Abstract
Lung cancer is one of the most commonly diagnosed cancers and the leading cause of cancer-related deaths worldwide. Although progress in the treatment of advanced non-small cell lung cancer (NSCLC) has been made over the past decade, the 5-year survival rate in patients with lung cancer remains only 10%–20%. Obviously, new therapeutic options are required for patients with advanced NSCLC and unmet medical needs. Cancer immunotherapy is an evolving treatment modality that uses a patient's own immune systems to fight cancer. Theoretically, cancer immunotherapy can result in long-term cancer remission and may not cause the same side effects as chemotherapy and radiation. Immuno-oncology has become an important focus of basic research as well as clinical trials for the treatment of NSCLC. Immune checkpoint inhibitors are the most promising approach for cancer immunotherapy and they have become the standard of care for patients with advanced NSCLC. This review summarizes basic tumor immunology and the relevant clinical data on immunotherapeutic approaches, especially immune checkpoint inhibitors in NSCLC.
Lung cancer is one of the most commonly diagnosed cancers and the leading cause of cancer-related deaths worldwide1. In Korea, lung cancer was the fourth most common cancer in 2013, with 23,177 newly diagnosed patients, and the most common cause of cancer-related deaths, accounting for 22.8% of all cancer-related deaths in 201423. Non-small cell lung cancer (NSCLC) is diagnosed in approximately 85% of patients with lung cancer, but most of these patients are diagnosed at an inoperable or advanced stage4.
Progress in the treatment of advanced NSCLC over the past decade includes the introduction of the cytotoxic chemotherapy agent pemetrexed for tumors of non-squamous histology and the development of molecularly targeted agents, including epidermal growth factor receptor–tyrosine kinase inhibitors (EGFR-TKI) and anaplastic lymphoma kinase (ALK) inhibitors, for tumors with activating mutations, most of adenocarcinoma histology45. These agents have resulted in better survival outcomes45. The frequency of EGFR mutations in tumors of adenocarcinoma is highest in patients from Asia–Pacific countries, at 47% (range, 20%–76%)6. Moreover, the incidence of adenocarcinoma is steadily increasing, making it the most common subtype of lung cancer in Korea7.
These advanced treatments, however, are unavailable to patients with squamous cell histology and those without appropriate molecular alterations, making their prognosis as poor as ever. Moreover, acquired resistance to targeted agents is now a challenging problem in patients who progress on these therapies45. Therefore, the 5-year survival rate in patients with lung cancer ranges remains only 10%–20% in both developed and developing countries, despite improving up to 10% in most countries8. Obviously, new therapeutic options are required for patients with advanced NSCLC and unmet medical needs.
Immunotherapy, which uses a patient's own immune system, has recently appeared as another modality for cancer treatment. Immuno-oncology has become an important focus of basic research and clinical trials for the treatment of NSCLC9101112131415. This review summarizes basic tumor immunology and clinical data on immunotherapeutic approaches, especially immune checkpoint inhibitors in NSCLC.
Historically, immunity has signified a defense mechanism against infectious diseases. However, noninfectious foreign substances can also elicit immune responses. Therefore, the immune system reacts not only to infectious microbes but to cancer cells, and has the potential to kill cancer cells16.
The immune system consists of the innate immune system, which reacts initially to foreign substances, and the adaptive immune system, which responds subsequently. The innate immune system includes complement proteins and cellular components, including natural killer cells (NKs), dendritic cells (DCs), polymorphonuclear leukocytes, mast cells, and macrophages. The adaptive immune system includes humoral immunity mediated by antibodies produced by B lymphocytes, and cellular immunity mediated by T lymphocytes161718. Natural killer T (NKT) cells and γδ T cells are involved in both innate and adaptive immunity17. The innate immune system is ready to respond rapidly, in the absence of prior exposure, and is antigen-nonspecific. By contrast, the adaptive immune response is slower to develop, educated to recall prior exposure, known as memory, and is antigen-specific161718.
Cancer immunotherapy is an evolving treatment modality that uses a patient's own immune system to fight cancer. Theoretically, cancer immunotherapy can result in long-term cancer remission and may not cause the same side effects as chemotherapy and radiation1920.
Classically, cancer immunosurveillance hypothesizes that the immune system can recognize and eliminate nascent transformed cells21. However, tumors that developed in immunocompetent mice were found to be less immunogenic than tumors that developed in immunodeficient mice22. These findings indicated that, paradoxically, the immune system assists in the eventual outgrowth of cancers that are better able to escape immune detection. Thus, tumors are imprinted by the immunologic environment in which they form2223.
Because the immune system can promote as well as suppress cancer growth, the broader term cancer immunoediting was proposed to describe better these activities, in place of the term cancer immunosurveillance222324.
Cancer immunoediting consists of three phases of relations between cancer cells and the immune system: elimination, equilibrium, and escape (Figure 1). In the elimination phase, the immune system detects and destroys transformed cancer cells from normal tissue before they become clinically detectable tumors. This phase corresponds to the concept of cancer immunosurveillance, in which the innate and adaptive immune systems work together. A proposed model for the elimination phase consists of four steps. In the first step, innate immune cells, such as NKs, NKT cells, and γδ T cells, recognize transformed cells and produce interferon γ (IFN-γ). In the second step, IFN-γ induces the death of a limited number of tumor cells and some chemokines recruit NKs, DCs, and macrophages. DCs ingest dead tumor cells and migrate to the draining lymph node. In the third step, NK cells and macrophages produce interleukin (IL)-12 and IFN-γ, which kill additional tumor cells while tumor antigen-specific T cells develop in the draining lymph nodes. In the fourth step, tumor antigen-specific T cells home to the tumor site and destroy tumor cells2324.
During the equilibrium phase, any cancer cell variant that has survived the elimination phase has increased resistance to immune recognition. The immune system also holds cancer cells in a functionally dormant state using adaptive immune cells such as T cells. Thus, the immune system prevents cancer cell outgrowth and sculpts the immunogenicity of these cancer cells2324.
During the escape phase, the immune system can no longer block tumor cells outgrowth, resulting in the emergence of cancer cells and the development of clinically observable malignant disease. Cancer cell escape can occur through many different mechanisms, including the loss of tumor antigens, resulting in the evasion of immune recognition, increased resistance to the cytotoxic effects of the immune system, and establishment of an immunosuppressive tumor microenvironment2324.
An anticancer immune response can be divided into several steps, known as the cancer-immunity cycle (Figure 2)25. First, tumors release tumor cell antigens, which are captured by antigen presenting cells (APCs) such as DCs. The APCs subsequently process and present these captured antigens on major histocompatibility complex (MHC) molecules, and migrate to draining lymph nodes. In the lymph nodes, T cell receptors on the surface of T cells recognize the antigenic peptides presented by the MHC. However, T-cell activation also requires the interaction of co-stimulatory signals, such as between proteins of the B7 family (CD80 or CD86) on APCs and CD28 on T cells. Finally, activated T cells migrate to and infiltrate the tumor bed, binding to tumor cells and killing them2526.
Each step of the cancer-immunity cycle may be a potential target for immunotherapy. Vaccines can promote tumor antigen presentation by APCs, cytotoxic T-lymphocyte-associated antigen 4 (CTLA-4) blockade can promote the priming phase, and programmed cell death protein 1 (PD-1) blockade or programmed death ligand 1 (PD-L1) blockade can promote the effector phase, consisting of the killing of cancer cells by activated T cells2526.
After T cells are activated, a checkpoint system is triggered to inhibit further T-cell activation. Originally this mechanism helps to regulate immune responses and maintain immune balance, thereby preventing autoimmune reactions. Tumors can also protect themselves from the immune system using checkpoints as immune resistance mechanisms91527.
Cancer cells can induce and recruit immunosuppressive cells in the tumor microenvironment. These immunosuppressive cells include regulatory T cells (Tregs), myeloid-derived suppressor cells (MDSCs), and M2 tumor-associated macrophages (TAMs)91528. Immunosuppressive molecules such as IL-10, transforming growth factor β, and indoleamine 2,3-dioxygenase (IDO) are also secreted by cancer cells or immunosuppressive cells1528.
Although lung cancer has been regarded classically as a non-immunogenic malignancy, recent understanding of the immune system suggests that antitumor immune responses to lung cancer can be induced, that their magnitude may correlate with clinical outcomes, and that immunotherapy may be a therapeutic option in lung cancer91115.
Several retrospective, immunohistochemical (IHC) analyses of lung cancer specimens have shown that cellular immune responses may be associated with clinical prognosis. For example, the survival rate of patients with squamous cell carcinoma of the lung, particularly those with early stage tumors, was found to be significantly higher in the presence than in the absence of tumor-infiltrating lymphocytes (TILs), which were almost entirely CD8+ T cells29. High levels of infiltration of both CD8+ T cells and CD4+ T cells were also associated with significantly higher survival rates in patients with NSCLC30. By contrast, a high ratio of Tregs to TILs correlated with a significantly higher risk of recurrence in patients with stage I NSCLC31.
The goal of immunotherapy is to potentiate the immune system's response to cancer cells. The adaptive immune system, especially T cells, plays an important role in anticancer immune responses15. Studies to date have focused on two immunotherapeutic strategies in NSCLC: cancer vaccines and immune checkpoint inhibitors. Cancer vaccines are antigen-specific immunotherapies that augment tumor recognition by the immune system, whereas immune checkpoint inhibitors are antigen non-specific therapies that overcome tumor immunosuppression9111215.
Cancer vaccines stimulate the immune system to recognize tumor antigens. Injection of a cancer vaccine, composed of tumor-associated antigens (TAA) and adjuvant, into the skin (usually intradermally) results in the uptake of TAAs by APCs such as DCs. These APCs process these TAAs and migrate to draining lymph nodes, where the activated APCs present antigens to T cells, resulting in their activation91532.
