there is no therapy which can permanently cure diabetes. Despite advances in the development of antihyperglycemic drugs greater attention is given in diabetes with cardiovascular disease. Diabetic patients are four to five times more likely to develop heart diseases and stroke compared to non-diabetic patients [23].
Type 2 DM along with chronic hyperglycemia is frequently associated with the state of insulin resistance (IR). Pancreatic β-cells secrete more insulin to counteract IR and to maintain glucose homeostasis. This increased level of circulating insulin is known as hyperinsulinemia, which is a compensatory process in response to high glucose level [24]. Previous studies have indicated that hyperinsulinemia is linked with hypersecretion of insulin from β cells, reduced hepatic clearance and post-receptor pathway desensitization [2526]. Yolanda and Adriana [27] have reported that polyunsaturated fatty acids ameliorated hyperlipidemia and IR. Our results clearly showed that the levels of glucose and insulin were significantly higher in diabetic control group. Daily administration of canagliflozin and omega-3 fatty acid for 28 days significantly reduced glucose and insulin levels, thereby preventing hyperglycemia and hyperinsulinemia. The combination of canagliflozin and omega-3 fatty acid counteracted hyperglycemia and hyperinsulinemia better than alone omega-3 fatty acid and canagliflozin. HOMA-R was calculated to determine the significance of IR. The results of present study demonstrated that treatment with canagliflozin, omega-3 fatty acid and their combinations significantly prevented the rise in HOMA-R level which was significantly higher in diabetic control group.
Inflammatory cytokines are predominant factor in the progression of diabetes mellitus. The estimation of inflammatory cytokines such as IL1-β, IL-2 and TNFα serve as an important marker to diagnose diabetes mellitus [7]. Interleukin-1β (IL-1β) and TNFα has been reported to alter insulin sensitivity by stimulating phosphorylation of serine residue instead of tyrosine residue in insulin receptor substrate (IRS), thus inhibit insulin signaling pathway and maintain insulin resistance [28]. Recent reports showed IL-1β has been reported to contribute β-cell failure that leads to macrophages stimulation. It has been suggested that IL-1β triggers nuclear factor kappa β (1kβ) kinase β and I – kappa α (Ikkα) to stimulate nuclear factor kappa β (NF-kβ) which promote insulin resistance [29]. In this work, there was increased IL-1β level after STZ+nicotinamide treatment and the level was decreased significantly after the treatment with alone canagliflozin and omega-3 fatty acid as well as their combinations when compared to diabetic group.
IL-2 is a dual functional cytokine exerts as pro-inflammatory effect by recruiting CD8+ cells as well as anti-inflammatory property by stimulating CD4+ cells. It exhibits both autocrine and paracrine action. Studies suggesting that IL-2 also encourage that natural killer (NK) cells increase the induction of cytotoxic activity. The IL-2 level exerts regulation by IL-2 gene by the transcriptional factor B lymphocyte induced maturation protein 1 (BLIMP 1) [30]. It is very interesting to note that IL-2 level was reduced after the treatment with alone canagliflozin either at 5 and 10 mg/kg and omega-3 fatty acid. The combination of canagliflozin and omega-3 fatty acid showed better result in controlling the level of IL-2 than alone indicating the regulation of IL-2. Thus combination of canagliflozin and omega-3 fatty acid is enhancing antiinflammatory property rather than pro-inflammatory response.
TNFα is a potent inflammatory cytokine and prime mediator of inflammatory cell damage. It is an adipocytokine derived from macrophages that has been concerned in the progress of insulin resistance. TNF-α has a role in regulating apoptosis and inflammatory processes in diabetes by stimulating CD8+ cells. The activation of inflammatory pathways by TNFα creates a state of insulin resistance in proportionate of glucose uptake by myocytes and adipocytes that damage insulin signaling at the receptor level. In this study the TNFα level significantly reduced when the animals were treated with canagliflozin alone or in combination with omega-3 fatty acid either with 5 mg or 10 mg. Thus, canagliflozin is preventing the cellular damage which is an important advantage to control the development and progression of diabetes mellitus with omega-3 fatty acid. Earlier report showed that the levels of the inflammatory cytokines were high in clinically diagnosed type 2 DM [31]. In this study, the results have been well demonstrating the role of combination of canagliflozin and omega-3 fatty acid in protecting cell. Canagliflozin either of its own or in combination with omega-3 fatty acid treatment exhibit a potent action to reduce IL-1β, IL-2 and TNFα level to near normal levels indicating the protective role in type 2 DM.
Cardiovascular complications such as myocardial infarction, stroke and peripheral vascular diseases are the foremost causes of death amongst both types of diabetic patients (type 1 and 2 DM) [23]. This complication may be protected by the consumption of a polyunsaturated fatty acid known as omega-3. It is essential for the human diet and play very important role in the management of various diseases. Daily uses of omega-3 fatty acids showed significant role in suppressing the risk factors of cardiovascular diseases and very effective in controlling glycemic index [3233]. Recent study showed that canagliflozin reduces fasting and post prandial glucose, control HbA1c and blood pressure with decrease in body weight [34]. American Diabetes Association (ADA) recommended strongly for the management of lipid abnormalities in type 2 diabetic patients to reduce the incidence of cardiovascular complications [1]. Inflammatory cytokines are associated in the development of CVDs and lipid abnormalities. Previous report showed that systemic inflammation and atherogenic factors are responsible for hypertriglyceridemia [35]. The increased levels of TGs and cholesterol could be due to over production of inflammatory cytokines which stimulate hepatic lipogenesis. Feingold and Grunfeld have reported that TNFα administration accelerate hepatic lipogenesis and increases TG and cholesterol [36]. Thus it is proved that TNF-α play an important role in lipid metabolism through modulation of adipose tissue, lipoprotein lipase activity and fatty acid synthesis in liver. Our results clearly exhibited that STZ+nicotinamide treated diabetic rats augmented TCh, TG and LDL levels and reduced level of HDL in diabetic control group. The objective of the therapy was to reduce TCh, TG and LDL and increase HDL to overcome the problem associated with CVDs. Administration of canagliflozin, omega-3 fatty acid and their combination to diabetic treated groups normalized the lipid profile by reducing TCh, TG and LDL levels and augmenting HDL levels.
Troponin, LDH and CKMB are frequently used as a biomarkers for myocardial damage which may account as a prognostic value for cardiovascular death. Earlier report suggests that these cardiac biomarkers are increased in diabetic patients [37]. Cardiovascular problem was assessed by measuring the levels of cardiac biomarkers such as LDH, CKMB and high sensitive troponin I in this study. STZ + nicotinamide treated diabetic significantly increased levels of cardiac biomarkers (LDH, CKMB and troponin I), thus showing sign of myocardial damage. Administration of canagliflozin, omega-3 fatty acid and their combination reestablished the elevated levels of LDH, CKMB and high sensitive troponin I, demonstrating myocardial protection. The decreased levels of LDH, CKMB and high sensitive troponin I might be due to increased levels of inflammatory cytokines. The present study revealed that the levels of inflammatory cytokines were reduced to near normal level after the administration of only canagliflozin, omega-3 fatty acid and their combination reduces the levels of LDH, CKMB and troponin I compared to diabetic group.
The protective effect of the combination of canagliflozin and omega-3 fatty acid is probably due to the amelioration of insulin resistance, improvement of cardiac biomarkers and counteraction of elevated levels of inflammatory cytokines which is also confirmed from the histological findings of pancreas. Type 2 diabetic control rats showed significant reduction in size and number of pancreatic β-cells. Only canagliflozin treated rats demonstrate slight increase in the size of pancreatic β-cells, which was not observed with only omega-3 fatty acid treatment. However, treatment with the combination of canagliflozin and omega-3 fatty acid offered better protection as evidenced by normal pancreatic acini and increase in the size of β-cells of islets possibly due to regeneration.
Figures and Tables
Fig. 1
Effect of canagliflozin and omega-3 fatty acid on glucose levels in STZ+nicotinamide treated diabetic rats.
##p<0.001 versus group I (normal), **p<0.001 versus group II (diabetic).
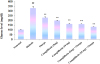
Fig. 2
Effect of canagliflozin and omega-3 fatty acid on insulin levels in STZ+nicotinamide treated diabetic rats.
##p<0.001 versus group I (normal), nsp>0.05, **p<0.001 versus group II (diabetic).
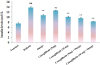
Fig. 3
Effect of canagliflozin and omega-3 fatty acid on HOMA-R levels in STZ+nicotinamide treated diabetic rats.
##p<0.001 compared with group I (normal), **p<0.001 compared with group II (diabetic).
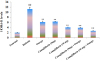
Fig. 4
Effect of canagliflozin and omega-3 fatty acid on LDH levels in STZ+nicotinamide treated diabetic rats.
##p<0.001 versus group I (normal), *p<0.01, **p<0.001 versus group II (diabetic).

