Abstract
Objective
The purpose of this study was to clarify the effects of continuous force application for extrusive tipping movement and occlusal interference on periapical root resorption in the rat mandibular first molar.
Methods
We constructed an appliance comprising a titanium screw implant with a cobalt-chromium post as the anchorage unit and a nickel-titanium closed coil spring (50 cN) as the active unit. Force was applied on the mandibular left first molar of rats for 8 (n = 10) and 15 days (n = 10; experimental groups), with the tooth in occlusion. Five rats were included as a non-treated control group to examine the body effect of the appliance. Active root resorption lacunae, identified using tartrate-resistant acid phosphatase, were evaluated in terms of the length, depth, and area.
Results
The rat mandibular first molars were mesially tipped and extruded in the occlusal direction. This mesio-occlusal tipping movement and occlusion resulted in the formation of a compression zone and active root resorption lacunae in the distoapical third of the distal roots. However, there was no significant difference in the amount of root resorption between the two experimental groups. The control group did not exhibit any active root resorption lacunae.
The etiology of undesirable root resorption is considered to be multifactorial. The factors responsible for root resorption are now investigated at the molecular level.12 Therefore, a root resorption-inducing model should be established to facilitate studies at morphological, cellular, and molecular levels. In the past, a number of etiological studies concerning root resorption have been conducted, and various root resorption models have been developed to test several hypotheses. The replantation model,3 internal resorption model,4 luxation model,5 surgical periodontal injury model,6 orthodontic injury model,78910 and freezing injury model11 have all provided novel information about the initial phases of the resorption process. An in-vitro model has also been developed.12 However, orthodontic root resorption differs from other types of root resorption because it is primarily caused by mechanical stress to the periodontium, not by bacterial infection or systemic/metabolic diseases.12 To date, the maxillary first molar has been moved and investigated in most orthodontic root resorption models in rats.12 However, the maxillary first molar in rats has five roots, including a thick mesial root and a narrow mesiobuccal root, that are quite different from their human counterparts in shape and composition. On the other hand, the mandibular first molar in rats has two thick roots that are quite similar to those of the human mandibular first molar. In order to clarify the characteristics of apical orthodontic root resorption that are different from those of other types of root resorption, we tried to establish an apical orthodontic root resorption model in the rat mandibular first molar for use in experiments examining factors such as the effects of differences in periodontal conditions and the effects of mechanical stimuli on periodontal and cellular responses. The purpose of this study was to clarify the effects of continuous force application for extrusive tipping movement and occlusal interference on periapical root resorption in the rat mandibular first molar.
All animal experiments were approved by the Institutional Animal Care and Use Committee of Tokyo Medical and Dental University (approval No. 0150192A, 0160246A). Twenty-five 8-week-old male Sprague Dawley rats were randomly divided into a non-treated control group (n = 5) and an experimental group (n = 20) to examine the body effect of an appliance. The rats in the experimental group were further randomized into an 8-day movement group (8d group, n = 10) and a 15-day movement group (15d group, n = 10). The animals were kept in separate cages in a 12-hour light/dark environment at a constant temperature of 23℃ and were provided food and water ad libitum. All procedures were conducted under general anesthesia with ketamine hydrochloride (KETALAR 50; Sankyo Co., Ltd., Tokyo, Japan) and 20% xylazine hydrochloride (Celactal 2% injections; BAYER-Japan Co., Ltd., Tokyo, Japan). During the study period, the rats were weighed every week.
When the rats in the experimental groups were 8 weeks old, the mandibular left first molars were mesioocclusally moved for 8 or 15 days. The necessary anchorage was provided by a 3.5-mm-long titanium screw implant with a 1.0-mm diameter (Shioda Co, Ltd., Tochigi, Japan)13 that was fixed to the left body of the mandible and a cobalt-chromium (Co-Cr) alloy wire with a 1.2-mm diameter extending along the incisal axis as a post (Figure 1A and 1B). A 2-mm-long, 50-cN, superelastic nickel-titanium (Ni-Ti) alloy closed coil spring (Tomy International Co., Ltd., Tokyo, Japan) extended from the tip of the Co-Cr alloy post to a clamp at the furcation of the mandibular left first molar (Figure 1C)14 to facilitate mesio-occlusal tipping movement. The antagonistic teeth were retained to produce occlusal interference.
