Abstract
Chronic obstructive pulmonary disease (COPD) is associated with abnormal inflammatory response and airflow limitation. Acute exacerbation involves increased inflammatory burden leading to worsening respiratory symptoms, including dyspnea and sputum production. Some COPD patients have frequent exacerbations (two or more exacerbations per year). A substantial proportion of COPD patients may remain stable without exacerbation. Bacterial and viral infections are the most common causative factors that breach airway stability and lead to exacerbation. The increasing prevalence of exacerbation is associated with deteriorating lung function, hospitalization, and risk of death. In this review, we summarize the mechanisms of airway inflammation in COPD and discuss how bacterial or viral infection, temperature, air pollution, eosinophilic inflammation, and concomitant chronic diseases increase airway inflammation and the risk of exacerbation.
Chronic obstructive pulmonary disease (COPD) is defined as a common, preventable, and treatable disease that is characterized by persistent respiratory symptoms and airflow limitation caused by exposure to noxious particles or gases. The progressive airflow limitation is attributed to chronic inflammation leading to small airway obstruction and parenchymal tissue destruction1. Exacerbation is defined as an acute worsening of respiratory symptoms1 that may be associated with the increase of airway inflammation mediated by neutrophils; lymphocytes; eosinophils; and the associated mediators, such as interleukin-8 (IL-8), regulated on activation, normal T cell expressed and secreted, and neutrophils elastase2. The causes of exacerbation are complex and mainly include respiratory, viral, or bacterial infections, and environmental pollution3, which lead to an increase in inflammatory burden and a decrease in protective defense by host immunity. In the Evaluation of COPD Longitudinally to Identify Predictive Surrogate Endpoints (ECLIPSE) study4, which is a clinical observation on COPD patients over a period of 3 years, the exacerbation frequency appeared to be linked to prior history of exacerbation and severity of diseases. COPD patients with frequent exacerbations during the previous year tended to have higher susceptibility to exacerbations in the following years. What is the underlying mechanism to explain this frequent-exacerbation phenotype of COPD? What is breach of stability of airway inflammation and what triggers exacerbation? The co-existing eosinophilic inflammation and concomitant chronic diseases will be discussed (Figure 1).
The airflow limitation in COPD is associated with abnormal inflammatory response. Progression of airflow limitation is associated with the increase in accumulation of mucus exudates in the lumen and infiltration of the small airway wall by inflammatory cells5. A variety of inflammatory cells are present in the airways of patients with COPD. Neutrophils, macrophages, eosinophils, mast cells, and CD8+ lymphocytes have been shown to play important roles in the inflammatory processes in COPD6789.
Neutrophils are one of the major inflammatory cell types in COPD5. Increased numbers of neutrophils in sputum were found to be correlated with a rapid decline in patients' forced expiratory volume in one second (FEV1) in a 15-year period of observation10. In stable COPD patients, FEV1 showed a significant inverse correlation with the number of neutrophils in induced sputum11 and in the sub-epithelium from bronchial biopsies12, suggesting that neutrophilic inflammation of the airways may play an important role in the pathogenesis of COPD.
Macrophages can be activated by cigarette smoke to release inflammatory mediators and matrix metalloproteases (MMPs). The number of alveolar macrophages was correlated to the extent of emphysema formation13 and to the severity of airway obstruction12. The MMPs, Zn2+-dependent proteases, and Ca2+-dependent proteases can degrade a broad range of connective tissue proteins and are involved in remodeling damaged tissue. Increased activity of MMP-8 (collagenase) and MMP-9 (gelatinase) was detected in induced sputum from patients with COPD, and this activity was correlated to the degree of airflow limitation14. In the animal model of knockout mice, MMP-12 deficiency can block the increase in macrophages in the lungs and the development of emphysema in response to long-term exposure to cigarette smoke15. In addition, MMP-12 mediates smoke-induced inflammation by releasing tumor necrosis factor α (TNF-α) from macrophages with subsequent endothelial activation, neutrophil influx, and proteolytic matrix breakdown caused by neutrophil-derived proteases16.
The role of eosinophils in COPD and the mechanism of eosinophil influx into airways remain to be clarified. Hogg et al.5 reported that eosinophils do exist in the small airways of various severity of COPD. In a prospective clinical observation, nearly one third of COPD patients had sputum eosinophilia and the number of eosinophils was significantly correlated to the level of exhaled nitric oxide17. The degree of eosinophilic inflammation has been related to early changes in lung function and smoking habits. The higher counts of eosinophils in induced sputum is associated with higher pack-years and lower peak expiratory flow 25%–75% values18. In a retrospective analysis, FEV1 reversibility was weakly correlated with the sputum eosinophil level. Patients with FEV1 >0.4 L and >15% increment had higher sputum eosinophil levels whereas the level did not differ when dichotomized by FEV1 increment >0.2 L and >12%19. The response to corticosteroids in COPD patients were better in a subset of patients presenting with more eosinophils and higher levels of eosinophil cationic protein20, suggesting that corticosteroids may be effective especially for patients with COPD with eosinophilic airway inflammation2122. In addition, we had reported that inhaled fluticasone (200 µg/day) can significantly suppress the numbers of eosinophils in the airways of COPD. Suppression of such eosinophilic inflammation was not associated with improvement in lung function. Brightling et al.23 also reported that the improvement in FEV1 is not associated with a reduction in the sputum eosinophil count in COPD with sputum eosinophilia.
Increased CD8+ T lymphocytes have been shown in the central and peripheral airways and lung parenchyma in COPD12132425. The increased number of CD8+ T lymphocytes in the subepithelium has been correlated with the severity of airflow limitation26. Increased cytotoxic activity of CD8+ T cells was observed in induced sputum of patients with COPD compared to those of smokers without COPD and healthy subjects. The expression of perforins, a secreted molecule that causes cytolysis, was also high27. However, one in vitro study demonstrated that cytolytic activity of CD8+ T cells against alveolar epithelial cells is perforin-independent and is attributed entirely by TNF-α, which is expressed on CD8+ T cells28. However, the contribution of CD8+ T cell and the ratio of CD4+/CD8+ cells to the pathogenesis of COPD as well as the cell recruitment to the airways are not clear.