Fig. 5
Effect of canagliflozin and omega-3 fatty acid on CKMB levels in STZ+nicotinamide treated diabetic rats.
##p<0.001 compared with group I (normal), **p<0.001 compared with group II (diabetic).
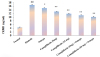
Fig. 6
Effect of canagliflozin and omega-3 fatty acid on troponin I levels in STZ+nicotinamide treated diabetic rats.
Group-I – not detected (ND), nsp>0.05, **p<0.001 compared with group II (diabetic).
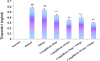
Fig. 7
Effect of canagliflozin and omega-3 fatty acid on histology of pancreatic β-cells.
Hematoxylin and eosin-stained sections of rat pancreas: (A) represents group I (normal) rat showing normal lobules of pancreatic acini and β-cells. (B) represents group II (diabetic) rat showing lobules of pancreatic acini with areas of fibrosis and depletion of β-cells. (C) represents pancreatic section of omega-3 fatty acid treated rat showing fibrosis of pancreatic acini with degenerated β-cells. (D, E) indicate pancreatic section of only canagliflozin treated rat showing mild fibrosis of pancreatic acini with slight increase in the size of β-cells. (F, G) indicate pancreatic section of combination of canagliflozin and omega-3 fatty acid showing normal lobules of pancreatic acini and β-cells.
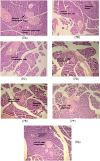
ACKNOWLEDGEMENTS
We are thankful to the Deanship of Scientific Research, Jazan University, Gizan, KSA, for financial support. We are also thankful to the College of Pharmacy for providing animal house and research laboratory to accomplish this research work.
Notes
References
1. American Diabetes Association. Diagnosis and classification of diabetes mellitus. Diabetes Care. 2014; 37:Suppl 1. S81–S90.
2. IDF Diabetes Atlas 5th edition [Internet]. International Diabetes Federation;2011. p-7 https://www.idf.org/e-library/epidemiology-research/diabetes-atlas/20-atlas-5th-edition.html.
3. Alqurashi KA, Aljabri KS, Bokhari SA. Prevalence of diabetes mellitus in a Saudi community. Ann Saudi Med. 2011; 31:19–23.