After the completion of tooth movement in the experimental groups, the animals were anesthetized using diethyl ether and sacrificed by cervical dislocation. The left half of the mandible was dissected and immersed overnight in 10% neutral buffered formalin (pH 7.4) at 4℃. Before decalcification, the horizontal distance between the first and second molars was recorded from the center of the distal contact area of the first molar to the center of the mesial contact area of the second molar on the horizontal stage of a noncontact digital microscopic gauge (MS-214; FUSOH Co., Ltd, Tokyo, Japan; Figure 1D).15 The specimens were then decalcified in 10% (W/V) ethylene diamine tetra-acetic acid for 4 weeks at 4℃, dehydrated, and embedded in paraffin. Serial sections with a 5.0-µm thickness were cut along the sagittal axis. The sections that included the root canal were stained with hematoxylin and eosin to examine root resorption in the compression zone. Active resorption lacunae were identified by the presence of odontoclasts on the tooth surface. These multinucleated odontoclasts were detected by staining with tartrate-resistant acid phosphatase (TRAP). According to our previous study,16 specimens were incubated at 37℃ for 10 minutes in 0.1 M acetate-buffered medium (pH 5.4) containing naphthol AS-MX (Sigma, St. Louis, MO, USA) as a substrate, fast red violet (Sigma) for a color reaction, and 10 mM sodium tartrate (Sigma). The reaction was stopped by the addition of distilled water and the specimens were counterstained with hematoxylin. The control specimens were incubated in medium without a substrate under the same conditions.
The distoapical region of the distal root was observed as the compression zone. All sections that included the root canal were examined. Sagittal sections from the buccal, central, and lingual portions of the middle third of the distal root were selected for measurement (Figure 1D).
Because the distal root has a stable conformation, the root outline can be easily estimated using a reference template. The distal root was microphotographed using a digital camera (DXm1200; Nikon, Tokyo, Japan), and the length (RL), depth (RD), and area (RA) of the resorption lacunae were three-dimensionally estimated using an image analysis program (Image-Pro Plus 4.0; Media Cybernetics, Silver Spring, MD, USA). RL and RA represented the lacunar surface area and volume, respectively,17 while RD was the deepest point from the simulated root surface to the resorption surface of the lacuna16 (Figure 1E).
The amount of tooth movement and the dimensions of the root resorption lacunae are expressed as means ± standard deviations (n = 10). Comparisons among the control, 8d, and 15d groups were performed using t-tests and Wilcoxon signed-rank tests with a statistical software program (SPSS ver. 15.0; SPSS Inc., Chicago, IL, USA). The level of significance was set at 0.05.
The animals in the control and experimental groups exhibited no significant differences with regard to changes in the body weight during the study period (Figure 2).
Horizontal tooth movement was observed in both the 8d (0.15 ± 0.09 mm, n = 10) and 15d (0.26 ± 0.13 mm, n = 10) groups, but not in the control group. The amount of tooth movement was not significantly different between the two experimental groups (Figure 3).
TRAP-positive multinucleated cells, i.e., odontoclasts, were identified in the root resorption lacunae, indicating newly formed lacunae (Figure 4). The control group did not exhibit any active lacunae. In all specimens from the 8d and 15d groups, resorption facing the alveolar bone close to the root apex had occurred in the distoapical third of the mesial and distal roots; this resorption was more severe in the mesial roots. Moreover, most root resorption areas did not exhibit a hyalinization zone or necrotic periodontal area.
The amount and severity of root resorption exhibited no significant differences between the 8d and 15d groups (Figure 5).
In our root resorption model, we applied known root resorption factors during tooth movement, such as continuous force application,8151819 long duration of force application or tooth movement,720 mesio-occlusal tipping for the induction of high stress at the root apex,2122 and movement under occlusal interference from antagonist teeth.2324 Therefore, this experimental model could result in root resorption in the anticipated compression zone.
Because of mesio-occlusal tipping movement, the distoapical third of the mesial and distal roots was defined as the compression zone. Mesial tipping alone may not create adequate stress or stress distribution to induce root resorption in the distoapical third of the root.22
Because tipping movement and occlusal interference were simultaneously applied, resorption was more evident in the mesial root than in the distal root. We speculate that an intruding vector of force for a jiggling effect may have occurred along the mesial root and was focused in the apical region, whereas the extruding vector occurred along the distal root.2225
Intermittent orthodontic forces have often been applied to the rat molar resulting in tooth movement without root resorption; this may be due to a decrease in force toward the end of the experiment.26 In the present study, we utilized a continuous force for the maintenance of mechanical forces acting on the periodontium. This continuous force application stimulates inflammation through the promotion of inflammatory mediators27 secreted from local cells and migrated leukocytes to induce bone and tooth destruction by maintaining the resorption function of osteoclasts and odontoclasts and protecting them from apoptosis.28
Moreover, most of the root resorption areas did not exhibit a hyalinization zone or necrotic periodontal area, probably because of a relatively long duration of tooth movement.29
The increase in the severity of root resorption with time may have resulted from the continuous force applied by the superelastic Ni-Ti closed coil spring, which does not provide a resting period to allow tissue repair.30