Acute exacerbation is associated with increased inflammatory burden leading to worsening respiratory symptoms including dyspnea and sputum production. In the ECLIPSE study, the exacerbation rates were 0.85, 1.34, and 2.0 (number/yr/patient) for patients with Global Initiative for Obstructive Lung Disease (GOLD) stages 2, 3, and 4, respectively, during the first year of follow-up4. The exacerbation rates were significantly associated with post-bronchodilator FEV1, impairment in health status, history of reflux or heartburn, white blood cell count, and prior history of exacerbation. In addition, acute exacerbation of COPD had been found to be an independent factor of mortality, suggesting more exacerbations with higher mortality29. In a subgroup analysis in the Inhaled Steroids in Obstructive Lung Disease in Europe (ISOLDE) study, 39% and 36% of patients with or without inhaled corticosteroid treatment had no exacerbation in the first year of follow-up. During the three-year study period, 22% and 23% of patients respectively had no exacerbations30. In a retrospective observational study, 85.5%, 80.4%, 60.6%, and 48.1% of COPD patients with GOLD classification group A, B, C, and D had no acute exacerbation in the previous year before enrollment31.
In a prospective observational study, 55% and 29% of COPD patients had bacteria or virus-associated exacerbations, respectively32. The most common causes of bacteria-associated exacerbations were Haemophilus influenzae, Moraxella catarrhalis, and Streptococcus pneumoniae33. The major viruses associated with COPD exacerbations were rhinovirus (common cold), coronavirus, influenza, and parainfluenza34. Papi et al.35 reported that patients with infectious exacerbation (bacteria, virus, or coinfection) may have longer hospitalization and greater impairment of lung function compared to those non-infectious exacerbations. Sputum neutrophilia were observed in all exacerbations and was associated with disease severity. Sputum eosinophilia was observed during virus-associated exacerbations35.
Normally, the lower airways are sterile. Bacterial components such as flagella, lipoproteins, and lipopolysaccharides may initiate inflammatory reactions by releasing a variety of pro-inflammatory mediators and recruiting inflammatory cells36. For example, protein A from staphylococci can recognize TNF-α receptors in airway epithelium and induce production of IL-8, which is an important chemokine for neutrophils37. Pseudomonas aeruginosa flagella can activate airway epithelial cells to produce IL-8 through toll-like receptors 2 and 538. In stable COPD patients, sputum neutrophils and IL-8 levels were higher than those in healthy subjects, which suggested ongoing neutrophilic inflammation in the airways11. Sputum IL-8 levels were reported to be correlated with total bacterial count, and bacterial load in the airways was associated with increased exacerbation frequency39. Rhinovirus infection can increase the production of IL-8, IL-6, and granulocyte-macrophage colony-stimulating factor (GM-CSF) from airway epithelial cells; IL-8 production was associated with viral replication40. In a study in mice, influenza virus infection resulted in influx of neutrophils, monocytes, lymphocytes, and eosinophils as well as various chemokines and cytokines 3 to 10 days after infection41.
Increased concentration of outdoor pollutants such as NO2, O3, SO2, particulate matter 2.5 (PM2.5), and particulate matter 10 (PM10) were associated with increased respiratory symptoms, acute exacerbations, and hospital admissions in COPD patients42. Inhalation of high concentration of NO2 may correlate to acute epithelial damage43. Cultured human bronchial epithelial cells exposed to NO2 could increase the expression of IL-1β, IL-8, GM-CSF, and TNF-α44. SO2 can lead to airway edema, inflammation, and fibrosis in an animal model after 3–25 days' exposure to different levels of SO245. O3 and PM10 have been reported to increase the incidence of hospital admission for pneumonia and acute exacerbation of COPD in a meta-analysis based on city-specific regression models46. Short-term PM2.5 exposure was associated with an increased risk of about 0.9% for COPD hospitalization47. In an epidemiologic study, for every 10 µg/m3 increase in O3, NO2, and SO2 concentrations, the risks of hospitalization for COPD increase to about 0.58%, 0.38%, and 0.44%, respectively48. Seasonal variations and temperature may affect the incidence of acute exacerbation of COPD. Tseng et al.49 reported that a 1℃ decrease in air temperature was associated with a 0.8% increase in the exacerbation rate on event-days. The cold temperature was reported to be linked to bronchoconstriction50 and FEV1 decrease51 especially for COPD patients.
The gastroesophageal refluxate contains gastric acids, bile, and pepsin. Gastroesophageal reflux (GER) is a major cause of subacute and chronic cough. In a questionnaire-based prospective survey, the prevalence of GER disease (GERD) was 26.7% among stable COPD patients, and severe GERD symptoms were associated with more frequent exacerbations of COPD and hospitalization with crude relative risks of 3.42 and 3.66, respectively51. Casanova et al.52 reported that severe COPD patients had high prevalence of GERD (62%) confirmed by esophageal 24-hour pH monitoring but 58% of them did not have any reflux symptoms. In addition, the annual rate of exacerbations of COPD was twice as high in patients with GER symptoms compared to those without GER symptoms (3.2/yr vs. 1.6/yr, p=0.02)53. In our previous study, the amount of total bile acids in induced sputum was significantly higher in patients with GER and asthma-associated GER symptoms compared to that of healthy control subjects (p<0.005). Bile acid significantly induced transforming growth factor β1 (TGF-β1) production from airway epithelial cells, which in turn enhanced fibroblast proliferation54 and altered alveolar epithelial permeability, which may contribute to the pathogenesis of lung injury55. Airway epithelium exposed to bile acids could induce fibrosis via production of connective tissue growth factor (CCN2). CCN2 is essential for TGF-β1–induced fibrogenesis and functions as a downstream mediator of TGF-β1 action on fibroblasts56. The major bile acid, chenodeoxycholic acid, stimulated alveolar epithelial cells to increase IL-8 production through p38 and c-Jun N-terminal kinase activation. This may enhance neutrophilic inflammation in chronic airway disorders57. How to intervene and attenuate GER-related inflammation and fibrogenesis in chronic airway disorders is worth further investigation.
The prevalence of bronchiectasis in COPD varies according to COPD severity. In a prospective observational study, 50% of moderate to severe COPD patients had significant detectable bronchiectasis on high-resolution computed tomography scanning. Lower lobe bronchiectasis was associated with bacterial colonization quantified by sputum sampling58. Martinez-Garcia et al.59 reported that the prevalence of bronchiectasis in moderate and severe COPD patients was 34.7% and 72.5%, respectively. Patients with bronchiectasis had more chronic expectoration, more exacerbation indices, more severe airflow obstruction, and greater positive cultures of potentially pathogenic microorganism. Haemophilus influenzae and Pseudomonas aeruginosa were the most common microorganisms isolated from sputum59. In addition, moderate-to-severe COPD patients with bronchiectasis was associated with increased all-cause mortality (37.4%) compared to COPD patients without bronchiectasis (9.3%)60.
