Abstract
Objectives
Simvastatin has been reported to attenuate the development of pulmonary hypertension through increased apoptosis as well as reduced proliferation of smooth muscle cells in obstructive vascular lesions. Microarray experiment can accomplish many genetic tests in parallel. The purpose of this study is to evaluate altered expressions of gene in rat hearts with monocrotaline (MCT)-induced pulmonary arterial hypertension after simvastatin treatment.
Methods
Six-week-old male rats were grouped as follows: control group (C group, saline injection), M group (MCT 60 mg/kg), and S group (MCT 60 mg/kg plus 10 mg/kg/day simvastatin by gavage during 28 days). Body weight, right ventricular pressure and right ventricular/left ventricle+septum ratio in each group were measured. The rats were sacrificed after 28 days. Total RNA was extracted from the rat heart tissue and microarray analysis was performed.
Results
Administration of simvastatin significantly inhibited the progression of right ventricular hypertrophy at day 28 in the S group than in the M group. Compared with the C group, MCT was associated with a significant difference in expression of genes related to biosynthesis and with the regulation of heart contraction rate. Simvastatin treatment resulted in a significantly changed expression of genes about the regulation of progression through cell cycle and system development compared to the M group. The expressions of nitric oxide synthase and brain natriuretic peptide were significantly decreased after simvastatin treatment.
Pulmonary arterial hypertension (PAH) is a disease noted for its progressive remodeling of the distal pulmonary arteries, leading to a loss of vascular cross-sectional area and an elevation in pulmonary vascular resistance (PVR) [1]. Without treatment, PAH is usually progressive, resulting in right heart failure and death [23].
PAH is characterized by a sustained reduction in vasodilator mediators such as prostaglandin I and nitric oxide (NO), and upregulation of vasoconstrictors such as endothelin (ET) [45]. Pulmonary arterial lesions in PAH consist of neointima formation and intimal fibrosis, medial hyperplasia of pulmonary artery smooth muscle cells, and adventitial fibrosis accompanied by a variable degree of perivascular inflammation [6]. Circulating cells and their mediators have also been hypothesized to be involved in disease progression because they are capable of promoting recruitment, retention and differentiation of circulating monocytic cell populations that contribute to vascular remodeling [7].
Monocrotaline (MCT) is an 11-membered macrocyclic pyrrolizidine alkaloid that leads to proliferative pulmonary vasculitis, PAH and cor-pulmonale. MCT induced PAH is commonly used as an animal model of human PAH. MCT is activated to a reactive metabolites in the liver and reactive hepatic metabolites [8]. Previous investigators have reported that when it is administered in rats it induces ET injury and causes progressive pulmonary vascular changes and right ventricular hypertrophy (RVH) [8].
Statins, which is 3-hydroxy-3-methylglutaryl coenzyme and a reductase inhibitors, have been used to treat for cardiovascular disease. Initially, the effect of statins was known to lead to lipid reduction. Statins also have varied immunomodulatory and anti-inflammatory effects on endothelial and smooth muscle cell seperating from their lipid-lowering ability [9]. Simvastatin limits RhoA/ROCK activity in the chronic hypoxia-exposed lung, thus preventing or ameliorating hemodynamic and structural markers of chronic PAH and improving long-term outcome, without causing adverse effects [10]. The beneficial effects of simvastatin on the development of PAH secondary to left ventricular dysfunction have been noted [11]. However, there has been controversial effect of simvastatin in PAH. Anand et al. [12] reported that statins were not beneficial in the treatment of PAH.
Microarray technology allows the expression and analysis of thousands of genes simultaneously [13]. Initially it was used to verify the molecular basis of cancer and the biological behavior of tumors. It is also a high throughput technique that has played a role in the understanding of the molecular basis of PAH because it can take a broad and relatively unbiased look at the gene expression changes that are presented in human and animal models of disease. Microarrays are a powerful and effective tool in the detection of genome-wide gene expression differences. They have been widely applied in the investigation of the pathobiology of many forms of PAH [14]. There is rare report about RVH associated genes in pulmonary hypertensive rat model after simvastatin treatment. The objective of this study was to investigate altered gene expressions associated RVH in MCT-induced PAH rat hearts after simvastatin.
Six-weeks-old male Sprague-Dawley rats, weighing approximately 250 g, were used for this study. All rats were housed in climate-controlled conditions with a 12-hour light:12-hour dark cycle, and had free access to chow and water. PAH was induced by the subcutaneous injection of 60 mg/kg MCT (Sigma Chemicals, St. Louis, MO, USA) dissolved in 0.5 N HCl solution. The rats were grouped as follows: control group (C group, n=12, subcutaneous injection of saline 0.1 mL/kg), MCT group (M group, n=12, subcutaneous single injection of MCT 60 mg/kg); simvastatin group (S group, n=12, subcutaneous injection of MCT 60 mg/kg plus simvastatin 10 mg/kg/day by gavage feeding). Simvastatin (Actelion Pharmaceuticals, Allschwil, Switzerland) was administrated by gavage feeding 10 mg/kg/day for 28 days. The rats were sacrificed at day 28. Heart tissues were removed and immediately frozen at −70℃ for microarray analysis, post-fixed in 10% formalin, and processed routinely for paraffin embedding. All protocols were approved by the institutional review of board (13-0226) of Ewha Womans University College of Medicine.