Unlike that in previously developed root resorption models such as the replantation model3 and the periodontal ligament injury model induced by surgical procedures,6 root resorption in our model was not caused by damage due to necrosis/infection. In addition, it occurred in the main roots of the rat mandibular first molar, which is convenient for several experimental procedures such as extraction and sectioning. Moreover, the rat mandibular first molar is a suitable representative of the human mandibular first molar because it exhibits a similar root configuration.
In conclusion, periapical root resorption was induced by continuous extrusive tipping force and occlusal interference in rat mandibular molars. These data suggest that we orthodontists had better take care not to induce severe and long lasting occlusal interference during our orthodontic treatment.
Figures and Tables
Figure 1
A and B, The orthodontic appliance used for extrusive tipping movement. The mandibular left first molar is mesio-occlusally tipped by an orthodontic appliance comprising a 3.5-mm-long titanium screw implant (I) with a 1.0-mm diameter; a cobalt-chromium wire (P) with a 1.2-mm diameter; and a 2-mm-long, 50-cN nickel-titanium (Ni-Ti) closed coil spring (CS). The attachment of the appliance to the body of the mandible and the mandibular first molar is also illustrated (A). B shows the appliance and screw implant fixed in the oral cavity of a rat. C, The load-displacement curve. A part of the load-displacement curve for the 50-cN Ni-Ti closed coil spring shows the unloading (reverse martensitic transformation) part of the force, when the spring returns to the original length. The spring was activated to 130–180% (arrowheads), which resulted in approximately 50 cN of continuous force. D, The areas assessed for root resorption. The horizontal distance between the first and second molars (the space between arrows) was recorded using a noncontact digital microscopic gauge. The distoapical compression zone (small rectangular area) in the distal root of the rat mandibular first molar (dotted line) is evaluated by averaging the area in the buccal, central, and lingual portions of the middle third of the root canal. The cross-section of the distal root is shown in the figure in the right panel. E, The measurement of root resorption lacunae. a, b, and c show how the length (RL, black line), depth (RD, dotted line), and area (RA, black region) of the active root resorption lacunae were measured on a histological section. Hematoxylin and eosin stain; bar, 250 µm.
L, Lower incisor; M, mesial; D, distal; T, tooth; B, alveolar bone; PDL, periodontal ligament.
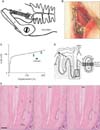
Figure 2
Changes in the body weight of rats in the control (solid line) and experimental (dotted line; extrusive tipping movement via continuous force application on the mandibular left first molar for 8 or 15 days) groups during the study period. Values are expressed as means ± standard deviations for 5 to 10 rats. All animals showed normal growth during the experiment, with no significant differences. This indicates few systemic problems caused by the implants and appliances.
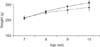
Figure 3
Horizontal tooth movement in the two experimental groups of rats (continuous extrusive tipping force application on the mandibular left first molar for 8 or 15 days). Horizontal tooth movement was observed in both the 8-day movement group (8d; 0.15 ± 0.09 mm, n = 10) and 15-day movement group (15d; 0.26 ± 0.13 mm, n = 10) groups, but not in the control (C) group. The amount of tooth movement was not significantly different between the two experimental groups. The bar indicates the standard deviation.
**p < 0.01 compared with the control group.
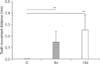
Figure 4
Tartrate-resistant acid phosphatase (TRAP) and hematoxylin and eosin (H–E)-stained sections from the distoapical third of the distal roots of rat mandibular left first molars. Active root resorption lacunae in the control (A, D), 8-day (B, E; continuous extrusive tipping force application for 8 days), and 15-day (C, F; continuous extrusive tipping force application for 15 days) specimens are stained with TRAP (A, B, C) and H–E (D, E, F). The solid line indicates the active root resorption sites. TRAP-positive multinucleated cells, i.e., odontoclasts (red-stained cells, arrowheads), are observed in the active lacunae (B, C). Bar, 250 µm.
B, Alveolar bone; T, tooth; PDL, periodontal ligament; M, mesial; D, distal.
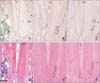
Figure 5
Amount of root resorption measured in terms of the length (RL), depth (RD), and area (RA) of resorption lacunae in 8- and 15-day specimens obtained from rat mandibular left first molars subjected to continuous extrusive tipping force for 8 and 15 days, respectively The amount and severity of root resorption exhibited no significant differences between the 8-day group (8d) and 15-day group (15d).
**p < 0.01 compared with the control (C) group. The bar indicates the standard deviation.
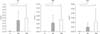
ACKNOWLEDGEMENTS
The authors express their gratitude to Professor Emeritus Kunimichi Soma and the staff members of our department for their kind advice. This study was financially supported by JSPS KAKENHI Grant Numbers 10771169, 12771271, 19671989, 23593022, 15K11344.
Notes
References
1. Roscoe MG, Meira JB, Cattaneo PM. Association of orthodontic force system and root resorption: a systematic review. Am J Orthod Dentofacial Orthop. 2015; 147:610–626.