Several cancer vaccines have been studied in NSCLC, including melanoma-associated antigen-A3 (MAGE-A3)3334, liposomal-BLP25 (tecemotide)3536, TG401037, and CIMAvax-EGF38. To date, however, these cancer vaccines remain suboptimal because the induction of the desired immune response is weak, responses are short lived, and memory formation is defective. The tumor microenvironment is also potently immunosuppressive, resulting in the rapid inactivation of vaccine-induced effector lymphocytes. These hurdles must be overcome to develop clinically effective cancer vaccines3940. Consistently, however, these vaccines have been found more effective in patients with minimal disease burden. Patient selection based on predictive biomarkers will be a major future challenge in the development of cancer vaccines124142.
Immune checkpoint inhibitors are the most promising approach for cancer immunotherapy. Immune checkpoint inhibitors most investigated in clinical trials of patients with NSCLC include antibodies against CTLA-4, PD-1, and PD-L1. A major advantage of these immune checkpoint inhibitors is their ability to elicit antitumor immune responses regardless of the specific tumor antigens1213141527.
CTLA-4 is expressed on T cells and initiates inhibitory regulation during the priming phase of a T-cell activation. CTLA-4 competes with T-cell co-stimulatory receptor CD28, which is needed for T-cell activation, by binding to members of the B7 family (CD80 or CD86) on APCs. Binding of CTLA-4 to CD80 or CD86, rather than to CD28, provides an inhibitory signal to the T cell. By contrast, PD-1 is expressed on activated T cells and regulates the effector phase of T-cell responses in the tumor microenvironment. PD-1 binds to one of its ligands, PD-L1 or PD-L2, which is usually expressed on cancer cells, providing an inhibitory signal to the T cell. Therefore, inhibition of CTLA-4, PD-1, or PD-L1 results in the activation of T cells and enhancement of anticancer immune responses (Figure 3)1213141527. Immune checkpoint inhibitors for the treatment of NSCLC and the results of clinical trials are summarized in Tables 1 and 2.
Nivolumab is the first anti–PD-1 antibody in clinical development. Two phase III trials compared nivolumab with docetaxel in patients with stage IIIB or IV squamous and nonsquamous NSCLC who experienced disease progression during or after one prior platinum-based chemotherapy regimen4344. In the first phase III trial, CheckMate 017, 272 patients with squamous NSCLC were randomly assigned to receive nivolumab (3 mg/kg every 2 weeks) or docetaxel (75 mg/m2 every 3 weeks). Median overall survival (OS, 9.2 months vs. 6.0 months; hazard ratio [HR], 0.59; 95% confidence interval [CI], 0.44–0.79; p<0.001) and median progression-free survival (PFS, 3.5 months vs. 2.8 months; HR, 0.62; 95% CI, 0.47–0.81; p<0.001) were significantly longer in the nivolumab than in the docetaxel group. In addition, the 1-year OS rate (42% vs. 24%) and the objective response rate (ORR, 20% vs. 9%; p=0.008) were higher in patients treated with nivolumab than with docetaxel. Across the prespecified expression levels (1%, 5%, and 10%), the expression of the ligand for PD-1 (PD-L1) was neither prognostic nor predictive of any of the efficacy end points. Treatment-related adverse events (AEs) of grades 3 and 4 occurred less frequently with nivolumab than with docetaxel (7% vs. 55%)43.
In the second phase III trial, CheckMate 057, 582 patients with non-squamous NSCLC were randomly assigned to receive nivolumab or docetaxel, in a design similar to that of the CheckMate 017 trial. Patients with known EGFR mutation or ALK translocation were allowed to receive additional TKI therapy. Nivolumab was associated with significantly longer median OS (OS, 12.2 months vs. 9.4 months; HR, 0.73; 95% CI, 0.59–0.89; p=0.002) and higher ORR (19% vs. 12%, p=0.02). Although median PFS was shorter in the nivolumab than in the docetaxel group (median PFS, 2.3 months vs. 4.2 months), the difference was not statistically significant (HR, 0.92; 95% CI, 0.77–1.11; p<0.39). In contrast to the CheckMate 017 trial, involving patients with squamous cell NSCLC, the CheckMate 057 trial found that PD-L1 protein expression was associated with improved OS and PFS in patients treated with nivolumab. However, PD-L1 protein expression was evaluated retrospectively in prospectively collected, pretreatment (archival or recent) tumor-biopsy specimens. Grade 3 and 4 treatment-related AEs were again less frequent in patients treated with nivolumab than with docetaxel (10% vs. 54%)44.
The phase III CheckMate 026 trial compared nivolumab with platinum-based agents as first-line therapy in patients with stage IV or recurrent NSCLC. Among the 423 patients with a PD-L1 expression level ≥5%, nivolumab did not improve median PFS compared with chemotherapy (PFS, 4.2 months vs. 5.9 months; HR, 1.15; 95% CI, 0.91–1.45; p=0.25). Median OS (OS, 14.4 months vs. 13.2 months; HR,1.02; 95% CI, 0.80–1.30) and ORR (26% vs. 33%) were similar in the nivolumab and chemotherapy groups. Rates of grades 3 and 4 treatment-related AEs were lower with nivolumab than with chemotherapy (18% vs. 51%)45.
The phase I KEYNOTE-001 trial found that higher PD-L1 expression in at least 50% of tumor cells (proportion score [PS] ≥50%) was associated with higher ORR and longer PFS and OS than lower levels (PS <1% and 1%–49%) in patients with advanced NSCLC46. In the subsequent phase II/III KEYNOTE-010 trial, 1,034 patients with previously treated NSCLC and PD-L1 expression (PS ≥1%) were randomly assigned to receive pembrolizumab (2 mg/kg or 10 mg/kg) or docetaxel (75 mg/m2) every 3 weeks. In the total population (PS ≥1%), median OS was significantly longer with pembrolizumab 2 mg/kg (10.4 months vs. 8.5 months; HR, 0.71; 95% CI, 0.58–0.88; p=0.0008) and with pembrolizumab 10 mg/kg (12.7 months vs. 8.5 months; HR, 0.61; 95% CI, 0.49–0.75; p<0.0001) than with docetaxel. Among patients with high PDL1 expression (PS ≥50%), median OS was significantly longer with pembrolizumab 2 mg/kg (14.9 months vs. 8.2 months; HR, 0.54; 95% CI, 0.38–0.77; p=0.0002) and pembrolizumab 10 mg/kg (17.3 months vs. 8.2 months; HR, 0.50; 95% CI, 0.36–0.70; p<0.0001) than with docetaxel. In this latter group (PS ≥50%), median PFS was also significantly longer with pembrolizumab 2 mg/kg (5.0 months vs. 4.1 months; HR, 0.59; 95% CI, 0.44–0.78; p=0.0001) and pembrolizumab 10 mg/kg (5.2 months vs. 4.1 months; HR, 0.59; 95% CI, 0.45–0.78; p<0.0001) than with docetaxel. In the total population, however, median PFS did not differ significantly in the pembrolizumab 2 mg/kg and 10 mg/kg and docetaxel groups (3.9 months vs. 4.0 months vs. 4.0 months). ORR was significantly higher in the total population of patients treated with pembrolizumab 2 mg/kg (18% vs. 9%, p=0.0005) and pembrolizumab 10 mg/kg (18% vs. 9%, p=0.0002) than with docetaxel. Similarly, ORR in patients with high PD-L1 expression (PS ≥50%) was significantly higher following treatment with pembrolizumab 2 mg/kg (30% vs. 8%, p<0.0001) and pembrolizumab 10 mg/kg (29% vs. 8%, p<0.0001) than with docetaxel. Treatment-related AEs of grades 3 to 5 were less frequent in both pembrolizumab groups than in the docectaxel group (13% vs. 16% vs. 35%)47.
The phase III KEYNOTE-024 trial compared pembrolizumab (200 mg every 3 weeks) with platinum-based chemotherapy as first-line treatment in 305 patients with stage IV NSCLC and high PD-L1 expression (PS ≥50%). Median PFS (10.3 months vs. 6.0 months; HR, 0.50; 95% CI, 0.37–0.68; p<0.001)48 and median OS (not reached vs. 14.5 months; HR, 0.63; 95% CI, 0.46–0.88; p=0.003)49 were significantly longer with pembrolizumab than with chemotherapy. ORR was higher (44.8% vs. 27.8%) and treatment-related AEs of grades 3 to 5 were less frequent (26.6% vs. 53.3%) with pembrolizumab than with chemotherapy48.
In the phase II POPLAR trial, 287 patients with NSCLC who progressed on post-platinum chemotherapy were randomly assigned to receive atezolizumab 1,200 mg or docetaxel 75 mg/m2 every 3 weeks. In this study, PD-L1 expression was prospectively determined by IHC in tumor cells (TCs) and tumor-infiltrating immune cells (ICs). Median OS was significantly longer in patients treated with atezolizumab than with docetaxel (12.6 months vs. 9.7 months; HR, 0.73; 95% CI, 0.53–0.99; p=0.04). The OS benefit from atezolizumab increased with increasing PD-L1 expression on TCs and/or ICs, but OS was similar with atezolizumab and doectaxel in patients without PD-L1 expression. Median PFS was similar in patients treated with atezolizumab and docetaxel (2.7 months vs. 3.0 months; HR, 0.94; 95% CI, 0.72–1.23; p=0.645). ORR was similar in these two groups (14.6% vs. 14.7%), but the median duration of response was longer with atezolizumab than with docetaxel (14.3 months vs. 7.2 months; HR, 0.41; 95% CI, 0.18–0.96; p=0.034). Treatment-related AEs of grades 3 and 4 were less frequent in patients treated with atezolizumab than with docetaxel (11% vs. 39%)50.
In the phase III OAK trial, 850 patients with stage IIIB or IV NSCLC who had received 1–2 previous lines of cytotoxic chemotherapy, including at least one platinum-based combination regimen, were randomly assigned to receive atezolizumab 1,200 mg or docetaxel 75 mg/m2 every 3 weeks. Median OS was significantly longer with atezolizumab than with docetaxel in the intention-to-treat (ITT) (13.8 months vs. 9.6 months; HR, 0.73; 95% CI, 0.62–0.87; p=0.0003) and the TC1/2/3 or IC1/2/3 (PD-L1 expression ≥1% on TCs or ICs) (15.7 months vs. 10.3 months; HR, 0.74; 95% CI, 0.58–0.93; p=0.0102) populations. The OS benefit from atezolizumab was the highest in patients with high levels of PD-L1, TC3 or IC3 (PD-L1 expression ≥50% on TCs or ≥10% on ICs) (20.5 months vs. 8.9 months; HR, 0.41; 95% CI, 0.27–0.64; p<0.0001). However, OS was also better with atezolizumab than with docetaxel in patients with TC0 and IC0 (PD-L1 expression <1% on TCs and ICs). Median PFS was similar in patients treated with atezolizumab and docetaxel in the ITT population (2.8 months vs. 4.0 months; HR, 0.95; 95% CI, 0.82–1.10; p=0.4928) and in TC1/2/3 or IC1/2/3 populations. However, median PFS was significantly longer with atezolizumab than with docetaxel in the TC3 or IC3 population (4.2 months vs. 3.3 months; HR, 0.63; 95% CI, 0.43–0.91; p=0.0123). Although ORR was similar in the two groups in the ITT (14% vs. 13%) and TC1/2/3 or IC1/2/3 populations, ORR was higher with atezolizumab than with docetaxel in patients with TC3 or IC3 (31% vs. 11%). Rates of grades 3 and 4 treatment-related AEs were lower in patients treated with atezolizumab than with docetaxel (15% vs. 43%)51.
The phase II, single-arm trial BIRCH study included 667 patients with stage IIIB or IV NSCLC and PD-L1 expression ≥5% on TCs or ICs (TC2/3 or IC2/3) who received atezolizumab as first-line, second-line, and third-line or higher treatment. ORR in the 139 patients treated with first-line atezolizumab was 22% (31% in those with TC3 or IC3). Median OS was 23.5 months (26.9 months in those with TC3 or IC3) and the 12-month OS rate was 66.4% (61.5% in patients with TC3 or IC3). Median PFS was 5.4 months (5.6 months in those with TC3 or IC3). Grade 3 and 4 treatment-related AEs occurred in 9% of these patients53.
