Abstract
Purpose
To determine the occurrence of COX-2 methylation in gastric carcinoma (GC), the status and level of CpG methylation in the promoter region of cyclooxygenase-2 (COX-2) were analyzed in early and advanced GCs, as well as in normal gastric tissues.
Methods
The extent of promoter methylation of the COX-2 gene was assessed quantitatively using pyrosequencing in 60 early and 60 advanced GCs samples harvested upon gastrectomy, and 40 normal gastric mucosa samples from patients with benign gastric diseases as controls.
Results
The methylation frequency for the COX-2 gene was significantly higher in early than in advanced GCs (40.0% vs. 20.0%, P < 0.05). A significant difference was found in COX-2 methylation between GCs and normal gastric tissues (30.0% vs. 10.0%, by PS; P < 0.05). COX-2 gene methylation was significantly associated with the depth of invasion (P = 0.003), lymph node metastasis (P = 0.009), distant metastasis (P = 0.036), and TNM staging (P = 0.007). The overall survival of patients with COX-2 methylation was significantly lower than that of patients without COX-2 methylation (P = 0.005).
Conclusion
These results demonstrated that COX-2 promoter methylation was significantly higher in tumor tissues, and was an early event for GC, thus, COX-2 gene methylation may be important in the initial development of gastric carcinogenesis. Thus, GCs with methylation in COX-2 may not be good candidates for treatment with COX-2 inhibitors. Furthermore, COX-2 methylation could be a significant prognostic factor predicting a favorable effect on GC patient outcome when downregulated.
Epigenetic silencing of tumor-related genes due to CpG island methylation has been reported recently to be another genetic alteration in gastric carcinoma (GC) [1]. CpG islands are 0.5- to 2-kb regions rich in cytosine-guanine dinucleotides and are present in the 5′ promoter region of approximately 40%–50% of human genes [2]. DNA methylation plays an important role in transcriptional repression of imprinted genes and genes on inactivated X chromosomes, maintaining the integrity of chromosomes or defense against highly repeated mobile elements [34]. Aberrant methylation of CpG islands, which are normally protected from DNA methylation, is associated with DNA structural change and consequent gene inactivation. Aberrant methylation of promoter CpG islands is now recognized as an important mechanism for gene inactivation as an alternative to gene mutation or deletion in tumorigenesis [5].
GC has been shown to have a high frequency of DNA hypermethylation [6]. Genes that are inactivated by CpG island hypermethylation have been reported in GC and involve tumor suppressor, cell-cycle regulator, tissue invasion-related, and DNA mismatch repair genes [7].
Cyclooxygenase-2 (COX-2) is important in the carcinogenesis of gastrointestinal cancers [8]. COX, the rate-limiting enzyme in the conversion of arachidonic acid to prostaglandin H2, is the main target of nonsteroidal anti-inflammatory drugs [9]. Two isoforms of COX share over 60% identity at the amino acid level [10]. COX-1 is constitutively expressed in most tissues and is associated with a housekeeping gene for cytoprotection of the stomach mucosa, vasodilation in the kidney, and control of platelet aggregation [11]. In contrast, COX-2 is undetectable in most normal tissues; it can be induced early by cytokines, growth factors, hormones, and tumor promoters and is involved in inflammation [12].
Several studies have suggested a crucial role of COX-2 in the pathophysiology of inflammation and carcinogenesis [13]. Understanding the role of COX-2 in carcinogenesis is thus particularly important because of the therapeutic implications. Overexpression of COX-2 promotes cell growth, inhibits apoptosis, and increases cell motility and adhesion in intestinal epithelial cells [14]. Thus, increased expression of COX-2 may contribute to carcinogenesis and metastasis. Moreover, the detection of COX-2 overexpression in colorectal and GCs may have prognostic significance [15].
Techniques for assessing promoter methylation status vary considerably. Several methods that assess this feature have been reported, but the most widely used is the methylation-specific polymerase chain reaction (MSP) analysis of DNA after bisulfite treatment [16]. However, the popularity of the MSP method is tempered by notable shortcomings, including its qualitative nature, the limited CpG sites targeted, the lack of internal control, and false-positive signals at high PCR cycles. Recent attempts to remedy some of these deficiencies have led to the development of an alternative high-throughput technique for methylation analysis, known as pyrosequencing (PS) [17]. PS, a sensitive real-time sequencing-by-synthesis method, is based on the conversion of DNA by pyrophosphate enzymatic treatment and stepwise luminescence incorporation of nucleotides for quantitative measurement of CpG island methylation.