Body weight, right ventricle (RV), left ventricle (LV) and septum, lung and kidney were measured. RV/LV+septum weight ratio was also estimated. The catheter was placed in the RV to estimate the mean right ventricular pressure (RVP).
Total RNA was extracted from the rat heart tissue using the Tri Reagent (MRC, Cincinnati, OH, USA) according to the manufacturer's instructions. Each total RNA sample (10 µg) was labeled with cyanine conjugated dCTP (Amersharm, Piscataway, NJ, USA) by a reverse transcription reaction using reverse transcriptase, SuperScrip ll (Invitrogen, Carlsbad, CA, USA). The labeled cDNA was then concentrated using ethanol precipitation method. The concentrated cyanine labeled cDNAs were resuspended in 10 µL of hybridization solution (GenoCheck, Ansan, Korea). After labeled cDNAs were placed on Roche NimbleGen Rat genome 12-plex array (Roche NimbleGen, Madison, WI, USA) and covered by a NimbleGen H12 mixer (Roche NimbleGen), the slides were hybridized for 12 hours at 42℃ MAUI system (Biomicro Systems, Salt Lake City, UT, USA). The hybridized slides were washed in 2×sodium chloride sodium citrate (SSC), 0.1 % sodium dodecyl sulfate for 2 minutes, 1×SSC for 3 minutes, and then 0.2×SSC for 2 minutes at room temperature. The slides were centrifuged at 3,000 rpm for 20 seconds to dry.
The Roche NimbleGen Rat genome 12-plex array was submitted to Roche NimbleGen for microarray design and manufacture using maskless, digital micromirror technology. Twelve replicates of the genome were included per chip. An average of 5 different 60-base oligonucleotides (60-mer probes) represented each gene in the genome. 60-mer probes were selected such that each probe had at least three mismatches compared to all other 60-mers in the target genome. A quality control check (hybridization) was performed for each array, which contained on-chip control oligonucleotides. The arrays were analyzed using an Axon GenePix 4000B scanner with associated software (Molecular Devices, Sunnyvale, CA, USA). Gene expression levels were calculated with NimbeScan Version 2.4 (Roche NimbleGen). Relative signal intensities for each gene were generated using the robust multi-array average algorithm. The data were processed based on median polish normalization method using the NimbeScan ver. 2.4 (Roche NimbleGen). This normalization method aims to make the distribution of intensities for each array in a set of arrays the same. The normalized, and log transformed intensity values were then analyzed using GeneSpring GX 7.3.1 (Agilent Technologies, Santa Clara, CA, USA). Fold change filters included the requirement that the genes be present in at least 150% of controls for up-regulated genes and lower than 66.67% of controls for down-regulated genes. Hierarchical clustering data clustered groups that behave similarly across experiments using GeneSpring GX 7.3.1. Clustering algorithm was Euclidean distance, average linkage.
Total RNA was extracted by using trizol reagent (Invitrogen) according to the Trizol method protocol and resuspended in diethyl pyrocarbonate water. Final RNA amount was spectrophotometrically determined at 260/280 nm. Quality was assessed as the absence of smear of 18S and 28S bands analyze by means of Bio analyzer 2100 (Agilent Technologies). RNA samples were stored at −70℃ until used. cDNAs were synthesized by 1 ug of total RNA, according to the manufacture's protocol (High Capacity RNA-to-cDNA kit; Applied Biosystems, Foster City, CA, USA).
We chose several candidate genes such as ET, nitric oxide synthase (NOS), brain natriuretic peptide (BNP) and troponin I. They showed more than twice differential expression by microarray analysis.
Total body weight of rats has decreased significantly in the M group than the C group. The S group also showed significant decrease in the total body weight of rats. The RV weight has significantly increased in the M group and in the S group. The LV and septum weight has significantly decreased in the M group at day 28 and there was no significant change in the S group. The RV/LV+septum weight ratio has significantly increased in the M group at day 28. The RV/LV+septum weight ratio has significantly decreased in the S group compared with the M group (Table 1). RVP has significantly increased in the M group, but the RVP was significantly decreased in the S group compared with M group (Table 1).
The expression profile of the genes that showed differential expression by more than 1.5-fold (P<0.05) is shown. The red color indicates overexpression while green indicates underexpression (Fig. 1).
Intensity ratio histograph showed the number of genes according to the expression level changes using log intensity. X-axis indicates log 2 ratio of experimental or C group and y-axis indicates the number of genes with the corresponding log intensity. The bars on the graph except the two in the middle represent the number of genes with significant differential expression.
We compared the M group and the S group with the C group. The M group had 25 different gene expressions and the S group had 82 different gene expressions. In addition, it should be noted that the change in gene expressions between the S group and the M group was quite significant. Compared to the C group, the number of genes with significant differences of expression in the M group and S group were 25 and 282 respectively. In the S group, the expression of 150 genes showed significant differences compared with the M group (Fig. 2).