4. Zimmet P, Thomas CR. Genotype, obesity and cardiovascular disease-has technical and social advancement outstripped evolution? J Intern Med. 2003; 254:114–125.
5. Barrett-Connor EL, Cohn BA, Wingard DL, Edelstein SL. Why is diabetes mellitus a stronger risk factor for fatal ischemic heart disease in women than in men? The Rancho Bernardo Study. JAMA. 1991; 265:627–631.


6. Trost S, LeWinter M. Diabetic cardiomyopathy. Curr Treat Options Cardiovasc Med. 2001; 3:481–492.


7. Alexandraki K, Piperi C, Kalofoutis C, Singh J, Alaveras A, Kalofoutis A. Inflammatory process in type 2 diabetes: The role of cytokines. Ann N Y Acad Sci. 2006; 1084:89–117.


8. Banerjee M, Saxena M. Interleukin-1 (IL-1) family of cytokines: role in type 2 diabetes. Clin Chim Acta. 2012; 413:1163–1170.


9. Dandona P, Aljada A, Bandyopadhyay A. Inflammation: the link between insulin resistance, obesity and diabetes. Trends Immunol. 2004; 25:4–7.


10. Mahmoud F, Al-Ozairi E. Inflammatory cytokines and the risk of cardiovascular complications in type 2 diabetes. Dis Markers. 2013; 35:235–241.


11. Lee MW, Park JK, Hong JW, Kim KJ, Shin DY, Ahn CW, Song YD, Cho HK, Park SW, Lee EJ. Beneficial effects of omega-3 fatty acids on low density lipoprotein particle size in patients with type 2 diabetes already under statin therapy. Diabetes Metab J. 2013; 37:207–211.


12. Eissa LA, Abdel-Rahman N, Eraky SM. Effects of omega-3 fatty acids and pioglitazone combination on insulin resistance through fibroblast growth factor 21 in type 2 diabetes mellitus. Egypt J Basic Appl Sci. 2015; 2:75–86.