Whether COX-2 gene expression is regulated by promoter methylation in GC tissues as well as in corresponding normal tissue remains controversial. Several reports have suggested that COX-2 expression is silenced by aberrant methylation of the COX-2 promoter region in colorectal and GCs [1819]. The aim of the present study was to evaluate the occurrence of CpG island methylation in GC in relation to COX-2 expression. Using quantitative PS and qualitative MSP techniques, promoter CpG methylation of the COX-2 gene was investigated in GCs and normal gastric mucosa in association with its gene product expression by immunohistochemistry. Subsequently, the relationships between methylation of COX-2 and clinicopathological features, including Helicobacter pylori infection and patients' survival, were analyzed.
GC specimens were obtained from 120 patients who had undergone surgical resection at Chonnam National University Hospital between January and December 2005. These cases were retrospectively identified from surgical pathology files at the hospital. The panel of tumor specimens consisted of 60 early gastric carcinoma (EGC) and 60 advanced gastric carcinoma (AGC) samples. A control group of 40 patients with benign gastric pathology was also selected. To exclude the possibility of cancer field defects, cases of normal gastric mucosa from a separate group of patients with benign gastric pathology were also selected. Diagnoses of the control group were as follows: 13 gastric peptic ulcers, 8 ectopic pancreases, 7 leiomyomas, 2 schwannomas, 9 hyperplastic polyps, and 1 inflammatory fibroid polyp. No control patient had a clinical history of gastric dysplasia or GC. Clinicopathologic data were available for the 120 patients with GC. The mean age of the patients was 63.5 ± 10.8 years (range, 30–85 years) and 71 men and 49 women were enrolled. The mean size of tumors, determined by horizontal diameter, was 3.6 ± 2.3 cm. Tumors were divided into 2 histological subgroups: the low-grade group, consisting of papillary and tubular adenocarcinomas of well or moderate differentiation; and the high-grade group, consisting of poorly differentiated adenocarcinomas, signet ring cell carcinomas, and mucinous adenocarcinomas. Sixty of 120 tumors were high grade and 78/120 tumors were of intestinal type according to the Lauren classification. Tumor extent was in accordance with the criteria of the 7th edition of the American Joint Committee on Cancer staging system [20]. Tumor metastasis to the lymph nodes and distant metastasis to other organs were observed in 53 and 14 of 120 cases, respectively. The present study was approved by the Institutional Review Board of Chonnam National University Hospital (IRB No. CNUHH-2013-078).
Tissues were carefully dissected from the carcinoma on hematoxylin and eosin-stained slides as described previously [21]. All samples of normal gastric mucosa were derived from the control specimens. Genomic DNA was extracted from microdissected tissue using a standard protocol. Briefly, microdissected tissue was treated with 50-µL buffer containing 0.5% Tween 20 (Boehringer Mannheim Biochemicals, Mannheim, Germany).
The methylation status of COX-2 exon 1 was determined by bisulfite treatment of DNA followed by MSP as described previously, with modification [22]. The following 2 MSP primer sets were designed using Methprimer (http://www.urogene. org/methprimer/index1.html) (Table 1). Bisulfite treatment was performed as follows. Briefly, 2-µg microdissected genomic DNA was denatured with NaOH, followed by incubation with sodium bisulfite. After treatment, DNA was purified using the DNA Clean-Up kit (Promega, Madison, WI, USA) as recommended by the manufacturer, treated with NaOH, precipitated with ethanol, resuspended in water. Amplification was performed in a GeneAmp PCR System 9700 thermocycler (Perkin Elmer, Waltham, MA, USA). A PCR product was visualized by ethidium bromide staining. Genomic DNA was treated with Sss1 methylase (New England Biolabs, Ipswich, MA, USA) according to the manufacturer's protocol and subjected to bisulfite modification, then used as a positive control for methylated DNA. A sample was determined to be positive for methylation if a band was observed in the DNA amplified by the methylated reaction primers.