The number of genes with a significant differential expression classified according to the function of the genes is shown in Table 2. In a simple function analysis, 788 genes of M group were expressed by more than 1.5-fold for control. Of these, 384 genes related with protein biosynthesis such as mitochondrial ribosomal protein L32 (Mrpl32), ribosomal protein L12 (Rpl12), ribosomal protein L36a (Rpl36a), ribosomal protein S21(Rps21), ribosomal protein S26 (Rps26), ribosomal protein S27 (Rps27) and eukaryotic translation elongation factor 1 alpha 2 (Eef1a2) were over expressed by more than 1.5-fold. In addition, 404 genes associated with regulation of heart contraction (GO:8016), including tropomyosin 1 (Tpm1, Tma2), troponin I (Tn l), phospholemman (Plm), glucagon-like peptide 1 receptor (Glip), were down expressed by more than 1.5.
In analysis of S group compared with the C group sample, a total of 2,545 genes were differentially expressed by more than 1.5-fold. Of these, 1,253 genes including the genes associated with protein folding such as heat shock protein family H (Hsp110) member 1 (Hsp105), DnaJ heat shock protein family (Hsp40) member B4 (Dnajb4), DnaJ heat shock protein family (Hsp40) member C8 (Dnajc8), DnaJ heat shock protein family (Hsp40) member B6 (Hsj2), FK506 binding protein 1B (Fkbp1b), peptidylprolyl isomerase B (Cypb), sarcolemma associated protein (Slmap) showed over expression by more than 1.5-fold. The other 1,292 genes including the genes of detection of stimulus such as cyclic nucleotide gated channel beta 1 (Gm1959), G protein subunit alpha transducin 1 (Gnat1), G protein subunit alpha transducin 2 (Gnat2), nuclear receptor subfamily 2 group F member 6 (Nr2f6), opsin 4 (Opn4), phospholipase C beta 2 (Plcb2), toll like receptor 3 (Tlr3) and olfactory receptor 737 (Tpcr09) showed down expression by over 1.5-fold.
In analysis of the S group sample in comparison with the M group, a total of 1268 genes were differentially expressed by more than 1.5-fold in microarray. Of these, 670 genes including the genes associated with regulation of progression through cell cycle such as transforming acidic coiled-coil containing protein 3 (Tacc3), BCL2 apoptosis regulator (Bcl2), inhibin beta A subunit (Inhba), phosphatidylinositol-4,5-bisphosphate 3-kinase catalytic subunit beta (Pik3cb), S100 calcium binding protein A6 (S100a6), CDK5 regulatory subunit associated protein 1 (Cdk5rap1), protein phosphatase Mg2+/Mn2+ dependent 1G (Ppm1g), cyclin (Ccne) and cell division cycle 25C (Cdc25c) showed more than 1.5-fold over expression. The other 598 genes including the genes associated with system development such as zinc finger E-box binding homeobox 2 (Zfhx1b), ATPase plasma membrane Ca2+ transporting 2 (Atp2b2), GDNF family receptor alpha 2 (Retl2), gremlin 1 DAN family BMP antagonist (Drm), Wilms tumor 1 (Wt1), GIPC PDZ domain containing family, member 1 (Gipc), placental growth factor (Plgf), kalirin RhoGEF kinase (Duo) and GATA binding protein 3 (Gata3) showed more than 1.5-fold down expression (Table 2).
The number of genes with a significant differential expression is classified according to the function of the genes such as proliferation, translation, inflammatory response, immune response, cell adhesion, cell migration, cell differentiation, apoptosis, cell growth, transport, cell cycle, transcription, signal transduction and homeostasis. Genes associated with transcription, translation, cell differentiation and signal transduction were differentially expressed significantly (Table 3).
The results of microarray analysis of ET associated genes which showed more than 2-fold differential expression are shown in Table 4. Compared with the C group, ET-1 gene was over expressed significantly by 1.31±0.03 (P<0.05) in the M group while its expression level in the S group remained similar to that of M group. ET-2 gene was over expressed significantly by 1.30±0.02 (P<0.05) in the M group compared with the C group and down expressed significantly by 0.73±0.02 (P<0.05) in the S group compared with the M group. In addition, ET receptor (ETR)-A gene showed over expression by 1.14±0.04 in the M group compared with the C group while it showed significant down expression by 0.78±0.03 (P<0.05) in the S group compared with the M group. ETR-B was down expressed significantly by 0.67±0.02 (P<0.05) in the M group compared with the C group while ETR-B was slightly down expressed in the S group compared with the M group by 0.94±0.01 (Table 4).
The results of microarray analysis of NO associated genes are shown in Table 5. Neuronal nitric oxide synthase (NOS1) gene was significantly over expressed by 1.15±0.02 (P<0.05) in the M group compared with the C group, while its expression level decreased significantly by 0.52±0.03 (P<0.05) in the S group compared with the M group. The expression of inducible NOS (NOS2) gene stayed the same in the M group compared with the C group while it increased slightly by 1.11±0.02 (P<0.05) in the S group compared with the M group. Moreover, Endothelial nitric oxide synthase gene (NOS3) showed a minor increase in expression by 1.12±0.04 (P<0.05) in the M group compared with the C group while it showed down expression by 0.87±0.03 (P<0.05) in the S group compared with the M group (Table 5).
Microarray analysis of differential expressions of BNP and troponin I associated genes showed that BNP gene was significantly over expressed by 3.29±0.04 (P<0.05) in the M group compared with the C group while it decreased in the S group compared with the M group by 0.80±0.03 (P<0.05). In addition, the expression level of troponin I gene was significantly increased by 1.53±0.01 (P<0.05) in the M group compared with the C group while it remained similar in the S group to that of the M group (Table 6).
In our study, RVH and PAH were developed by intraperitoneal single injection of MCT. We were able to confirm this by increased RVP and RV/LV+septum ratio in MCT induced PAH rat model. We also identified the effect of simvastatin on RVH in this study. The RV weight was significantly decreased in the S group compared with the M group at day 28. The RV/LV+septum weight ratio had also significantly decreased in the S group compared with the M group.