Eosinophilic airway inflammation is a characteristic feature of atopic asthma and exists in a subgroup of COPD. Bafadhel et al.32 reported that 28% of cases of acute exacerbation of COPD were associated with sputum eosinophilia. In our previous study, 32% of naïve COPD patients had sputum eosinophilia and tended to have higher serum IgE levels and positive allergen tests17. Patients with asthma-COPD overlap syndrome may present with more respiratory symptoms, worse lung function, and increased risk of exacerbation and hospitalization (prevalence ratio, 2.11 and 4.11, respectively) compared to patients with COPD alone61. Hardin et al.62 reported that patients with asthma and COPD overlap syndrome (ACOS) were more likely to experience frequent exacerbations (odds ratio, 3.55) and have had a severe exacerbation in the past years. In a prospective randomized study, a reduction in eosinophilic airway inflammation was associated with a reduction in severe exacerbation of COPD63. Sputum eosinophilia and high blood eosinophil counts are associated with increased responsiveness to steroids in COPD patients22236465. Accordingly, the detection of eosinophilic inflammation is a crucial step to make a precise treatment in COPD patients.
In an epidemiologic study for COPD, the risk of obstructive sleep apnea (OSA) assessed by questionnaire was 29.5% among COPD patients66. The prevalence was reported to be 65.9% for moderate to severe COPD confirmed by polysomnography67. Marin et al.68 showed that COPD patients with OSA overlap syndrome without continuous positive airway pressure (CPAP) treatment had higher mortality rates and were more likely to have a severe COPD exacerbation leading to hospitalization (relative risk, 1.70; 95% confidence interval [CI], 1.21–2.38) compared to patients with COPD alone. OSA exacerbated airway inflammation by increasing neutrophil proportion and levels of IL-8 and TNF-α in bronchoalveolar lavage fluid samples from moderate to severe COPD patients. The augmentation of inflammation can be curbed by treatment with CPAP69. The mechanisms of how OSA exacerbates airway inflammation in COPD remain to be elucidated.
The significant predictors or factors that are associated with increased risk of acute exacerbation in COPD is summarized in Table 1.
Long-acting bronchodilators such as long-acting muscarinic antagonist (LAMA) and long acting β2-adrenergic receptor agonist (LABA) are the major pharmacological drugs for COPD to improve airflow limitation, respiratory symptoms, and quality of life as well as reduce exacerbations7071. The mechanisms of long-acting bronchodilators in reducing exacerbation either by improving hyperinflation or targeting inflammation remain controversial. The combination of LABA and corticosteroids can reduce the numbers of macrophages72, neutrophils73, and CD8+ T lymphocytes7273 in bronchial biopsy tissue and in induced sputum from patients with COPD. We had reported that the combination of inhaled salmeterol and fluticasone caused a significant reduction in IL-8 and MMP-9 in induced sputum from COPD patients compared to levels in those who received treatment with tiotropium alone74. Tiotropium can inhibit airway inflammation and remodeling75 and attenuate neutrophilic inflammation as well as associated mediators LTB4, IL-6, monocyte chemoattractant protein-1, macrophage inflammatory protein (MIP)-1, MIP-2, and TNF-α in the animal model76. In clinical studies, the odds ratio for tiotropium in reducing exacerbations compared to placebo was 0.74 (95% CI, 0.66–0.83) and hospitalizations 0.69 (95% CI, 0.55–0.87)77. Indacaterol and formoterol can suppress neutrophil-associated mediators such as reactive oxygen species, LTB4, and elastase in a dose-dependent manner78. In a prospective, randomized, blinded, and double-dummy study, it was found that tiotropium was superior to indacaterol in terms of annualized exacerbation rate (0.61 vs. 0.79) for severe COPD79. A combination of indacaterol and glycopyrronium significantly reduced the annual rate of moderate to severe exacerbations versus glycopyrronium alone by 12%, suggesting that the combination of indacaterol/glycopyrronium was superior in preventing moderate to severe exacerbations compared to glycopyrronium alone80. Roflumilast, a]' phosphodiesterase-4 (PDE4) inhibitor, has been reported to significantly reduce moderate to severe exacerbations by 17% compared to placebo for severe COPD patients with chronic bronchitis and frequent exacerbation phenotype81. PDE4 inhibitors were associated with a reduced likelihood of COPD exacerbation with odds ratio 0.77 (95% CI, 0.71–0.83) in a pooled analysis82. Choosing the optimal LABA, LAMA, dual bronchodilator, PDE4 inhibitor, inhaled corticosteroids, or specific combination of different drugs for COPD patients with various severity and phenotype is challenging in our clinical practice.
Pneumococcal and influenza vaccinations have been recommended for patients with COPD as a preventive strategy1. A recent meta-analysis showed that pneumococcal vaccination can provide significant protection against community-acquired pneumonia (CAP) and reduce the likelihood of a COPD exacerbation83. By contrast, Sehatzadeh84 reported that the time to the first episode of CAP due to pneumococcus or of unknown etiology was not significantly different between the vaccine and control groups. Nevertheless, hospital admission rates and median lengths of hospital stay were lower in the vaccine group but were not statistically significant84. For influenza vaccination, it was found that the vaccine group had fewer episodes of influenza-related acute respiratory illnesses in all severities (mild, moderate, and severe) of COPD, fewer hospitalizations, and fewer requirements for mechanical ventilation84.
It is necessary to promote and conduct research on ambient air pollution as a COPD risk factor. It is necessary to form a partnership with government institutions such as the Environmental Protection Agency (EPA) and academic institutions to investigate the detrimental effect of environmental exposure and occupational hazards as well as to apply prevention strategy and patient education. The U.S. EPA has recommended that time and intensity of outdoor activity should be regulated by air quality index since 2009. The N95 respirator was reported to be able to provide excellent protection against airborne particles85.