2. Weltman B, Vig KW, Fields HW, Shanker S, Kaizar EE. Root resorption associated with orthodontic tooth movement: a systematic review. Am J Orthod Dentofacial Orthop. 2010; 137:462–476.


3. Andreasen FM, Andreasen JO. Resorption and mineralization processes following root fracture of permanent incisors. Endod Dent Traumatol. 1988; 4:202–214.


4. Wedenberg C, Lindskog S. Experimental internal resorption in monkey teeth. Endod Dent Traumatol. 1985; 1:221–227.


5. Birkedal-Hansen H. External root resorption caused by luxation of rat molars. Scand J Dent Res. 1973; 81:47–61.


6. Andreasen JO. Histometric study of healing of periodontal tissues in rats after surgical injury. II. Healing events of alveolar bone, periodontal ligaments and cementum. Odontol Revy. 1976; 27:131–144.
7. Casa MA, Faltin RM, Faltin K, Sander FG, Arana-Chavez VE. Root resorptions in upper first premolars after application of continuous torque moment. Intra-individual study. J Orofac Orthop. 2001; 62:285–295.


8. Faltin RM, Faltin K, Sander FG, Arana-Chavez VE. Ultrastructure of cementum and periodontal ligament after continuous intrusion in humans: a transmission electron microscopy study. Eur J Orthod. 2001; 23:35–49.


9. Choy K, Pae EK, Park Y, Kim KH, Burstone CJ. Effect of root and bone morphology on the stress distribution in the periodontal ligament. Am J Orthod Dentofacial Orthop. 2000; 117:98–105.


10. Killiany DM. Root resorption caused by orthodontic treatment: an evidence-based review of literature. Semin Orthod. 1999; 5:128–133.