PCR products from the bisulfate-treated DNA were analyzed to determine the methylated fraction percentage of eight CpG sites within the COX-2 promoter region by PS. Bisulfite-modified DNA (30–50 ng) was amplified by PCR in a 50-µL reaction forward primer (5′-GGAGATTAGTTTAGAATTGGTTTT-3′), and biotinylated reverse primer (5′-biotin-AATCCCCACTCTCCTATCTAATCC-3′). All amplification reactions were performed as follows: 95℃ for 15 minutes, 35 cycles of 95℃ for 30 seconds, 54℃ for 40 seconds, and 72℃ for 1 minute, followed by 72℃ for 10 minutes. The methylation status determined by the PS assay was analyzed as both a continuous variable (methylation level) and a categorical variable (methylation negative, methylation level < 30%; methylation positive, methylation level ≥ 30%). The empiric cutoff value of ≥30% was selected by the limit of the unmethylated control. The technical controls for PS revealed median methylations of “N/A” (not available; unmethylated control) and 92.6% (methylated control) in the eight sequential CpG sites, thereby defining the detection limits of this assay.
Immunohistochemical staining for COX-2 protein was performed on paraffin-embedded tissue sections. Avidin-biotin peroxidase complex staining using diaminobenzidine (Sigma-Aldrich, St. Louis, MO, USA) as the chromogen was performed using the streptavidin-horseradish peroxidase detection system (Ventana, Biotek Solutions, Tucson, AZ, USA). The primary antibody (1:100) used for the immunohistochemical analysis was a monoclonal antibody against COX-2 (SP21, EPITOMICS, Burlingame, CA, USA). The specificity of this antibody had been previously confirmed by the manufacturer. Immunohistochemical staining was performed twice for samples yielding inconsistent results. The intensity, area, and pattern of immunostaining were evaluated independently by 2 pathologists with no knowledge of the clinical data. The staining intensity was graded on a 4-point scale: 0, no staining of cancer cells; 1, weak staining; 2, moderate staining; and 3, strong staining. The percentage of the staining area was also graded on a four-point scale: 0, none; 1, <10%; 2, 10%–50%; and 3, >50%. An overall score was calculated as the product of the staining intensity and area. Theoretically, overall scores could range from 0 to 9. Specimens with a score > 4 were considered to show positive expression, and those with a score ≤ 4 were considered to show negative expression. Staining of tumor cytoplasm was evaluated in coded slides without knowledge of the molecular analysis results.
All statistical analyses were conducted using the SPSS ver. 15.0 (SPSS Inc., Chicago, IL, USA). The methylation status of the COX-2 gene along the progression of GC and the association of promoter gene methylation status with clinicopathologic variables were analyzed using Pearson chi-square test or 2-tailed Fisher exact probability test, when appropriate. Agreement between the methylation status and immunohistochemical expression was estimated by calculating the kappa value. Comparison of Kaplan-Meier product limit survival curves was performed using the log-rank test. All reported P-values were 2-sided, and the level of significance was set at P < 0.05.
Of 120 primary GCs, 19 (15.8%) exhibited methylation in a CpG island of the COX-2 gene, and 101 (84.2%) showed no evidence of promoter methylation (Fig. 1). Of 60 EGCs and 60 AGCs, 9 (15.0%) and 10 (16.7%) exhibited COX-2 promoter methylation, respectively (Table 2). In the 40 normal tissues, 8 (20.0%) showed methylation of the gene. A slight decreasing tendency for COX-2 promoter methylation was observed from normal gastric mucosa to more invasive carcinomas. The difference in the frequency of COX-2 promoter methylation between normal mucosa and GC was statistically insignificant (20.0 vs. 15.8%, P = 0.542).
Methylation levels were quantified by bisulfate PS. PS analysis of the GC samples revealed low levels of COX-2 methylation, with wide variation among the methylated tumors. The overall mean methylation densities of the eight CpG sites in the COX-2 promoter in the 40 normal gastric mucosa, 60 EGC, and 60 AGC samples were 19.9%, 33.5%, and 18.5%, respectively (Table 3). Interestingly, the methylation level varied only to a small extent among tumors and normal mucosa, suggesting that the epigenetic pattern of tumor tissues may represent some sort of concordance of the methylation pattern observed in normal mucosa. The percentage of methylation among the eight sites showed similar patterns in all groups of normal and tumor tissues.