In our microarray data on the heart tissue, genes with a significant differential expression were classified according to the function of the genes, including proliferation, translation, inflammatory response, immune response, cell adhesion, cell migration, cell differentiation, apoptosis, cell growth, transport, cell cycle, transcription, signal transduction and homeostasis. Genes associated with transcription, translation, cell differentiation, and signal transduction were significantly differentially expressed.
At first, the RV undergoes an increase in wall thickness and contractility in order to maintain a normal cardiac output and ventriculo-arterial coupling. There is a change in RV remodeling from an adaptive to a maladaptive phenotype as the disease progresses. And, as the PAH deteriorates, the RV remodeling moves from an adaptive to a maladaptive state, including RV dilatation, decreased contractility, and ventriculo-arterial uncoupling [15].
It depends on the degree of pressure overload, but RVH may progress to a condition of impaired contractile function and heart failure. Unfortunately, this is unlikely to be discerned in the early stages of ventricular remodeling. So, microarray analysis can be a valuable tool to reveal pivotal divergent mRNA expression profiles early in the development of compensated ventricular hypertrophy [15].
In our research, we selected a number of candidate genes such as ET, NOS, BNP and troponin I. They were found to have a more than 2-fold differential expression by microarray analysis. ET-2, ETR-A, NOS1, NOS3 and BNP genes were significantly decreased after simvastatin treatment in the heart tissues. Several studies have suggested that statins could prevent PAH in MCT-induced PAH rat models.
However, there has been rare reporting about RVH associated genes in pulmonary hypertensive rat model after simvastatin treatment. The purpose of this research is to elucidate the genetic pathophysiology of secondary RVH due to PAH by microarray analysis after simvastatin treatment.
There have been many investigations into the role of specific genes in the pathobiology of PAH which have focused on the balance of vasoconstriction and vasodilation [9]. It has been reported that pulmonary vascular remodeling, associated with increasing mean pulmonary arterial pressure, upregulated expressions of endothelial nitric oxide synthase (eNOS), and ET-1 results in the development of PAH [45].
Other research has been done to identify the changes of endothelium, smooth muscle cells, and the adventitia/extracellular matrix. This research showed that various genes have a role in the pathogenesis of PAH. Expression of ET-1 was found to be elevated in a number of animal models [4] and in human patients with PAH [1213]. In addition, eNOS [5], VEGF-2, PGIS, 5-lipoxigenase, and several genes are involved in the progression of PAH and have been elucidated [12].
Oxidative stress and the RhoA/Rho-kinase pathway are also thought to be involved in the pathophysiology of PAH [29]. Statins are 3-hydroxy-3-methylglutaryl-coenzyme A reductase inhibitors with pleiotropic effects [29]. Recent studies have suggested that statins may have potential use in the treatment of PAH [8916]. Statin safety and efficacy for use against PAH have been recently verified in vivo. These results seem promising due to the pleiotropic effects of statins [16]. Simvastatin attenuates neointimal thickening of the smooth muscle [10]. Simvastatin is effective in inducing apoptosis in hyperproliferative pulmonary vascular lesions and could be considered as a potential drug for treatment of human severe PAH. However, there is still controversy on the effect of simvastatin on PAH. One explanation for the negative results of atorvastatin treatment may be due to the relatively short duration of the therapy [12].
There are various microarray studies on both human and animal PAH [1315]. There have been gene studies of PAH using human samples such as lung specimen and circulating cells from PAH patients [1315]. Mitochondrial dysfunction, inflammation and mutant bone morphogenetic protein receptor 2 are associated with PAH, an incurable disease characterized by pulmonary arterial endothelial cell apoptosis, decreased microvessels and occlusive vascular remodeling [17]. Laumanns et al. [18] found only 773 differentially regulated genes, and then looked for these genes into known signaling pathways. And they focused on the planar cell polarity pathway, containing the RHO kinase. Chen et al. [2] published that his high-enrichment gene ontology analysis identified several sets of genes, and established a miRNA-mRNA network by outlining the interactions of miRNA and gene ontology-associated genes. Three downregulated miRNAs (miRNA 125-3p [miR-125-3p], miR-148-3p and miR-193) displayed the most marked regulatory function, and miR-148-3p and miR-193 were observed to have the highest number of target mRNAs. Signaling pathway analysis demonstrated 26 signal transduction pathways, with MAPK, TGF-β and cell cycle signaling as the most prominent.
Lee et al. [9] have previously published the results of lung tissues of MCT induced PAH animals. In lung tissue, the administration of simvastatin exerted weak inhibitory effects on the number of muscular pulmonary arterioles, during the development of MCT induced PAH in rats. And gene expressions of ET-1, ETR-A, NOS2, NOS3, matrix metallo proteinase and tissue inhibitor of matrix proteinase significantly decreased after simvastatin treatment. In our research, we tried to figure out altered expressions of genes in the heart. In this study, compared with the C group, MCT was associated with a significant increased expression of 384 genes of biosynthesis. And the M group presented a significant decreased expression of 404 genes regulating heart contraction rate. Simvastatin treatment resulted in a significantly increased expression of 670 genes in the regulation of the cell cycle in the S group compared with the M group. The expression of 598 genes about system development significantly decreased in the S group compared to the C group.