Aspiration of gastroesophageal refluxate can trigger exacerbation of COPD and enhance airway inflammation and fibrosis as we discussed earlier. Among COPD patients with GERD, those who did not use acid inhibitory treatment regularly had a significantly increased risk of COPD exacerbation with a hazard ratio (HR) of 2.7 as compared to those who were treated regularly with an HR of 1.286. In moderate to severe asthma patients with GERD and nocturnal respiratory symptoms, taking proton pump inhibitors significantly improved morning and evening peak expiratory flow over those taking palcebo87. The ability to identify COPD patients who are at risk for GERD or GER-related chronic cough is essential to COPD management. It is worth conducting randomized trials to investigate the efficacy of anti-acid and anti-reflux therapy on COPD patients with GERD and whether these treatments can decrease respiratory symptoms, improve lung function, and quality of life as well as reduce exacerbation.
In a prospective study, the incidence of bacterial colonization in bronchiectasis was as high as 64%. The risk factors included diagnosis of bronchiectasis before the age of 14 years (odds ratio [OR], 3.92), FEV1 <80% predicted (OR, 3.91) and presence of varicose or cystic bronchiectasis (OR, 4.8)88. Bacterial infection can cause airway inflammation, which can subsequently result in exacerbation. COPD patients with bronchiectasis have more respiratory symptoms, more exacerbations, and worse prognosis. Azithromycin 250 mg taken daily for 1 year, decreased the frequency of exacerbations (HR, 0.73; p<0.001) and improved the quality of life among COPD patients89. The clinical efficacy on hospitalization, urgent care visit, and intubation was similar between both groups and the adverse reaction (hearing impairment) was more common in the azithromycin group. Uzun et al.90 reported that azithromycin 500 mg taken 3 times a week for 12 months resulted in a significant reduction in the exacerbation rate versus placebo (HR, 0.58; p=0.001) among COPD patients who had 3 or more exacerbations in the previous year. A small study demonstrated that inhaled tobramycin reduced the inflammatory impact of Pseudomonas aeruginosa in a multiresistant Pseudomonas-colonized patient with severe COPD after 2-week treatment. A 42% reduction in exacerbation rate was observed in the 6-month follow-up91. The ability to select patients, choose appropriate antibiotics, decide the duration of treatment are the major concerns for treatment strategy for frequent exacerbations of COPD with bacterial colonization.
Whether eosinophilic inflammation in COPD is attributable to ACOS or is a specific phenotype of COPD is still controversial. However, the response of eosinophilic inflammation to treatment would be more important than the delineation of the differences between these two terms. Early detection of characteristic features of asthma or eosinophilic airway inflammation in COPD patients is necessary before starting treatment. Zanini et al.92 reported that COPD patients with bronchial hyper-responsiveness (BHR) had higher sputum eosinophils than those without BHR. The change in FEV1 was positively correlated with sputum eosinophils while patients had bronchial reversibility1992. The prevalence of elevated serum IgE had been reported to be 47.3% in COPD patients93. Serum IgE levels were associated with COPD severity94 and sputum eosinophilia17. The levels of eosinophils in sputum samples were significantly correlated with exhaled nitric oxide level (eNO)17. The eNO, blood eosinophils, and antigenspecific IgE were significantly higher in ACOS than in COPD alone according to a cross-sectional study in Japan95. Blood eosinophils were proposed to be a surrogate marker for airway eosinophilia in stable COPD96 and a marker of response to inhaled corticosteroids97. COPD patients with blood eosinophils ≥2% treated with inhaled corticosteroid/LABA had significantly fewer exacerbations than those treated with LAMA (relative risk [RR], 0.75; p=0.006, INSPIRE study) or placebo (RR, 0.63; p<0.001)98. It is warranted to conduct a prospective study to determine which marker is better in predicting corticosteroid response in COPD with eosinophilic airway inflammation.
CPAP is considered to be the standard treatment for OSA patients. The benefit of CPAP treatment on reducing airway inflammation in COPD is barely discussed. CPAP may reduce oxidative stress by decreasing plasma malondialdehyde in OSA99 and significantly reduce urinary norepinephrine levels and blood pressure in patients with severe OSA100. Briefly, OSA may exacerbate airway inflammation in COPD and CPAP is the optimal choice to reverse the inflammatory process so far.
Though airway inflammation in COPD varies based on severity, there is still a gap between inflammation and exacerbation. Bacterial or viral infection, air pollution, GERD, bronchiectasis with bacterial colonization, and eosinophils may breach the airway stability and lead to overwhelming inflammation and acute exacerbation. The application of antiinflammatory agents and vaccines, prevention strategies for air pollution, early detection of GERD, reduction of bacterial load in bronchiectasis, and measurement of biomarkers that can predict response to corticosteroids are becoming the therapeutic goals to minimize the risks of acute exacerbation. We still need more evidence and clinical studies to achieve these endpoints and improve health-related quality of life among COPD patients.
Figures and Tables
![]() | Figure 1The factors that augment airway inflammation and lead to exacerbation. GERD: gastroesophageal reflux disease; OSA: obstructive sleep apnea. |
Table 1
Significant predictors/factors associated with AECOPD/hospitalizations
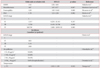
Odds ratio or relative risk | 95% CI | p-value | Reference | |
---|---|---|---|---|
GERD | 3.42 | 2.02–5.69 | Takada et al.51 | |
Bronchiectasis | 3.07 | 1.07–8.77 | 0.037 | Martinez-Garcia et al.59 |
Eosinophilia | 4.20 | 1.05–16.62 | 0.069 | Menezes et al.61 |
OSA | 1.70 | 1.21–2.38 | 0.002 | Marin et al.68 |
GOLD stage | Takada et al.51 | |||
I | 1 | |||
II | 3.117 | 0.291–33.413 | 0.347 | |
III | 4.702 | 0.419–52.760 | 0.209 | |
IV | 108.704 | 3.883–3,099.135 | 0.005 |
Incidence (number/yr/patient) | ||||
---|---|---|---|---|
GOLD stage | Hurst et al.4 | |||
I | - | - | - | |
II | 0.85 | - | - | |
III | 1.34 | - | - | |
IV | 2.00 | - | - | |
Air pollution | Ghozikali et al.48 | |||
↑ O3 10 µg/m3 | ↑0.58% | - | - | |
↑ NO2 10 µg/m3 | ↑0.38% | - | - | |
↑ SO2 10 µg/m3 | ↑0.44% | - | - | |
↑ PM2.5 10 µg/m3 | ↑0.9% (hospitalization) | - | - | Dominici et al.47 |
Temperature | ||||
↓ 1°C | 0.8% | 1.015–1.138 | 0.015 | Tseng et al.49 |
References
1. National Institutes of Health. National Heart, Lung, and Blood Institute. Global Initiative for Chronic Obstructive Lung Disease: global strategy for the diagnosis, management, and prevention of chronic obstructive pulmonary disease. NHLBI/WHO workshop report, publication No. 2701. Bethesda, MD: National Institutes of Health; National Heart, Lung, and Blood Institute;2001.