PS analysis revealed a relatively high frequency of COX-2 methylation in 30.0% (36 of 120) of the GC samples by applying an empirical cutoff value of ≥30%, whereas COX-2 was infrequently methylated in normal gastric mucosa (10.0%, 4 of 40; Table 2). Additionally, the frequency difference was statistically significant (P < 0.05). In the 120 GC samples analyzed, the COX-2 methylation frequency by the 2 combined techniques was 45.8% (55 of 120 GC cases: 55.5% EGC, 36.7% AGC). Of the 40 normal tissues, the methylation rate using both assays was 30% (12 of 40 cases). Thus, PS may be a more sensitive and specific tool than MSP for assessing methylation status.
To examine whether aberrant methylation of COX-2 correlated with loss of expression in GC, immunohistochemistry was performed. COX-2 protein was not overexpressed in the normal gastric mucosa. Of 120 GCs, positive expression was observed in 75.0% (90 of 120: 80% EGC, 70% AGC) of GC cases. Seventeen cases were unmethylated in the COX-2 gene and 13 cases showed methylation that did not express the COX-2 protein. COX-2 promoter methylation determined by combining the MSP and PS techniques was not significantly associated with a lack of protein expression, based on immunohistochemical staining (P = 0.371). As shown in Table 4, PS revealed COX-2 promoter methylation in 10 of 30 (33.3%) GCs with negative COX-2 expression (41.7% of EGCs, 27.8% of AGCs) and in 26 of 90 (28.9%) GCs with positive COX-2 expression. A comparison between COX-2 expression and COX-2 promoter methylation indicated that COX-2 methylation was not significantly correlated with negative COX-2 expression in GCs (P = 0.403).
H. pylori positivity (n = 41) and negativity (n = 79) was observed among patients with GC. Promoter methylation of the COX-2 gene was not correlated with H. pylori infection (P = 0.571). However, COX-2 promoter methylation determined by combining MSP and PS was closely associated with depth of invasion (P = 0.003), lymph node metastasis (P = 0.009), distant metastasis (P = 0.036), and tumor node metastasis staging (P = 0.007) (Table 5). In both EGCs and AGCs, immunohistochemical overexpression of COX-2 was significantly more common in intestinal-type than in diffuse-type tumors (P = 0.031). Survival analysis was performed by log-rank analysis of Kaplan-Meier survival curves (Figs. 2, 3). In the present study, the median follow-up duration after surgery was 67.8 months (range, 0.9–77.1 months).The overall survival for patients with COX-2 methylation was significantly lower than that for patients without COX-2 methylation (P = 0.005).
Increased COX-2 methylation has been shown to be a tumor-related event in GC [1823]. In the present study, relatively low levels (10%) of COX-2 methylation were detected in normal gastric tissues compared with those in GCs using the PS technique. COX-2 methylation was detected using PA assay in 30% of GCs using the predetermined cutoff level. According to data obtained from other published reports, a wide range (12%–63.5%) of COX-2 methylation in GC was observed, depending on the method of methylation analysis and primer design used [181923]. The frequency of COX-2 methylation in GC was 15%–40% in the present study, which was similar to other data from Korean populations [23]. Although subtle methodological differences could exist among these studies, the methylation rate was relatively higher, even in normal tissues from patients without cancer (20.0% by MSP), in the current study.