In addition to these results, we carried out further evaluation about specific genes associated with the progression of PAH. Of these genes, the expressions of ET-1 and ET-2 were increased in the M group compared with the C group, but there was no statistically significant difference. In addition, in the S group compared with the M group, expression of ET-2 gene was decreased. This, also, was not statistically significant. ET-1 activates both ETA and ETB-receptors, and it stimulates the production of cytokines and growth factors in response to oxidized LDL, angiotensin II [1019]. ET-1 stimulates interleukin and tumor necrosis factor-a expressions in monocytes, leukocyte adherence, platelet aggregation, and adhesion molecule expression. ET-1 stimulates the production and action of growth factors, DNA and protein synthesis, and cell cycle progression. Increased expressions of ET-1 and ET-2 in the MCT induced PAH rats supports these hypothesis.
We next examined whether the beneficial effect of simvastatin on the expressions of eNOS gene in the heart tissues. Expressions of NOS 1 and 3 were significantly decreased in the S group compared to the M group. This finding may be explained by the stabilizing of increased expressions of eNOS in MCT induced PAH.
NO, is synthesized by 3 subtypes of NO synthase (eNOS, iNOS, and nNOS) and relaxes smooth muscle, inhibits neutrophil and platelet activation and adhesion, and attenuates smooth muscle cell proliferation [20]. Some study groups have found increased NO production, enhanced NO-dependent vasodilation, and a NO-mediated attenuation of resting vascular tone in hypoxia-induced hypertensive rat lungs [2122]. Increased expression of eNOS mRNA and protein have also been observed [232425]. In other observations [5], the expressions of NOS gene in MCT induced PAH rats were increased similarly to that in hypoxia-induced hypertensive rat lungs. In our data, expressions of NOS genes were increased in MCT induced PAH rats. However, that result was not statistically significant, probably due to limited samples of heart tissue.
Simvastatin may have therapeutic potential for preventing and attenuating the development of PAH in RV dysfunction and LV dysfunction through the Rho-kinase pathway and NADPH oxidase [2]. A translational study in humans is needed to substantiate these findings.
Simvastatin attenuated the mean pulmonary artery pressure, pulmonary arteriolar remodeling [16], The main mechanisms of statins appear to be restoration of endothelial function, attenuation of pulmonary vascular remodeling, regulation of gene expression, regulation of intracellular signaling processes involved in PAH, anti-inflammatory responses, and synergy with other targeted drugs [16].
The current gold standard for diagnosing PAH and monitoring efficiency of treatment is right heart catheterization. As an alternative, serum biomarkers can also be used. Cardiac troponin T, BNP, and NT-proBNP seem to have the most potential.
BNP is synthesized by cardiac myocyte and their production is increased by factors that increase cardiac pressure and volume. Also, BNP levels correlate with mean pulmonary artery pressure, right atrial pressure, and total pulmonary resistance [26]. In our data, expressions of BNP were significantly increased in the M group compared with the C group and were decreased in the S group compared with the M group. It matched well with RV pressure and RV weight in each group. Simvastatin treatment resulted in decreased BNP expression. NT-proBNP showed a correlation with mortality and might have a prognostic value in PAH [27].
Troponin I is one of three distinct gene products and prevents contraction in the absence of Ca2+ binding to troponin C and troponin T. These troponins play an important role in regulating excitation-contraction coupling in the heart. And expressions of these gene products are precisely regulated during development and in response to myocardial injury and hemodynamic stress [28]. Troponin I was significantly increased in the M group compared with the C group. However, there was no significant difference in the expression of troponin I between the M and S group in our study.
Several genetic, molecular, biochemical, and environmental factors may explain the variable expressivity of PAH and may contribute to its aggravation or acceleration. Severe PAH seems to represent the far end of a spectrum, in which there is an augmented burden of vascular injury and dysregulated repair [29]. Our result will be helpful to understand the underlying molecular mechanisms of the failing RV in PAH.
This study is preliminary study for searching for the candidate genes associated with RVH. Limitation of this study is that sample size is small. We did not estimate westesternblot analysis. In the future, we plan to perform westernblot analysis on the protein levels of several genes associated with RVH.
In conclusion, administration of simvastatin in MCT induced PAH rats attenuated the progress of PAH. Microarray analysis showed the altered expression of various genes. This study plays a role as a screening for further investigation about the specific gene expression in PAH rat heart.
Figures and Tables
Fig. 2
Intensity ratio histograph in each group. (A) Monocrotaline vs. control group, (B) simvastatin vs. control group, and (C) simvastatin vs. monocrotaline group.
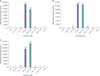
Acknowledgments
The authors gratefully acknowledge the support provided by Basic Science Research Program through the National Research Foundation of Korea funded by Ministry of Education (NRF-2013 R1A1A3004619 and NRF-2017 R1D1A1B03030831).
References
1. Humbert M, Lau EM, Montani D, Jais X, Sitbon O, Simonneau G. Advances in therapeutic interventions for patients with pulmonary arterial hypertension. Circulation. 2014; 130:2189–2208.