2. Fujimoto K, Yasuo M, Urushibata K, Hanaoka M, Koizumi T, Kubo K. Airway inflammation during stable and acutely exacerbated chronic obstructive pulmonary disease. Eur Respir J. 2005; 25:640–646.
3. Sapey E, Stockley RA. COPD exacerbations. 2: aetiology. Thorax. 2006; 61:250–258.
4. Hurst JR, Vestbo J, Anzueto A, Locantore N, Mullerova H, Tal-Singer R, et al. Susceptibility to exacerbation in chronic obstructive pulmonary disease. N Engl J Med. 2010; 363:1128–1138.
5. Hogg JC, Chu F, Utokaparch S, Woods R, Elliott WM, Buzatu L, et al. The nature of small-airway obstruction in chronic obstructive pulmonary disease. N Engl J Med. 2004; 350:2645–2653.
6. Balzano G, Stefanelli F, Iorio C, De Felice A, Melillo EM, Martucci M, et al. Eosinophilic inflammation in stable chronic obstructive pulmonary disease: relationship with neutrophils and airway function. Am J Respir Crit Care Med. 1999; 160(5 Pt 1):1486–1492.
7. Hattotuwa KL, Gizycki MJ, Ansari TW, Jeffery PK, Barnes NC. The effects of inhaled fluticasone on airway inflammation in chronic obstructive pulmonary disease: a double-blind, placebo-controlled biopsy study. Am J Respir Crit Care Med. 2002; 165:1592–1596.
8. Lacoste JY, Bousquet J, Chanez P, Van Vyve T, Simony-Lafontaine J, Lequeu N, et al. Eosinophilic and neutrophilic inflammation in asthma, chronic bronchitis, and chronic obstructive pulmonary disease. J Allergy Clin Immunol. 1993; 92:537–548.
9. Peleman RA, Rytila PH, Kips JC, Joos GF, Pauwels RA. The cellular composition of induced sputum in chronic obstructive pulmonary disease. Eur Respir J. 1999; 13:839–843.
10. Stanescu D, Sanna A, Veriter C, Kostianev S, Calcagni PG, Fabbri LM, et al. Airways obstruction, chronic expectoration, and rapid decline of FEV1 in smokers are associated with increased levels of sputum neutrophils. Thorax. 1996; 51:267–271.
11. Perng DW, Huang HY, Chen HM, Lee YC, Perng RP. Characteristics of airway inflammation and bronchodilator reversibility in COPD: a potential guide to treatment. Chest. 2004; 126:375–381.
12. Di Stefano A, Capelli A, Lusuardi M, Balbo P, Vecchio C, Maestrelli P, et al. Severity of airflow limitation is associated with severity of airway inflammation in smokers. Am J Respir Crit Care Med. 1998; 158:1277–1285.
13. Finkelstein R, Fraser RS, Ghezzo H, Cosio MG. Alveolar inflammation and its relation to emphysema in smokers. Am J Respir Crit Care Med. 1995; 152(5 Pt 1):1666–1672.
14. Vernooy JH, Lindeman JH, Jacobs JA, Hanemaaijer R, Wouters EF. Increased activity of matrix metalloproteinase-8 and matrix metalloproteinase-9 in induced sputum from patients with COPD. Chest. 2004; 126:1802–1810.
15. Hautamaki RD, Kobayashi DK, Senior RM, Shapiro SD. Requirement for macrophage elastase for cigarette smoke-induced emphysema in mice. Science. 1997; 277:2002–2004.
16. Churg A, Wang RD, Tai H, Wang X, Xie C, Dai J, et al. Macrophage metalloelastase mediates acute cigarette smoke-induced inflammation via tumor necrosis factor-alpha release. Am J Respir Crit Care Med. 2003; 167:1083–1089.
17. Chou KT, Su KC, Huang SF, Hsiao YH, Tseng CM, Su VY, et al. Exhaled nitric oxide predicts eosinophilic airway inflammation in COPD. Lung. 2014; 192:499–504.
18. Dippolito R, Foresi A, Chetta A, Castagnaro A, Malorgio R, Marangio E, et al. Eosinophils in induced sputum from asymptomatic smokers with normal lung function. Respir Med. 2001; 95:969–974.
19. Chou KT, Su KC, Hsiao YH, Huang SF, Ko HK, Tseng CM, et al. Post-bronchodilator reversibility of FEV1 and eosinophilic airway inflammation in COPD. Arch Bronconeumol. 2017; 04. 1529. DOI: 10.1016/j.arbres.2017.01.014. [Epub].
20. Chanez P, Vignola AM, O'Shaugnessy T, Enander I, Li D, Jeffery PK, et al. Corticosteroid reversibility in COPD is related to features of asthma. Am J Respir Crit Care Med. 1997; 155:1529–1534.
21. Fujimoto K, Kubo K, Yamamoto H, Yamaguchi S, Matsuzawa Y. Eosinophilic inflammation in the airway is related to glucocorticoid reversibility in patients with pulmonary emphysema. Chest. 1999; 115:697–702.
22. Brightling CE, Monteiro W, Ward R, Parker D, Morgan MD, Wardlaw AJ, et al. Sputum eosinophilia and short-term response to prednisolone in chronic obstructive pulmonary disease: a randomised controlled trial. Lancet. 2000; 356:1480–1485.
23. Brightling CE, McKenna S, Hargadon B, Birring S, Green R, Siva R, et al. Sputum eosinophilia and the short term response to inhaled mometasone in chronic obstructive pulmonary disease. Thorax. 2005; 60:193–198.
24. Saetta M, Di Stefano A, Turato G, Facchini FM, Corbino L, Mapp CE, et al. CD8+ T-lymphocytes in peripheral airways of smokers with chronic obstructive pulmonary disease. Am J Respir Crit Care Med. 1998; 157(3 Pt 1):822–826.
25. Majo J, Ghezzo H, Cosio MG. Lymphocyte population and apoptosis in the lungs of smokers and their relation to emphysema. Eur Respir J. 2001; 17:946–953.