In the phase II, single-arm ATLANTIC study, 333 patients with stage IIIB or IV and EGFR/ALK wild-type NSCLC who had received at least two prior lines of chemotherapy were treated with durvalumab 10 mg/kg every 2 weeks. ORRs in patients with PD-L1 expression ≥25% and ≥90% were 16.4% and 30.9%, respectively. Median OS was 10.9 months in patients with PD-L1 ≥25% and was not reached in patients with PD-L1 ≥90%. One-year OS rates in patients with PD-L1 expression ≥25% and ≥90% were 47.7% and 50.8%, respectively52.
In the phase III PACIFIC trial, 709 patients with stage III NSCLC who had not progressed after platinum-based chemoradiotherapy were randomly assigned 2:1 to receive durvalumab 10 mg/kg or placebo every 2 weeks for up to 12 months. Median PFS was significantly longer with durvalumab than with placebo (16.8 months vs. 5.6 months; HR, 0.52; 95% CI, 0.42–0.65; p<0.001) and was independent of PD-L1 expression. ORR was significantly higher with durvalumab than with placebo (28.4% vs. 16.0%, p<0.001), whereas rates of grades 3 and 4 treatment-related AEs were similar in the two groups (29.9% vs. 26.1%)54.
Ipilimumab has been combined with cytotoxic chemotherapy or another immunotherapy agent in patients with NSCLC. In a phase II trial, 204 patients with stage IIIB or IV NSCLC were randomly assigned 1:1:1 to receive first-line paclitaxel and carboplatin with two different schedules (concurrent or phased arm) of ipilimumab or placebo. The concurrent arm consisted of four doses of ipilimumab plus chemotherapy followed by two doses of placebo plus chemotherapy. The phased arm consisted of two doses of placebo plus chemotherapy followed by four doses of ipilimumab plus chemotherapy. The median immune-related PFS (irPFS) was longer with phased treatment than with placebo (5.7 months vs. 4.6 months; HR, 0.72; 95% CI, 0.50–1.06; p=0.05), whereas concurrent treatment did not improve irPFS55.
By contrast, a subsequent phase III trial did not show that the combination of ipilimumab plus first-line chemotherapy enhanced median OS compared with chemotherapy alone in patients with squamous NSCLC (13.4 months vs. 12.4 months; HR, 0.91; 95% CI, 0.77–1.07; p=0.25)56.
The combination of immune checkpoint inhibitors and conventional therapy with different mechanisms of action may have a synergistic effect and result in clinical benefit. Conventional therapies, especially targeted therapy, can lead to a rapid initial response, but most responders will later acquire resistance and develop progressive disease. Conversely, immune checkpoint inhibitors can lead to a durable response in a relatively small percentage of responders57. Conventional therapies can also modulate the immune system, thereby affecting immunotherapy5758.
The combination of two immune checkpoint inhibitors with distinct targets, particularly anti–PD-1/anti–PD-L1 and anti–CTLA-4, may also improve response rate and survival benefit compared with monotherapy58. Many ongoing clinical trials are testing combination immunotherapies, and we look forward to successful results from phase III studies.
As part of the phase II KEYNOTE-021 trial, 123 patients with non-squamous, stage IIIB or IV, chemotherapy-naive NSCLC were randomly assigned to treatment with pembrolizumab plus carboplatin and pemetrexed or to carboplatin and pemetrexed alone. The ORR was significantly higher in patients who received pembrolizumab plus chemotherapy than chemotherapy alone (55% vs. 29%, p=0.0016). In the combination group, the ORR was 80% in patients with PS ≥50%, although there was no difference between those with PS <1% and PS ≥1% (57% vs. 54%). Median PFS was significantly longer in the combination than in the chemotherapy alone (13.0 months vs. 8.9 months; HR, 0.53; 95% CI, 0.31–0.91; p=0.010)59. The ORR was higher and PFS was longer in patients treated with pembrolizumab plus chemotherapy in this trial than in those treated with pembrolizumab alone in the KEYNOTE-024 trial. Median OS was also significantly longer in the combination group than in the chemotherapy alone group (not reached vs. 20.9 months; HR, 0.59; 95% CI, 0.34–1.05; p=0.0344)60. Treatment-related AEs of grades 3 to 5 were reported in 39% of patients in the combination group compared with 26% in the chemotherapy alone group59.
In the phase I, multicohort CheckMate 012 trial, patients with stage IIIB or IV chemotherapy-naive NSCLC were assigned to receive nivolumab alone or combination therapies. In one cohort, 56 patients were assigned to receive nivolumab 10 mg/kg plus three platinum-based doublet chemotherapy agents every 3 weeks or nivolumab 5 mg/kg plus paclitaxelcarboplatin. ORRs ranged from 33% to 47% irrespective of PD-L1 expression. The 2-year OS rate in patients treated with nivolumab 5 mg/kg plus paclitaxel-carboplatin was 62%. Treatment-related grades 3 and 4 AEs were reported in 45% of patients, and the discontinuation rate was 21%61.
In another cohort of the CheckMate 012 trial, 78 patients were randomly assigned to receive nivolumab 3 mg/kg every 2 weeks plus ipilimumab 1 mg/kg every 6 or 12 weeks. The ORRs in these groups were 38% and 47%, respectively. After pooling these two groups, ORR was found to correlate with PD-L1 expression, being 92% and 57% in patients with PD-L1 expression ≥50% and ≥1%, respectively. ORR was higher in patients treated with nivolumab plus ipilimuab in this trial than with nivolumab monotherapy in another cohort of the CheckMate 012 trial. Median PFS was longer in patients treated with ipilimumab every 12 than every 6 weeks (8.1 months vs. 3.9 months). Treatment-related grade 3 and 4 AEs in these two groups were 37% and 33%, respectively62.
In a phase Ib trial (study 006), 102 patients with immunotherapy-naive, locally advanced, or metastatic NSCLC were enrolled into the dose-escalation phase and received durvalumab and tremelimumab, a selective human IgG2 monoclonal antibody against CTLA-4. Based on safety and clinical activity, durvalumab 20 mg/kg every 4 weeks plus tremelimumab 1 mg/kg were chosen as the expansion phase doses. This dose cohort showed a manageable safety profile, with a 17% rate of grades 3 and 4 treatment-related AEs, and antitumor activity (ORR 23%) irrespective of PD-L1 status63.
The immune checkpoint inhibitors showed durable clinical responses and enhanced long-term survival in patients who benefited from these agents. However, clinical trials of unselected patients with NSCLC showed that these agents had low ORRs, of approximately 20%, as monotherapy64. Moreover, these agents have immune-related AEs, which may be life-threatening, and their costs might result in financial burdens on patients. Therefore, biomarkers predicting the benefits of these agents are required6465.
PD-L1 expression, as determined by IHC, is the most commonly used predictive biomarker for anti–PD-1 and anti–PD-L1 agents in clinical trials, with tests currently available in clinical practice646566. PD-L1 expression on TCs has been associated with immunosuppression, inhibiting the antitumor activity of T cells, as PD-L1 binding to PD-1 on activated T cells inhibits T-cell signaling and blocks antitumor immune responses27. PD-L1 expression alone may not be representative of the entire tumor microenvironment, as other concurrent immunosuppressive mechanisms, involving Treg, MDSC, TAM, and IDO, can be present6465. Higher ORRs have been observed in patients positive than negative for PD-L1, although up to 17% of PD-L1 negative patients responded4365666768. Moreover, about 50% of patients with high PD-L1 expression did not respond4865. High PD-L1 expression has also been associated with longer PFS and OS, although the results varied among clinical trials6465666768. Taken together, these findings show that PD-L1 IHC is insufficient as a predictive biomarker and has limitations. For example, multiple antibodies have been utilized in IHC assays and the interpretation of their results has not been standardized. Other limitations include tumor heterogeneity among different sections of the same sample or at different tumor sites, and dynamic changes in PD-L1 expression over time68. Several studies have compared different antibodies and IHC systems for PD-L1 tests. The Blueprint PD-L1 IHC assay comparison project revealed high concordance among the 28-8, 22C3, and SP263 assays, whereas fewer TCs were stained with the SP142 assay69. Another study found that the 28-8 and E1L3N assays were comparable, whereas fewer cells were stained using the 22C3 assay and the SP142 assay detected significantly lower PD-L1 expression in TCs70. High similarity among the different IHC assays suggests their potential interchangeability in clinical practice71. The U.S. Food and Drug Administration (FDA) has approved PD-L1 IHC 22C3 pharmDx as a companion diagnostic assay for pembrolizumab treatment in patients with NSCLC72.
Clinical trials have shown that melanomas and NSCLCs respond better to immune checkpoint inhibitors than other tumor types68. One study showed that melanomas and NSCLCs have the highest somatic mutation burden among tumor types73, suggesting that tumor mutation burden may be a predictive biomarker for treatment with immune checkpoint inhibitors. In patients with NSCLC who were treated with pembrolizumab, a higher nonsynonymous mutation burden was associated with higher ORR, longer PFS, and more durable clinical benefits74.
Many ongoing studies are attempting to identify new predictive biomarkers, including TILs, immune gene signatures, and multiplex IHC assays67. Understanding the tumor microenvironment and characterizing TILs and concurrent immunosuppressive mechanisms are therefore important.
Better understanding of the immune system in the tumor microenvironment has resulted in the development of new immunotherapy agents such as immune checkpoint inhibitors. Clinician familiarity with tumor immunology can help in understanding the process of immunotherapy and developing optimal treatment strategies for patients.
Immune checkpoint inhibitors, especially anti–PD-1 and anti–PD-L1, have become the standard of care in patients with advanced NSCLC. Nivolumab, pembrolizumab, and atezolizumab are safer and more effective than docetaxel. Pembrolizumab should be considered a first-line treatment in NSCLC with PD-L1 expression ≥50%, but without EGFR mutation and ALK rearrangement. Durvalumab may be an effective adjuvant treatment in stage III NSCLC after chemoradiotherapy.
Despite durable long-term survival, immune checkpoint inhibitors alone have a relatively low response rate. Therefore, combining immune checkpoint inhibitor with other immunotherapy or conventional therapy agents may improve response rates and provide clinical benefits to more patients. Predictive biomarkers are also essential in selecting patients who would benefit from treatment, reducing the unnecessary cost burden to patients who would not benefit from these agents. Although IHC assays of PD-L1 expression have drawbacks, they are the only currently available tests in clinical practice. Ultimately, biomarker-driven combination therapy will become a standard strategy in future immunotherapy.
References
1. Ferlay J, Soerjomataram I, Dikshit R, Eser S, Mathers C, Rebelo M, et al. Cancer incidence and mortality worldwide: sources, methods and major patterns in GLOBOCAN 2012. Int J Cancer. 2015; 136:E359–E386. PMID: 25220842.