11. Wesselink PR, Beertsen W, Everts V. Resorption of the mouse incisor after the application of cold to the periodontal attachment apparatus. Calcif Tissue Int. 1986; 39:11–21.


12. Boyde A, Ali NN, Jones SJ. Resorption of dentine by isolated osteoclasts in vitro. Br Dent J. 1984; 156:216–220.


13. Kameyama T, Matsumoto Y, Warita H, Otsubo K, Soma K. A mechanical stress model applied to the rat periodontium: using controlled magnitude and direction of orthodontic force with an absolute anchorage. Oral Med Pathol. 2002; 7:1–7.


14. Tobiume Y, Otsubo K, Soma K, Yoneyama T, Hamanaka H. Improvement in the load changeability of super-elastic Ti-Ni alloy orthodontic closed coil spring by the two-step heat treatment. J Jpn Soc Dent Mater Dev. 2000; 19:170–178.
15. Kohno T, Matsumoto Y, Kanno Z, Warita H, Soma K. Experimental tooth movement under light orthodontic forces: rates of tooth movement and changes of the periodontium. J Orthod. 2002; 29:129–135.


16. Domon S, Shimokawa H, Matsumoto Y, Yamaguchi S, Soma K. In situ hybridization for matrix metalloproteinase-1 and cathepsin K in rat root-resorbing tissue induced by tooth movement. Arch Oral Biol. 1999; 44:907–915.


17. Kameyama Y, Nakane S, Maeda H, Fujita K, Takesue M, Sato E. Inhibitory effect of aspirin on root resorption induced by mechanical injury of the soft periodontal tissues in rats. J Periodontal Res. 1994; 29:113–117.


18. Owman-Moll P, Kurol J, Lundgren D. Continuous versus interrupted continuous orthodontic force related to early tooth movement and root resorption. Angle Orthod. 1995; 65:395–401.
19. Chan E, Darendeliler MA. Physical properties of root cementum: Part 5. Volumetric analysis of root resorption craters after application of light and heavy orthodontic forces. Am J Orthod Dentofacial Orthop. 2005; 127:186–195.


20. Kurol J, Owman-Moll P, Lundgren D. Time-related root resorption after application of a controlled continuous orthodontic force. Am J Orthod Dentofacial Orthop. 1996; 110:303–310.


21. Horiuchi A, Hotokezaka H, Kobayashi K. Correlation between cortical plate proximity and apical root resorption. Am J Orthod Dentofacial Orthop. 1998; 114:311–318.


22. Rudolph DJ, Willes PMG, Sameshima GT. A finite element model of apical force distribution from orthodontic tooth movement. Angle Orthod. 2001; 71:127–131.
23. Follin ME, Ericsson I, Thilander B. Occurrence and distribution of root resorption in orthodontically moved premolars in dogs. Angle Orthod. 1986; 56:164–175.
24. Brin I, Ben-Bassat Y, Heling I, Engelberg A. The influence of orthodontic treatment on previously traumatized permanent incisors. Eur J Orthod. 1991; 13:372–377.


25. Parker RJ, Harris EF. Directions of orthodontic tooth movements associated with external apical root resorption of the maxillary central incisor. Am J Orthod Dentofacial Orthop. 1998; 114:677–683.


26. Konoo T, Kim YJ, Gu GM, King GJ. Intermittent force in orthodontic tooth movement. J Dent Res. 2001; 80:457–460.


27. Cooper SM, Sims MR. Evidence of acute inflammation in the periodontal ligament subsequent to orthodontic tooth movement in rats. Aust Orthod J. 1989; 11:107–109.
28. Hughes B, King GJ. Effect of orthodontic appliance reactivation during the period of peak expansion in the osteoclast population. Anat Rec. 1998; 251:80–86.


29. Brudvik P, Rygh P. Root resorption beneath the main hyalinized zone. Eur J Orthod. 1994; 16:249–263.


30. Owman-Moll P, Kurol J, Lundgren D. Repair of orthodontically induced root resorption in adolescents. Angle Orthod. 1995; 65:403–408.