26. O'Shaughnessy TC, Ansari TW, Barnes NC, Jeffery PK. Inflammation in bronchial biopsies of subjects with chronic bronchitis: inverse relationship of CD8+ T lymphocytes with FEV1. Am J Respir Crit Care Med. 1997; 155:852–857.
27. Chrysofakis G, Tzanakis N, Kyriakoy D, Tsoumakidou M, Tsiligianni I, Klimathianaki M, et al. Perforin expression and cytotoxic activity of sputum CD8+ lymphocytes in patients with COPD. Chest. 2004; 125:71–76.
28. Liu AN, Mohammed AZ, Rice WR, Fiedeldey DT, Liebermann JS, Whitsett JA, et al. Perforin-independent CD8(+) T-cell-mediated cytotoxicity of alveolar epithelial cells is preferentially mediated by tumor necrosis factor-alpha: relative insensitivity to Fas ligand. Am J Respir Cell Mol Biol. 1999; 20:849–858.
29. Soler-Cataluna JJ, Martinez-Garcia MA, Roman Sanchez P, Salcedo E, Navarro M, Ochando R. Severe acute exacerbations and mortality in patients with chronic obstructive pulmonary disease. Thorax. 2005; 60:925–931.
30. Jones PW, Willits LR, Burge PS, Calverley PM. Inhaled Steroids in Obstructive Lung Disease in Europe Study Investigators. Disease severity and the effect of fluticasone propionate on chronic obstructive pulmonary disease exacerbations. Eur Respir J. 2003; 21:68–73.
31. Wei YF, Kuo PH, Tsai YH, Tao CW, Cheng SL, Lee CH, et al. Factors associated with the prescription of inhaled corticosteroids in GOLD group A and B patients with COPD: subgroup analysis of the Taiwan obstructive lung disease cohort. Int J Chron Obstruct Pulmon Dis. 2015; 10:1951–1956.
32. Bafadhel M, McKenna S, Terry S, Mistry V, Reid C, Haldar P, et al. Acute exacerbations of chronic obstructive pulmonary disease: identification of biologic clusters and their biomarkers. Am J Respir Crit Care Med. 2011; 184:662–671.
33. Sethi S, Murphy TF. Bacterial infection in chronic obstructive pulmonary disease in 2000: a state-of-the-art review. Clin Microbiol Rev. 2001; 14:336–363.
34. Wedzicha JA. Role of viruses in exacerbations of chronic obstructive pulmonary disease. Proc Am Thorac Soc. 2004; 1:115–120.
35. Papi A, Bellettato CM, Braccioni F, Romagnoli M, Casolari P, Caramori G, et al. Infections and airway inflammation in chronic obstructive pulmonary disease severe exacerbations. Am J Respir Crit Care Med. 2006; 173:1114–1121.
36. Gomez MI, Prince A. Airway epithelial cell signaling in response to bacterial pathogens. Pediatr Pulmonol. 2008; 43:11–19.
37. Gomez MI, Lee A, Reddy B, Muir A, Soong G, Pitt A, et al. Staphylococcus aureus protein A induces airway epithelial inflammatory responses by activating TNFR1. Nat Med. 2004; 10:842–848.
38. Adamo R, Sokol S, Soong G, Gomez MI, Prince A. Pseudomonas aeruginosa flagella activate airway epithelial cells through asialoGM1 and toll-like receptor 2 as well as toll-like receptor 5. Am J Respir Cell Mol Biol. 2004; 30:627–634.
39. Patel IS, Seemungal TA, Wilks M, Lloyd-Owen SJ, Donaldson GC, Wedzicha JA. Relationship between bacterial colonisation and the frequency, character, and severity of COPD exacerbations. Thorax. 2002; 57:759–764.
40. Subauste MC, Jacoby DB, Richards SM, Proud D. Infection of a human respiratory epithelial cell line with rhinovirus: induction of cytokine release and modulation of susceptibility to infection by cytokine exposure. J Clin Invest. 1995; 96:549–557.
41. Buchweitz JP, Harkema JR, Kaminski NE. Time-dependent airway epithelial and inflammatory cell responses induced by influenza virus A/PR/8/34 in C57BL/6 mice. Toxicol Pathol. 2007; 35:424–435.
42. Ko FW, Hui DS. Air pollution and chronic obstructive pulmonary disease. Respirology. 2012; 17:395–401.
43. Persinger RL, Blay WM, Heintz NH, Hemenway DR, Janssen-Heininger YM. Nitrogen dioxide induces death in lung epithelial cells in a density-dependent manner. Am J Respir Cell Mol Biol. 2001; 24:583–590.
44. Devalia JL, Campbell AM, Sapsford RJ, Rusznak C, Quint D, Godard P, et al. Effect of nitrogen dioxide on synthesis of inflammatory cytokines expressed by human bronchial epithelial cells in vitro. Am J Respir Cell Mol Biol. 1993; 9:271–278.
45. Wagner U, Staats P, Fehmann HC, Fischer A, Welte T, Groneberg DA. Analysis of airway secretions in a model of sulfur dioxide induced chronic obstructive pulmonary disease (COPD). J Occup Med Toxicol. 2006; 1:12.
46. Medina-Ramon M, Zanobetti A, Schwartz J. The effect of ozone and PM10 on hospital admissions for pneumonia and chronic obstructive pulmonary disease: a national multicity study. Am J Epidemiol. 2006; 163:579–588.
47. Dominici F, Peng RD, Bell ML, Pham L, McDermott A, Zeger SL, et al. Fine particulate air pollution and hospital admission for cardiovascular and respiratory diseases. JAMA. 2006; 295:1127–1134.
48. Ghozikali MG, Mosaferi M, Safari GH, Jaafari J. Effect of exposure to O3, NO2, and SO2 on chronic obstructive pulmonary disease hospitalizations in Tabriz, Iran. Environ Sci Pollut Res Int. 2015; 22:2817–2823.
49. Tseng CM, Chen YT, Ou SM, Hsiao YH, Li SY, Wang SJ, et al. The effect of cold temperature on increased exacerbation of chronic obstructive pulmonary disease: a nationwide study. PLoS One. 2013; 8:e57066.
50. Koskela HO, Koskela AK, Tukiaineu HO. Bronchoconstriction due to cold weather in COPD. The roles of direct airway effects and cutaneous reflex mechanisms. Chest. 1996; 110:632–636.