2. Korea Central Cancer Registry. Annual report of cancer statistics in Korea in 2013 [Internet]. Goyang: National Cancer Information Center;2016. cited 2016 Jun 20. Available from: http://www.cancer.go.kr/.
3. Korean Statistical Information Service. Statistics Korea [Internet]. Daejeon: Statistics Korea;2016. cited 2016 Jun 20. Available from: http://kosis.kr/.
4. Reck M, Heigener DF, Mok T, Soria JC, Rabe KF. Management of non-small-cell lung cancer: recent developments. Lancet. 2013; 382:709–719. PMID: 23972814.


5. National Comprehensive Cancer Network. NCCN Clinical practice guidelines in oncology: non-small cell lung cancer. Version 9. 2017 [Internet]. Fort Washington: National Comprehensive Cancer Network;2017. cited 2017 Sep 28. Available from: https://www.nccn.org/professionals/physician_gls/pdf/nscl.pdf.
6. Midha A, Dearden S, McCormack R. EGFR mutation incidence in non-small-cell lung cancer of adenocarcinoma histology: a systematic review and global map by ethnicity (mutMapII). Am J Cancer Res. 2015; 5:2892–2911. PMID: 26609494.
7. Park JY, Jang SH. Epidemiology of lung cancer in Korea: recent trends. Tuberc Respir Dis (Seoul). 2016; 79:58–69. PMID: 27064578.