2. Chen IC, Tan MS, Wu BN, Chai CY, Yeh JL, Chou SH, et al. Statins ameliorate pulmonary hypertension secondary to left ventricular dysfunction through the Rho-kinase pathway and NADPH oxidase. Pediatr Pulmonol. 2017; 52:443–457.


3. Galie N, Humbert M, Vachiery JL, Gibbs S, Lang I, Torbicki A, et al. 2015 ESC/ERS Guidelines for the diagnosis and treatment of pulmonary hypertension: The Joint Task Force for the Diagnosis and Treatment of Pulmonary Hypertension of the European Society of Cardiology (ESC) and the European Respiratory Society (ERS): Endorsed by: Association for European Paediatric and Congenital Cardiology (AEPC), International Society for Heart and Lung Transplantation (ISHLT). Eur Heart J. 2016; 37:67–119.
4. Lim KA, Kim KC, Cho MS, Lee BE, Kim HS, Hong YM. Gene expression of endothelin-1 and endothelin receptor a on monocrotaline-induced pulmonary hypertension in rats after bosentan treatment. Korean Circ J. 2010; 40:459–464.


5. Koo HS, Kim KC, Hong YM. Gene expressions of nitric oxide synthase and matrix metalloproteinase-2 in monocrotaline-induced pulmonary hypertension in rats after bosentan treatment. Korean Circ J. 2011; 41:83–90.