13. Hasan FM, Alsahli M, Gerich JE. SGLT inhibitors in the treatment of type 2 diabetes. Diabetes Res Clin Pract. 2014; 104:297–322.
14. NRCC (National Research Council). Guide for the care and use of laboratory animals. 7th ed. Washington DC: National Academy Press;1996.
15. Shirwaikar A, Rajendran K, Punitha IS. Antidiabetic activity of alcoholic stem extract of Coscinium fenestratum in streptozotocin-nicotinamide induced type 2 diabetic rats. J Ethnopharmacol. 2005; 97:369–374.


16. Barham D, Trinder P. An improved colour reagent for the determination of blood glucose by the oxidase system. Analyst. 1972; 97:142–145.


17. Matthews DR, Hosker JP, Rudenski AS, Naylor BA, Treacher DF, Turner RC. Homeostasis model assessment: insulin resistance and beta-cell function from fasting plasma glucose and insulin concentrations in man. Diabetologia. 1985; 28:412–419.
18. Bucolo G, David H. Quantitative determination of serum triglycerides by the use of enzymes. Clin Chem. 1973; 19:476–482.


19. Wybenga DR, Pileggi VJ, Dirstine PH, Di Giorgio J. Direct manual determination of serum total cholesterol with a single stable reagent. Clin Chem. 1970; 16:980–984.


20. Warnick GR, Nguyen T, Albers AA. Comparison of improved precipitation methods for quantification of high-density lipoprotein cholesterol. Clin Chem. 1985; 31:217–222.


21. Friedewald WT, Levy RI, Fredrickson DS. Estimation of the concentration of low-density lipoprotein cholesterol in plasma, without use of the preparative ultracentrifuge. Clin Chem. 1972; 18:499–502.


22. Vanderlinde RE. Measurement of total lactate dehydrogenase activity. Ann Clin Lab Sci. 1985; 15:13–31.
23. Yki-Järvinen H. Management of type 2 diabetes mellitus and cardiovascular risk: lessons from intervention trials. Drugs. 2000; 60:975–983.
24. Goldstein BJ. Insulin resistance as the core defect in type 2 diabetes mellitus. Am J Cardiol. 2002; 90:3G–10G.


25. Bonora E, Zavaroni I, Coscelli C, Butturini U. Decreased hepatic insulin extraction in subjects with mild glucose intolerance. Metabolism. 1983; 32:438–446.


26. Olefsky JM, Revers RR, Prince M, Henry RR, Garvey WT, Scarlett JA, Kolterman OG. Insulin resistance in non-insulin dependent (type II) and insulin dependent (type I) diabetes mellitus. Adv Exp Med Biol. 1985; 189:176–205.


27. Lombardo YB, Chicco AG. Effects of dietary polyunsaturated n-3 fatty acids on dyslipidemia and insulin resistance in rodents and humans. A review. J Nutr Biochem. 2006; 17:1–13.


28. Fasshauer M, Paschke R. Regulation of adipocytokines and insulin resistance. Diabetologia. 2003; 46:1594–1603.


29. McArdle MA, Finucane OM, Connaughton RM, McMorrow AM, Roche HM. Mechanisms of obesity-induced inflammation and insulin resistance: insights into the emerging role of nutritional strategies. Front Endocrinol (Lausanne). 2013; 4:52.


30. Boyman O, Sprent J. The role of interleukin-2 during homeostasis and activation of the immune system. Nat Rev Immunol. 2012; 12:180–190.


31. Weisberg SP, McCann D, Desai M, Rosenbaum M, Leibel RL, Ferrante AW Jr. Obesity is associated with macrophage accumulation in adipose tissue. J Clin Invest. 2003; 112:1796–1808.


32. Endo J, Arita M. Cardioprotective mechanism of omega-3 polyunsaturated fatty acids. J Cardiol. 2016; 67:22–27.


33. De Caterina R, Madonna R, Bertolotto A, Schmidt EB. n-3 fatty acids in the treatment of diabetic patients: biological rationale and clinical data. Diabetes Care. 2007; 30:1012–1026.


34. Bode B, Stenlöf K, Sullivan D, Fung A, Usiskin K. Efficacy and safety of canagliflozin treatment in older subjects with type 2 diabetes mellitus: a randomized trial. Hosp Pract (1995). 2013; 41:72–84.


35. Jonkers IJ, Mohrschladt MF, Westendorp RG, van der Laarse A, Smelt AH. Severe hypertriglyceridemia with insulin resistance is associated with systemic inflammation: reversal with bezafibrate therapy in a randomized controlled trial. Am J Med. 2002; 112:275–280.