51. Takada K, Matsumoto S, Kojima E, Iwata S, Okachi S, Ninomiya K, et al. Prospective evaluation of the relationship between acute exacerbations of COPD and gastroesophageal reflux disease diagnosed by questionnaire. Respir Med. 2011; 105:1531–1536.
52. Casanova C, Baudet JS, del Valle Velasco M, Martin JM, Aguirre-Jaime A, de Torres JP, et al. Increased gastro-oesophageal reflux disease in patients with severe COPD. Eur Respir J. 2004; 23:841–845.
53. Rascon-Aguilar IE, Pamer M, Wludyka P, Cury J, Coultas D, Lambiase LR, et al. Role of gastroesophageal reflux symptoms in exacerbations of COPD. Chest. 2006; 130:1096–1101.
54. Perng DW, Chang KT, Su KC, Wu YC, Wu MT, Hsu WH, et al. Exposure of airway epithelium to bile acids associated with gastroesophageal reflux symptoms: a relation to transforming growth factor-beta1 production and fibroblast proliferation. Chest. 2007; 132:1548–1556.
55. Su KC, Wu YC, Chen CS, Hung MH, Hsiao YH, Tseng CM, et al. Bile acids increase alveolar epithelial permeability via mitogen-activated protein kinase, cytosolic phospholipase A2, cyclooxygenase-2, prostaglandin E2 and junctional proteins. Respirology. 2013; 18:848–856.
56. Perng DW, Wu YC, Tsai CC, Su KC, Liu LY, Hsu WH, et al. Bile acids induce CCN2 production through p38 MAP kinase activation in human bronchial epithelial cells: a factor contributing to airway fibrosis. Respirology. 2008; 13:983–989.
57. Wu YC, Hsu PK, Su KC, Liu LY, Tsai CC, Tsai SH, et al. Bile acid aspiration in suspected ventilator-associated pneumonia. Chest. 2009; 136:118–124.
58. Patel IS, Vlahos I, Wilkinson TM, Lloyd-Owen SJ, Donaldson GC, Wilks M, et al. Bronchiectasis, exacerbation indices, and inflammation in chronic obstructive pulmonary disease. Am J Respir Crit Care Med. 2004; 170:400–407.
59. Martinez-Garcia MA, Soler-Cataluna JJ, Donat Sanz Y, Catalan Serra P, Agramunt Lerma M, Ballestin Vicente J, et al. Factors associated with bronchiectasis in patients with COPD. Chest. 2011; 140:1130–1137.
60. Martinez-Garcia MA, de la Rosa Carrillo D, Soler-Cataluna JJ, Donat-Sanz Y, Serra PC, Lerma MA, et al. Prognostic value of bronchiectasis in patients with moderate-to-severe chronic obstructive pulmonary disease. Am J Respir Crit Care Med. 2013; 187:823–831.
61. Menezes AM, Montes de Oca M, Perez-Padilla R, Nadeau G, Wehrmeister FC, Lopez-Varela MV, et al. Increased risk of exacerbation and hospitalization in subjects with an overlap phenotype: COPD-asthma. Chest. 2014; 145:297–304.
62. Hardin M, Silverman EK, Barr RG, Hansel NN, Schroeder JD, Make BJ, et al. The clinical features of the overlap between COPD and asthma. Respir Res. 2011; 12:127.
63. Siva R, Green RH, Brightling CE, Shelley M, Hargadon B, McKenna S, et al. Eosinophilic airway inflammation and exacerbations of COPD: a randomised controlled trial. Eur Respir J. 2007; 29:906–913.
64. Perng DW, Wu CC, Su KC, Lee YC, Perng RP, Tao CW. Inhaled fluticasone and salmeterol suppress eosinophilic airway inflammation in chronic obstructive pulmonary disease: relations with lung function and bronchodilator reversibility. Lung. 2006; 184:217–222.
65. Pizzichini E, Pizzichini MM, Gibson P, Parameswaran K, Gleich GJ, Berman L, et al. Sputum eosinophilia predicts benefit from prednisone in smokers with chronic obstructive bronchitis. Am J Respir Crit Care Med. 1998; 158(5 Pt 1):1511–1517.
66. Hang LW, Hsu JY, Chang CJ, Wang HC, Cheng SL, Lin CH, et al. Predictive factors warrant screening for obstructive sleep apnea in COPD: a Taiwan National Survey. Int J Chron Obstruct Pulmon Dis. 2016; 11:665–673.
67. Soler X, Gaio E, Powell FL, Ramsdell JW, Loredo JS, Malhotra A, et al. High prevalence of obstructive sleep apnea in patients with moderate to severe chronic obstructive pulmonary disease. Ann Am Thorac Soc. 2015; 12:1219–1225.
68. Marin JM, Soriano JB, Carrizo SJ, Boldova A, Celli BR. Outcomes in patients with chronic obstructive pulmonary disease and obstructive sleep apnea: the overlap syndrome. Am J Respir Crit Care Med. 2010; 182:325–331.
69. Wang Y, Hu K, Liu K, Li Z, Yang J, Dong Y, et al. Obstructive sleep apnea exacerbates airway inflammation in patients with chronic obstructive pulmonary disease. Sleep Med. 2015; 16:1123–1130.
70. Hanania NA, Donohue JF. Pharmacologic interventions in chronic obstructive pulmonary disease: bronchodilators. Proc Am Thorac Soc. 2007; 4:526–534.
71. Montuschi P. Drugs for chronic obstructive pulmonary disease. Curr Med Chem. 2013; 20:1461–1463.
72. Bourbeau J, Christodoulopoulos P, Maltais F, Yamauchi Y, Olivenstein R, Hamid Q. Effect of salmeterol/fluticasone propionate on airway inflammation in COPD: a randomised controlled trial. Thorax. 2007; 62:938–943.
73. Barnes NC, Qiu YS, Pavord ID, Parker D, Davis PA, Zhu J, et al. Antiinflammatory effects of salmeterol/fluticasone propionate in chronic obstructive lung disease. Am J Respir Crit Care Med. 2006; 173:736–743.
74. Perng DW, Tao CW, Su KC, Tsai CC, Liu LY, Lee YC. Anti-inflammatory effects of salmeterol/fluticasone, tiotropium/fluticasone or tiotropium in COPD. Eur Respir J. 2009; 33:778–784.