6. Stacher E, Graham BB, Hunt JM, Gandjeva A, Groshong SD, McLaughlin VV, et al. Modern age pathology of pulmonary arterial hypertension. Am J Respir Crit Care Med. 2012; 186:261–272.


7. Foris V, Kovacs G, Tscherner M, Olschewski A, Olschewski H. Biomarkers in pulmonary hypertension: what do we know? Chest. 2013; 144:274–283.
8. Wilson DW, Segall HJ, Pan LC, Lame MW, Estep JE, Morin D. Mechanisms and pathology of monocrotaline pulmonary toxicity. Crit Rev Toxicol. 1992; 22:307–325.


9. Lee YH, Kim KC, Cho MS, Hong YM. Changes of pulmonary pathology and gene expressions after simvastatin treatment in the monocrotaline-induced pulmonary hypertension rat model. Korean Circ J. 2011; 41:518–527.


10. Wong MJ, Kantores C, Ivanovska J, Jain A, Jankov RP. Simvastatin prevents and reverses chronic pulmonary hypertension in newborn rats via pleiotropic inhibition of RhoA signaling. Am J Physiol Lung Cell Mol Physiol. 2016; 311:L985–L999.


11. Schroll S, Lange TJ, Arzt M, Sebah D, Nowrotek A, Lehmann H, et al. Effects of simvastatin on pulmonary fibrosis, pulmonary hypertension and exercise capacity in bleomycin-treated rats. Acta Physiol (Oxf). 2013; 208:191–201.


12. Anand V, Garg S, Duval S, Thenappan T. A systematic review and meta-analysis of trials using statins in pulmonary arterial hypertension. Pulm Circ. 2016; 6:295–301.


13. Menon S, Fessel J, West J. Microarray studies in pulmonary arterial hypertension. Int J Clin Pract Suppl. 2011; (169):19–28.