8. Allemani C, Weir HK, Carreira H, Harewood R, Spika D, Wang XS, et al. Global surveillance of cancer survival 1995-2009: analysis of individual data for 25,676,887 patients from 279 population-based registries in 67 countries (CONCORD-2). Lancet. 2015; 385:977–1010. PMID: 25467588.


9. Shepherd FA, Douillard JY, Blumenschein GR Jr. Immunotherapy for non-small cell lung cancer: novel approaches to improve patient outcome. J Thorac Oncol. 2011; 6:1763–1773. PMID: 21876456.


10. Thomas A, Hassan R. Immunotherapies for non-small-cell lung cancer and mesothelioma. Lancet Oncol. 2012; 13:e301–e310. PMID: 22748269.


11. Reck M. What future opportunities may immuno-oncology provide for improving the treatment of patients with lung cancer? Ann Oncol. 2012; 23(Suppl 8):viii28–viii34. PMID: 22918925.


12. Brahmer JR. Harnessing the immune system for the treatment of non-small-cell lung cancer. J Clin Oncol. 2013; 31:1021–1028. PMID: 23401435.


13. Massarelli E, Papadimitrakopoulou V, Welsh J, Tang C, Tsao AS. Immunotherapy in lung cancer. Transl Lung Cancer Res. 2014; 3:53–63. PMID: 25806281.
14. Anagnostou VK, Brahmer JR. Cancer immunotherapy: a future paradigm shift in the treatment of non-small cell lung cancer. Clin Cancer Res. 2015; 21:976–984. PMID: 25733707.


15. Carbone DP, Gandara DR, Antonia SJ, Zielinski C, Paz-Ares L. Non-small-cell lung cancer: role of the immune system and potential for immunotherapy. J Thorac Oncol. 2015; 10:974–984. PMID: 26134219.


16. Abbas AK, Lichtman AH, Pillai S. Cellular and molecular immunology. 9th ed. Philadelphia: Saunders/Elsevier;2018.
17. Liu Y, Zeng G. Cancer and innate immune system interactions: translational potentials for cancer immunotherapy. J Immunother. 2012; 35:299–308. PMID: 22495387.
18. Vesely MD, Kershaw MH, Schreiber RD, Smyth MJ. Natural innate and adaptive immunity to cancer. Annu Rev Immunol. 2011; 29:235–271. PMID: 21219185.


19. Finn OJ. Immuno-oncology: understanding the function and dysfunction of the immune system in cancer. Ann Oncol. 2012; 23(Suppl 8):viii6–viii9. PMID: 22918931.


20. Eggermont AM. Can immuno-oncology offer a truly pan-tumour approach to therapy? Ann Oncol. 2012; 23(Suppl 8):viii53–viii57. PMID: 22918930.


21. Burnet M. Cancer: a biological approach. I. The processes of control. Br Med J. 1957; 1:779–786. PMID: 13404306.
22. Shankaran V, Ikeda H, Bruce AT, White JM, Swanson PE, Old LJ, et al. IFNgamma and lymphocytes prevent primary tumour development and shape tumour immunogenicity. Nature. 2001; 410:1107–1111. PMID: 11323675.
23. Dunn GP, Bruce AT, Ikeda H, Old LJ, Schreiber RD. Cancer immunoediting: from immunosurveillance to tumor escape. Nat Immunol. 2002; 3:991–998. PMID: 12407406.


24. Schreiber RD, Old LJ, Smyth MJ. Cancer immunoediting: integrating immunity's roles in cancer suppression and promotion. Science. 2011; 331:1565–1570. PMID: 21436444.


25. Chen DS, Mellman I. Oncology meets immunology: the cancer-immunity cycle. Immunity. 2013; 39:1–10. PMID: 23890059.


26. Mellman I, Coukos G, Dranoff G. Cancer immunotherapy comes of age. Nature. 2011; 480:480–489. PMID: 22193102.


27. Pardoll DM. The blockade of immune checkpoints in cancer immunotherapy. Nat Rev Cancer. 2012; 12:252–264. PMID: 22437870.


28. Butt AQ, Mills KH. Immunosuppressive networks and checkpoints controlling antitumor immunity and their blockade in the development of cancer immunotherapeutics and vaccines. Oncogene. 2014; 33:4623–4631. PMID: 24141774.


29. Ruffini E, Asioli S, Filosso PL, Lyberis P, Bruna MC, Macri L, et al. Clinical significance of tumor-infiltrating lymphocytes in lung neoplasms. Ann Thorac Surg. 2009; 87:365–371. PMID: 19161739.


30. Hiraoka K, Miyamoto M, Cho Y, Suzuoki M, Oshikiri T, Nakakubo Y, et al. Concurrent infiltration by CD8+ T cells and CD4+ T cells is a favourable prognostic factor in non-small-cell lung carcinoma. Br J Cancer. 2006; 94:275–280. PMID: 16421594.


31. Petersen RP, Campa MJ, Sperlazza J, Conlon D, Joshi MB, Harpole DH Jr, et al. Tumor infiltrating Foxp3+ regulatory T-cells are associated with recurrence in pathologic stage I NSCLC patients. Cancer. 2006; 107:2866–2872. PMID: 17099880.


32. Drake CG, Lipson EJ, Brahmer JR. Breathing new life into immunotherapy: review of melanoma, lung and kidney cancer. Nat Rev Clin Oncol. 2014; 11:24–37. PMID: 24247168.


33. Vansteenkiste J, Zielinski M, Linder A, Dahabreh J, Gonzalez EE, Malinowski W, et al. Adjuvant MAGE-A3 immunotherapy in resected non-small-cell lung cancer: phase II randomized study results. J Clin Oncol. 2013; 31:2396–2403. PMID: 23715567.


34. Vansteenkiste JF, Cho BC, Vanakesa T, De Pas T, Zielinski M, Kim MS, et al. Efficacy of the MAGE-A3 cancer immunotherapeutic as adjuvant therapy in patients with resected MAGE-A3-positive non-small-cell lung cancer (MAGRIT): a randomised, double-blind, placebo-controlled, phase 3 trial. Lancet Oncol. 2016; 17:822–835. PMID: 27132212.