75. Pera T, Zuidhof A, Valadas J, Smit M, Schoemaker RG, Gosens R, et al. Tiotropium inhibits pulmonary inflammation and remodelling in a guinea pig model of COPD. Eur Respir J. 2011; 38:789–796.
76. Wollin L, Pieper MP. Tiotropium bromide exerts anti-inflammatory activity in a cigarette smoke mouse model of COPD. Pulm Pharmacol Ther. 2010; 23:345–354.
77. Rice KL, Kunisaki KM, Niewoehner DE. Role of tiotropium in the treatment of COPD. Int J Chron Obstruct Pulmon Dis. 2007; 2:95–105.
78. Anderson R, Theron AJ, Steel HC, Durandt C, Tintinger GR, Feldman C. The beta-2-adrenoreceptor agonists, formoterol and indacaterol, but not salbutamol, effectively suppress the reactivity of human neutrophils in vitro. Mediators Inflamm. 2014; 2014:105420.
79. Decramer ML, Chapman KR, Dahl R, Frith P, Devouassoux G, Fritscher C, et al. Once-daily indacaterol versus tiotropium for patients with severe chronic obstructive pulmonary disease (INVIGORATE): a randomised, blinded, parallel-group study. Lancet Respir Med. 2013; 1:524–533.
80. Wedzicha JA, Decramer M, Ficker JH, Niewoehner DE, Sandstrom T, Taylor AF, et al. Analysis of chronic obstructive pulmonary disease exacerbations with the dual bronchodilator QVA149 compared with glycopyrronium and tiotropium (SPARK): a randomised, double-blind, parallel-group study. Lancet Respir Med. 2013; 1:199–209.
81. Miravitlles M, D'Urzo A, Singh D, Koblizek V. Pharmacological strategies to reduce exacerbation risk in COPD: a narrative review. Respir Res. 2016; 17:112.
82. Chong J, Leung B, Poole P. Phosphodiesterase 4 inhibitors for chronic obstructive pulmonary disease. Cochrane Database Syst Rev. 2013; (11):CD002309.
83. Walters JA, Tang JN, Poole P, Wood-Baker R. Pneumococcal vaccines for preventing pneumonia in chronic obstructive pulmonary disease. Cochrane Database Syst Rev. 2017; 1:CD001390.
84. Sehatzadeh S. Influenza and pneumococcal vaccinations for patients with chronic obstructive pulmonary disease (COPD): an evidence-based review. Ont Health Technol Assess Ser. 2012; 12:1–64.
85. Qian Y, Willeke K, Grinshpun SA, Donnelly J, Coffey CC. Performance of N95 respirators: filtration efficiency for airborne microbial and inert particles. Am Ind Hyg Assoc J. 1998; 59:128–132.
86. Ingebrigtsen TS, Marott JL, Vestbo J, Nordestgaard BG, Hallas J, Lange P. Gastro-esophageal reflux disease and exacerbations in chronic obstructive pulmonary disease. Respirology. 2015; 20:101–107.
87. Kiljander TO, Harding SM, Field SK, Stein MR, Nelson HS, Ekelund J, et al. Effects of esomeprazole 40 mg twice daily on asthma: a randomized placebo-controlled trial. Am J Respir Crit Care Med. 2006; 173:1091–1097.
88. Angrill J, Agusti C, de Celis R, Rano A, Gonzalez J, Sole T, et al. Bacterial colonisation in patients with bronchiectasis: microbiological pattern and risk factors. Thorax. 2002; 57:15–19.
89. Albert RK, Connett J, Bailey WC, Casaburi R, Cooper JA Jr, Criner GJ, et al. Azithromycin for prevention of exacerbations of COPD. N Engl J Med. 2011; 365:689–698.
90. Uzun S, Djamin RS, Kluytmans JA, Mulder PG, van't Veer NE, Ermens AA, et al. Azithromycin maintenance treatment in patients with frequent exacerbations of chronic obstructive pulmonary disease (COLUMBUS): a randomised, double-blind, placebo-controlled trial. Lancet Respir Med. 2014; 2:361–368.
91. Dal Negro R, Micheletto C, Tognella S, Visconti M, Turati C. Tobramycin nebulizer solution in severe COPD patients colonized with Pseudomonas aeruginosa: effects on bronchial inflammation. Adv Ther. 2008; 25:1019–1030.
92. Zanini A, Cherubino F, Zampogna E, Croce S, Pignatti P, Spanevello A. Bronchial hyperresponsiveness, airway inflammation, and reversibility in patients with chronic obstructive pulmonary disease. Int J Chron Obstruct Pulmon Dis. 2015; 10:1155–1161.
93. Jin J, Liu X, Sun Y. The prevalence of increased serum IgE and Aspergillus sensitization in patients with COPD and their association with symptoms and lung function. Respir Res. 2014; 15:130.
94. Singh B, Arora S, Khanna V. Association of severity of COPD with IgE and interleukin-1 beta. Monaldi Arch Chest Dis. 2010; 73:86–87.
95. Kobayashi S, Hanagama M, Yamanda S, Ishida M, Yanai M. Inflammatory biomarkers in asthma-COPD overlap syndrome. Int J Chron Obstruct Pulmon Dis. 2016; 11:2117–2123.
96. Negewo NA, McDonald VM, Baines KJ, Wark PA, Simpson JL, Jones PW, et al. Peripheral blood eosinophils: a surrogate marker for airway eosinophilia in stable COPD. Int J Chron Obstruct Pulmon Dis. 2016; 11:1495–1504.
97. Barnes NC, Sharma R, Lettis S, Calverley PM. Blood eosinophils as a marker of response to inhaled corticosteroids in COPD. Eur Respir J. 2016; 47:1374–1382.
98. Pavord ID, Lettis S, Locantore N, Pascoe S, Jones PW, Wedzicha JA, et al. Blood eosinophils and inhaled corticosteroid/long-acting beta-2 agonist efficacy in COPD. Thorax. 2016; 71:118–125.
99. Hernandez C, Abreu J, Abreu P, Colino R, Jimenez A. Effects of nasal positive airway pressure treatment on oxidative stress in patients with sleep apnea-hypopnea syndrome. Arch Bronconeumol. 2006; 42:125–129.
100. Pinto P, Barbara C, Montserrat JM, Patarrao RS, Guarino MP, Carmo MM, et al. Effects of CPAP on nitrate and norepinephrine levels in severe and mild-moderate sleep apnea. BMC Pulm Med. 2013; 13:13.