14. Ryan JJ, Archer SL. The right ventricle in pulmonary arterial hypertension: disorders of metabolism, angiogenesis and adrenergic signaling in right ventricular failure. Circ Res. 2014; 115:176–188.
15. Buermans HP, Redout EM, Schiel AE, Musters RJ, Zuidwijk M, Eijk PP, et al. Microarray analysis reveals pivotal divergent mRNA expression profiles early in the development of either compensated ventricular hypertrophy or heart failure. Physiol Genomics. 2005; 21:314–323.


16. Liu WH, Xu XH, Luo Q, Zhang HL, Wang Y, Xi QY, et al. Inhibition of the RhoA/Rho-associated, coiled-coil-containing protein kinase-1 pathway is involved in the therapeutic effects of simvastatin on pulmonary arterial hypertension. Clin Exp Hypertens. 2018; 40:224–230.


17. Diebold I, Hennigs JK, Miyagawa K, Li CG, Nickel NP, Kaschwich M, et al. BMPR2 preserves mitochondrial function and DNA during reoxygenation to promote endothelial cell survival and reverse pulmonary hypertension. Cell Metab. 2015; 21:596–608.


18. Laumanns IP, Fink L, Wilhelm J, Wolff JC, Mitnacht-Kraus R, Graef-Hoechst S, et al. The noncanonical WNT pathway is operative in idiopathic pulmonary arterial hypertension. Am J Respir Cell Mol Biol. 2009; 40:683–691.


19. Varshney R, Ali Q, Wu C, Sun Z. Monocrotaline-induced pulmonary hypertension involves downregulation of antiaging protein klotho and eNOS activity. Hypertension. 2016; 68:1255–1263.


20. Murata T, Kinoshita K, Hori M, Kuwahara M, Tsubone H, Karaki H, et al. Statin protects endothelial nitric oxide synthase activity in hypoxia-induced pulmonary hypertension. Arterioscler Thromb Vasc Biol. 2005; 25:2335–2342.


21. Jaitovich A, Jourd'heuil D. A Brief overview of nitric oxide and reactive oxygen species signaling in hypoxia-induced pulmonary hypertension. Adv Exp Med Biol. 2017; 967:71–81.


22. Klinger JR, Kadowitz PJ. The nitric oxide pathway in pulmonary vascular disease. Am J Cardiol. 2017; 120(8S):S71–S79.


23. Isaacson TC, Hampl V, Weir EK, Nelson DP, Archer SL. Increased endothelium-derived NO in hypertensive pulmonary circulation of chronically hypoxic rats. J Appl Physiol (1985). 1994; 76:933–940.


24. Tyler RC, Muramatsu M, Abman SH, Stelzner TJ, Rodman DM, Bloch KD, et al. Variable expression of endothelial NO synthase in three forms of rat pulmonary hypertension. Am J Physiol. 1999; 276(2 Pt 1):L297–L303.
25. Dupont LL, Glynos C, Bracke KR, Brouckaert P, Brusselle GG. Role of the nitric oxide-soluble guanylyl cyclase pathway in obstructive airway diseases. Pulm Pharmacol Ther. 2014; 29:1–6.


26. Nagaya N, Nishikimi T, Okano Y, Uematsu M, Satoh T, Kyotani S, et al. Plasma brain natriuretic peptide levels increase in proportion to the extent of right ventricular dysfunction in pulmonary hypertension. J Am Coll Cardiol. 1998; 31:202–208.


27. Ten Kate CA, Tibboel D, Kraemer US. B-type natriuretic peptide as a parameter for pulmonary hypertension in children: a systematic review. Eur J Pediatr. 2015; 174:1267–1275.


28. Lammers AE, Apitz C, Zartner P, Hager A, Dubowy KO, Hansmann G. Diagnostics, monitoring and outpatient care in children with suspected pulmonary hypertension/paediatric pulmonary hypertensive vascular disease. Expert consensus statement on the diagnosis and treatment of paediatric pulmonary hypertension. The European Paediatric Pulmonary Vascular Disease Network, endorsed by ISHLT and DGPK. Heart. 2016; 102:Suppl 2. ii1–ii13.


29. Vonk-Noordegraaf A, Haddad F, Chin KM, Forfia PR, Kawut SM, Lumens J, et al. Right heart adaptation to pulmonary arterial hypertension: physiology and pathobiology. J Am Coll Cardiol. 2013; 62:25 Suppl. D22–D33.