35. Butts C, Socinski MA, Mitchell PL, Thatcher N, Havel L, Krzakowski M, et al. Tecemotide (L-BLP25) versus placebo after chemoradiotherapy for stage III non-small-cell lung cancer (START): a randomised, double-blind, phase 3 trial. Lancet Oncol. 2014; 15:59–68. PMID: 24331154.


36. Wu YL, Park K, Soo RA, Sun Y, Tyroller K, Wages D, et al. INSPIRE: a phase III study of the BLP25 liposome vaccine (L-BLP25) in Asian patients with unresectable stage III non-small cell lung cancer. BMC Cancer. 2011; 11:430. PMID: 21982342.


37. Quoix E, Lena H, Losonczy G, Forget F, Chouaid C, Papai Z, et al. TG4010 immunotherapy and first-line chemotherapy for advanced non-small-cell lung cancer (TIME): results from the phase 2b part of a randomised, double-blind, placebo-controlled, phase 2b/3 trial. Lancet Oncol. 2016; 17:212–223. PMID: 26727163.


38. Rodriguez PC, Popa X, Martinez O, Mendoza S, Santiesteban E, Crespo T, et al. A phase III clinical trial of the epidermal growth factor vaccine CIMAvax-EGF as switch maintenance therapy in advanced non-small cell lung cancer patients. Clin Cancer Res. 2016; 22:3782–3790. PMID: 26927662.


39. Sasada T, Komatsu N, Suekane S, Yamada A, Noguchi M, Itoh K. Overcoming the hurdles of randomised clinical trials of therapeutic cancer vaccines. Eur J Cancer. 2010; 46:1514–1519. PMID: 20413296.


40. Mellstedt H, Vansteenkiste J, Thatcher N. Vaccines for the treatment of non-small cell lung cancer: investigational approaches and clinical experience. Lung Cancer. 2011; 73:11–17. PMID: 21474197.


41. Cuppens K, Vansteenkiste J. Vaccination therapy for non-small-cell lung cancer. Curr Opin Oncol. 2014; 26:165–170. PMID: 24441502.


42. Makkouk A, Weiner GJ. Cancer immunotherapy and breaking immune tolerance: new approaches to an old challenge. Cancer Res. 2015; 75:5–10. PMID: 25524899.


43. Brahmer J, Reckamp KL, Baas P, Crino L, Eberhardt WE, Poddubskaya E, et al. Nivolumab versus docetaxel in advanced squamous-cell non-small-cell lung cancer. N Engl J Med. 2015; 373:123–135. PMID: 26028407.


44. Borghaei H, Paz-Ares L, Horn L, Spigel DR, Steins M, Ready NE, et al. Nivolumab versus docetaxel in advanced nonsquamous non-small-cell lung cancer. N Engl J Med. 2015; 373:1627–1639. PMID: 26412456.


45. Carbone DP, Reck M, Paz-Ares L, Creelan B, Horn L, Steins M, et al. First-Line Nivolumab in stage IV or recurrent non-small-cell lung cancer. N Engl J Med. 2017; 376:2415–2426. PMID: 28636851.


46. Garon EB, Rizvi NA, Hui R, Leighl N, Balmanoukian AS, Eder JP, et al. Pembrolizumab for the treatment of non-small-cell lung cancer. N Engl J Med. 2015; 372:2018–2028. PMID: 25891174.


47. Herbst RS, Baas P, Kim DW, Felip E, Perez-Gracia JL, Han JY, et al. Pembrolizumab versus docetaxel for previously treated, PD-L1-positive, advanced non-small-cell lung cancer (KEYNOTE-010): a randomised controlled trial. Lancet. 2016; 387:1540–1550. PMID: 26712084.


48. Reck M, Rodriguez-Abreu D, Robinson AG, Hui R, Csoszi T, Fulop A, et al. Pembrolizumab versus chemotherapy for PD-L1-positive non-small-cell lung cancer. N Engl J Med. 2016; 375:1823–1833. PMID: 27718847.


49. Brahmer JR, Rodriguez-Abreu D, Robinson AG, Hui R, Csoszi T, Fulop A, et al. Progression after the next line of therapy (PFS2) and updated OS among patients (pts) with advanced NSCLC and PD-L1 tumor proportion score (TPS) ≥50% enrolled in KEYNOTE-024. J Clin Oncol. 2017; 35(15 Suppl):9000.


50. Fehrenbacher L, Spira A, Ballinger M, Kowanetz M, Vansteenkiste J, Mazieres J, et al. Atezolizumab versus docetaxel for patients with previously treated non-small-cell lung cancer (POPLAR): a multicentre, open-label, phase 2 randomised controlled trial. Lancet. 2016; 387:1837–1846. PMID: 26970723.


51. Rittmeyer A, Barlesi F, Waterkamp D, Park K, Ciardiello F, von Pawel J, et al. Atezolizumab versus docetaxel in patients with previously treated non-small-cell lung cancer (OAK): a phase 3, open-label, multicentre randomised controlled trial. Lancet. 2017; 389:255–265. PMID: 27979383.


52. Garassino M, Vansteenkiste J, Kim JH, Lena H, Mazieres J, Powderly J, et al. Durvalumab in ≥3rd-line locally advanced or metastatic, EGFR/ALK wild-type NSCLC: results from the phase 2 ATLANTIC study. J Thorac Oncol. 2017; 12(Suppl):S10–S11.
53. Peters S, Gettinger S, Johnson ML, Janne PA, Garassino MC, Christoph D, et al. Phase II trial of atezolizumab as first-line or subsequent therapy for patients with programmed death-ligand 1-selected advanced non-small-cell lung cancer (BIRCH). J Clin Oncol. 2017; 35:2781–2789. PMID: 28609226.
54. Antonia SJ, Villegas A, Daniel D, Vicente D, Murakami S, Hui R, et al. Durvalumab after chemoradiotherapy in stage III non-small-cell lung cancer. N Engl J Med. 2017; 377:1919–1929. PMID: 28885881.


55. Lynch TJ, Bondarenko I, Luft A, Serwatowski P, Barlesi F, Chacko R, et al. Ipilimumab in combination with paclitaxel and carboplatin as first-line treatment in stage IIIB/IV non-small-cell lung cancer: results from a randomized, double-blind, multicenter phase II study. J Clin Oncol. 2012; 30:2046–2054. PMID: 22547592.


56. Govindan R, Szczesna A, Ahn MJ, Schneider CP, Gonzalez Mella PF, Barlesi F, et al. Phase III trial of ipilimumab combined with paclitaxel and carboplatin in advanced squamous non-small-cell lung cancer. J Clin Oncol. 2017; 35:3449–3457. PMID: 28854067.


57. Champiat S, Ileana E, Giaccone G, Besse B, Mountzios G, Eggermont A, et al. Incorporating immune-checkpoint inhibitors into systemic therapy of NSCLC. J Thorac Oncol. 2014; 9:144–153. PMID: 24419410.


58. Antonia SJ, Larkin J, Ascierto PA. Immuno-oncology combinations: a review of clinical experience and future prospects. Clin Cancer Res. 2014; 20:6258–6268. PMID: 25341541.


59. Langer CJ, Gadgeel SM, Borghaei H, Papadimitrakopoulou VA, Patnaik A, Powell SF, et al. Carboplatin and pemetrexed with or without pembrolizumab for advanced, non-squamous non-small-cell lung cancer: a randomised, phase 2 cohort of the open-label KEYNOTE-021 study. Lancet Oncol. 2016; 17:1497–1508. PMID: 27745820.


60. Borghaei H, Langer CJ, Gadgeel S, Papadimitrakopoulou VA, Patnaik A, Powell SF, et al. Updated results from KEYNOTE-021 cohort G: a randomized, phase 2 study of pemetrexed and carboplatin (PC) with or without pembrolizumab (pembro) as first-line therapy for advanced nonsquamous NSCLC. Ann Oncol. 2017; 28(Suppl_5):636–637.


61. Rizvi NA, Hellmann MD, Brahmer JR, Juergens RA, Borghaei H, Gettinger S, et al. Nivolumab in combination with platinum-based doublet chemotherapy for first-line treatment of advanced non-small-cell lung cancer. J Clin Oncol. 2016; 34:2969–2979. PMID: 27354481.


62. Hellmann MD, Rizvi NA, Goldman JW, Gettinger SN, Borghaei H, Brahmer JR, et al. Nivolumab plus ipilimumab as first-line treatment for advanced non-small-cell lung cancer (CheckMate 012): results of an open-label, phase 1, multicohort study. Lancet Oncol. 2017; 18:31–41. PMID: 27932067.


63. Antonia S, Goldberg SB, Balmanoukian A, Chaft JE, Sanborn RE, Gupta A, et al. Safety and antitumour activity of durvalumab plus tremelimumab in non-small cell lung cancer: a multicentre, phase 1b study. Lancet Oncol. 2016; 17:299–308. PMID: 26858122.


64. Remon J, Chaput N, Planchard D. Predictive biomarkers for programmed death-1/programmed death ligand immune checkpoint inhibitors in nonsmall cell lung cancer. Curr Opin Oncol. 2016; 28:122–129. PMID: 26756384.


65. Gridelli C, Ardizzoni A, Barberis M, Cappuzzo F, Casaluce F, Danesi R, et al. Predictive biomarkers of immunotherapy for non-small cell lung cancer: results from an Experts Panel Meeting of the Italian Association of Thoracic Oncology. Transl Lung Cancer Res. 2017; 6:373–386. PMID: 28713682.


66. Chae YK, Pan A, Davis AA, Raparia K, Mohindra NA, Matsangou M, et al. Biomarkers for PD-1/PD-L1 blockade therapy in non-small-cell lung cancer: is PD-L1 expression a good marker for patient selection? Clin Lung Cancer. 2016; 17:350–361. PMID: 27137346.
67. Gibney GT, Weiner LM, Atkins MB. Predictive biomarkers for checkpoint inhibitor-based immunotherapy. Lancet Oncol. 2016; 17:e542–e551. PMID: 27924752.


68. Sacher AG, Gandhi L. Biomarkers for the clinical use of PD-1/PD-L1 inhibitors in non-small-cell lung cancer: a review. JAMA Oncol. 2016; 2:1217–1222. PMID: 27310809.
69. Hirsch FR, McElhinny A, Stanforth D, Ranger-Moore J, Jansson M, Kulangara K, et al. PD-L1 immunohistochemistry assays for lung cancer: results from phase 1 of the blueprint PD-L1 IHC assay comparison project. J Thorac Oncol. 2017; 12:208–222. PMID: 27913228.


70. Rimm DL, Han G, Taube JM, Yi ES, Bridge JA, Flieder DB, et al. A prospective, multi-institutional, pathologist-based assessment of 4 immunohistochemistry assays for PD-L1 expression in non-small cell lung cancer. JAMA Oncol. 2017; 3:1051–1058. PMID: 28278348.


71. Buttner R, Gosney JR, Skov BG, Adam J, Motoi N, Bloom KJ, et al. Programmed death-ligand 1 immunohistochemistry testing: a review of analytical assays and clinical implementation in non-small-cell lung cancer. J Clin Oncol. 2017; 35:3867–3876. PMID: 29053400.
72. Scheerens H, Malong A, Bassett K, Boyd Z, Gupta V, Harris J, et al. Current status of companion and complementary diagnostics: strategic considerations for development and launch. Clin Transl Sci. 2017; 10:84–92. PMID: 28121072.


73. Lawrence MS, Stojanov P, Polak P, Kryukov GV, Cibulskis K, Sivachenko A, et al. Mutational heterogeneity in cancer and the search for new cancer-associated genes. Nature. 2013; 499:214–218. PMID: 23770567.


74. Rizvi NA, Hellmann MD, Snyder A, Kvistborg P, Makarov V, Havel JJ, et al. Cancer immunology: mutational landscape determines sensitivity to PD-1 blockade in non-small cell lung cancer. Science. 2015; 348:124–128. PMID: 25765070.
Figure 1
The three phases of the cancer immunoediting process: elimination, equilibrium, and escape. DC: dendritic cell; γδ: γδ T cell; IDO: indoleamine 2,3-dioxygenase; IFN-γ: interferon γ; IL: interleukin; M1: M1 macrophage; M2: M2 macrophage; MDSC: myeloid-derived suppressor cell; NK: natural killer cell; NKT: natural killer T cell; PD-L1: programmed death ligand 1; TGF-β: transforming growth factor β; Treg: regulatory T cell. Modified from Schreiber et al. Science 2011;331:1565-70, with permission of The American Association for the Advancement of Science24.
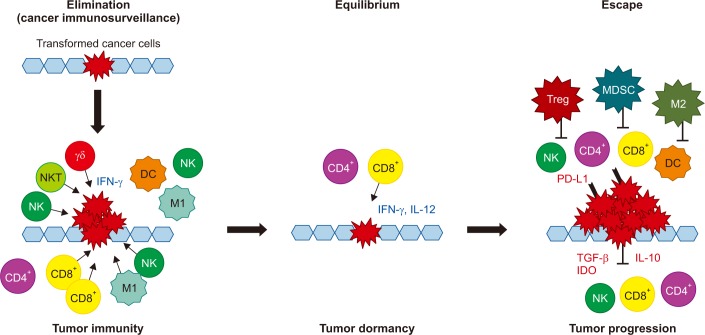
Figure 2
The cancer-immunity cycle. APC: antigen presenting cell; MHC: major histocompatibility complex; TCR: T cell receptors.
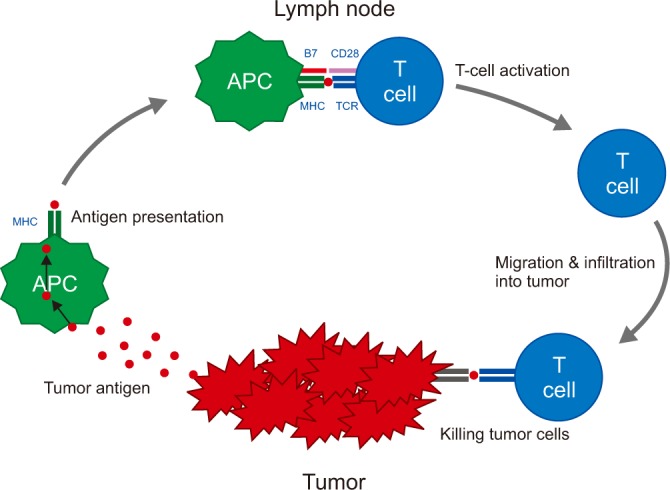
Figure 3
The immune system activation and checkpoint inhibitors. APC: antigen presenting cell; CTLA-4: cytotoxic T-lymphocyte-associated antigen 4; PD-1: programmed cell death protein 1; PD-L1: programmed death ligand 1; TCR: T cell receptors. Modified from Pardoll. Nat Rev Cancer 2012;12:252-64, with permission of Springer Nature27.
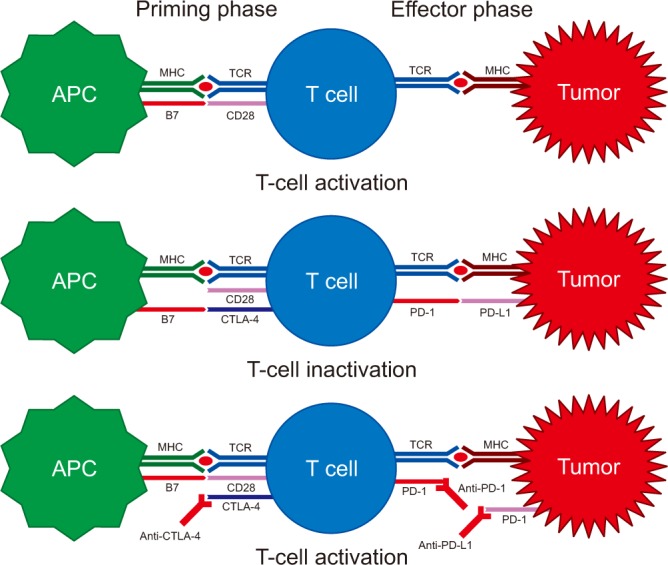
Table 1
Immune checkpoint inhibitors for the treatment of non-small cell lung cancer
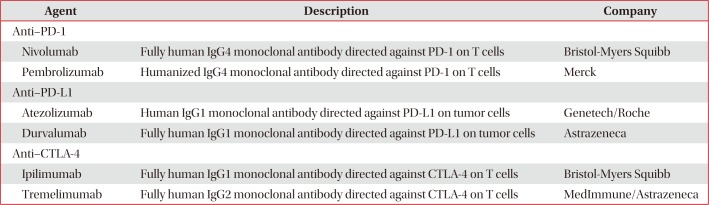
Table 2
Selected clinical trials of immune checkpoint inhibitors for treatment of non-small cell lung cancer
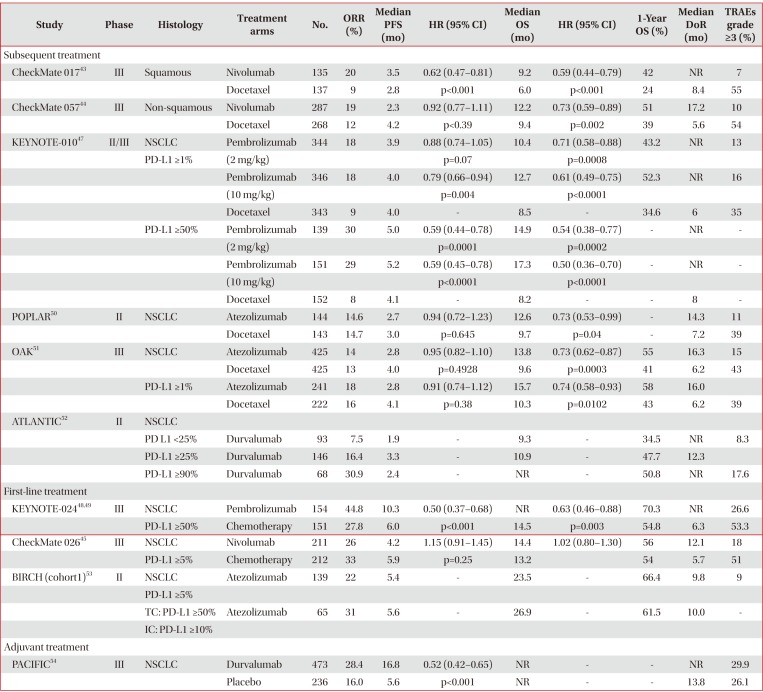
Study | Phase | Histology | Treatment arms | No. | ORR (%) | Median PFS (mo) | HR (95% CI) | Median OS (mo) | HR (95% CI) | 1-Year OS (%) | Median DoR (mo) | TRAEs grade ≥3 (%) |
---|---|---|---|---|---|---|---|---|---|---|---|---|
Subsequent treatment | ||||||||||||
CheckMate 01743 | III | Squamous | Nivolumab | 135 | 20 | 3.5 | 0.62 (0.47–0.81) | 9.2 | 0.59 (0.44–0.79) | 42 | NR | 7 |
Docetaxel | 137 | 9 | 2.8 | p<0.001 | 6.0 | p<0.001 | 24 | 8.4 | 55 | |||
CheckMate 05744 | III | Non-squamous | Nivolumab | 287 | 19 | 2.3 | 0.92 (0.77–1.11) | 12.2 | 0.73 (0.59–0.89) | 51 | 17.2 | 10 |
Docetaxel | 268 | 12 | 4.2 | p<0.39 | 9.4 | p=0.002 | 39 | 5.6 | 54 | |||
KEYNOTE-01047 | II/III | NSCLC | Pembrolizumab | 344 | 18 | 3.9 | 0.88 (0.74–1.05) | 10.4 | 0.71 (0.58–0.88) | 43.2 | NR | 13 |
PD-L1 ≥1% | (2 mg/kg) | p=0.07 | p=0.0008 | |||||||||
Pembrolizumab | 346 | 18 | 4.0 | 0.79 (0.66–0.94) | 12.7 | 0.61 (0.49–0.75) | 52.3 | NR | 16 | |||
(10 mg/kg) | p=0.004 | p<0.0001 | ||||||||||
Docetaxel | 343 | 9 | 4.0 | - | 8.5 | - | 34.6 | 6 | 35 | |||
PD-L1 ≥50% | Pembrolizumab | 139 | 30 | 5.0 | 0.59 (0.44–0.78) | 14.9 | 0.54 (0.38–0.77) | - | NR | - | ||
(2 mg/kg) | p=0.0001 | p=0.0002 | ||||||||||
Pembrolizumab | 151 | 29 | 5.2 | 0.59 (0.45–0.78) | 17.3 | 0.50 (0.36–0.70) | - | NR | - | |||
(10 mg/kg) | p<0.0001 | p<0.0001 | ||||||||||
Docetaxel | 152 | 8 | 4.1 | - | 8.2 | - | - | 8 | - | |||
POPLAR50 | II | NSCLC | Atezolizumab | 144 | 14.6 | 2.7 | 0.94 (0.72–1.23) | 12.6 | 0.73 (0.53–0.99) | - | 14.3 | 11 |
Docetaxel | 143 | 14.7 | 3.0 | p=0.645 | 9.7 | p=0.04 | - | 7.2 | 39 | |||
OAK51 | III | NSCLC | Atezolizumab | 425 | 14 | 2.8 | 0.95 (0.82–1.10) | 13.8 | 0.73 (0.62–0.87) | 55 | 16.3 | 15 |
Docetaxel | 425 | 13 | 4.0 | p=0.4928 | 9.6 | p=0.0003 | 41 | 6.2 | 43 | |||
PD-L1 ≥1% | Atezolizumab | 241 | 18 | 2.8 | 0.91 (0.74–1.12) | 15.7 | 0.74 (0.58–0.93) | 58 | 16.0 | |||
Docetaxel | 222 | 16 | 4.1 | p=0.38 | 10.3 | p=0.0102 | 43 | 6.2 | 39 | |||
ATLANTIC52 | II | NSCLC | ||||||||||
PD L1 <25% | Durvalumab | 93 | 7.5 | 1.9 | - | 9.3 | - | 34.5 | NR | 8.3 | ||
PD-L1 ≥25% | Durvalumab | 146 | 16.4 | 3.3 | - | 10.9 | - | 47.7 | 12.3 | |||
PD-L1 ≥90% | Durvalumab | 68 | 30.9 | 2.4 | - | NR | - | 50.8 | NR | 17.6 | ||
First-line treatment | ||||||||||||
KEYNOTE-02448,49 | III | NSCLC | Pembrolizumab | 154 | 44.8 | 10.3 | 0.50 (0.37–0.68) | NR | 0.63 (0.46–0.88) | 70.3 | NR | 26.6 |
PD-L1 ≥50% | Chemotherapy | 151 | 27.8 | 6.0 | p<0.001 | 14.5 | p=0.003 | 54.8 | 6.3 | 53.3 | ||
CheckMate 02645 | III | NSCLC | Nivolumab | 211 | 26 | 4.2 | 1.15 (0.91–1.45) | 14.4 | 1.02 (0.80–1.30) | 56 | 12.1 | 18 |
PD-L1 ≥5% | Chemotherapy | 212 | 33 | 5.9 | p=0.25 | 13.2 | 54 | 5.7 | 51 | |||
BIRCH (cohort1)53 | II | NSCLC | Atezolizumab | 139 | 22 | 5.4 | - | 23.5 | - | 66.4 | 9.8 | 9 |
PD-L1 ≥5% | ||||||||||||
TC: PD-L1 ≥50% | Atezolizumab | 65 | 31 | 5.6 | - | 26.9 | - | 61.5 | 10.0 | - | ||
IC: PD-L1 ≥10% | ||||||||||||
Adjuvant treatment | ||||||||||||
PACIFIC54 | III | NSCLC | Durvalumab | 473 | 28.4 | 16.8 | 0.52 (0.42–0.65) | NR | - | - | NR | 29.9 |
Placebo | 236 | 16.0 | 5.6 | p<0.001 | NR | - | - | 13.8 | 26.1 |