PS technology provides a quantitative measurement of the DNA methylation level [17]. The concept of a DNA methylation level can be confusing, because it has been defined both as the level of methylated DNA in a genome and as the number of CpG loci methylated in a specific DNA gene sequence. In the present study, which may be the first to document quantitative analysis of COX-2 in GC, the DNA methylation level has been considered to be the C/T ratio for a given gene: i.e., the number of methylated sites in a DNA gene sequence. The DNA mixture from different types of cells, such as stromal and inflammatory cells, can mask the true level of DNA methylation at a specific CpG site in a specific cell type. This is particularly important for quantitative DNA methylation studies. The potential for detecting multiple CpG loci with PS allows the levels of DNA methylation to be compared among multiple CpG loci. Thus, the present study found similar DNA methylation levels at the eight CpG loci for the COX-2 promoter in the methylated tumor DNA samples. This finding of similar methylation topography in tumors suggests that the epigenetic topography at this locus is largely stable. The structure in a particular region, chromatin configuration, or DNA-binding protein interactions may be responsible for the apparent stability of the pattern across patients with GC. However, PS has several disadvantages [17]. Above all, the reading length in a single reaction is currently limited to <100 bp and 20 CpG loci. Owing to difficulties in primer design, PS cannot always be used to detect certain regions in a CpG island.
H. pylori is believed to be the major contributing factor to the development of chronic gastritis and peptic ulcer diseases in humans, and epidemiological and interventional studies in humans and experimental animals strongly suggest that H. pylori infection increases the risk of adenocarcinoma in the distal stomach [24]. Up-regulation of COX-2 plays a critical role in the inflammatory changes and tissue damage associated with chronic H. pylori infection, and is also involved in gastric tumorigenesis [25]. The expression of COX-2 protein is significantly higher in patients with GC than in those with normal gastric mucosa. Thus, COX-2 expression is assumed to play a key role in H. pylori–associated GC in addition to the propagation of gastric inflammation. Based on the strict criteria used in the present study, positive expression of COX-2 in GCs was shown in 75% (90 of 120) of samples. Among 120 GC cases tested for H. pylori, 41 (34.2%) were H. pylori–positive, but 32 (78%) showed COX-2 expression. In contrast to previous observations, the current study showed that COX-2 expression did not correlate with H. pylori infection. Despite the apparent selective advantage given by COX-2 overexpression, results from the present study and others suggest that COX-2 expression may not be essential in all cases of gastric carcinogenesis [26]. Recent studies have documented that H. pylori–induced inflammation is associated with COX-2 expression [25]. However, no significant correlation between H. pylori and COX-2 methylation status was found in the present study. Virulence has been reported to vary among different strains and types of H. pylori infection, sometimes showing geographic patterns [27]. The expression/activation of reliable H. pylori effector genes in gastric tissues in the presence and absence of H. pylori infection should be investigated to exclude possible decreased virulence of H. pylori strains infecting GCs.
Expression of COX-2 is regulated by positive and negative regulatory factors. For example, overexpression of COX-2 has been shown to contribute to tumorigenicity of ras-transformed intestinal epithelial cells. Oncogenes such as Ras and c-Myc have also been demonstrated to positively regulate COX-2 [28]. By contrast, tumor suppressor genes such as p53 negatively regulate COX-2. In addition to transcriptional regulation of the gene, several experimental results have suggested that epigenetic changes such as DNA methylation affect gene expression by modifying the chromatin structure [29]. Here, methylation status of COX-2 tends to be associated with negative expression of COX-2. In GC cells that do not express COX-2, a high methylation level of the gene was detected, indicating that methylation plays a role in regulation of the gene. An inverse correlation between DNA methylation and immunohistochemical expression of COX-2 in GC has been well documented [18]. Transcriptional silencing of COX-2 due to promoter methylation was also found in 43.1% of patients with GC [19]. Although immunohistochemistry revealed that 25% (30 of 120) of GCs showed negative expression of COX-2, 13 tumors with COX-2 methylation maintained COX-2 expression and 17 tumors lacking COX-2 methylation showed negative COX-2 expression by combined MSP and PS analysis. The genotype-phenotype discordance in 42.5% (51 of 120) of the cases may be explained at the molecular level. The observed variation in COX-2 expression may indicate that it is controlled by other molecular pathways in a cell type-specific manner. As such, DNA methylation in the promoter region, but not in the coding region, has a substantial effect on gene transcription.
The present study provides a more comprehensive picture of the distribution of DNA methylation throughout the promoter region of COX-2 in GC. CpG methylation in COX-2 may prove to be a useful molecular biomarker for assessing the risk for GC development, and drug therapies targeting COX-2 expression may improve the prognosis in patients with GC. Considering the important role of methylation in carcinogenesis, immeasurable benefits may be gained by adopting a more quantitative and standardized analytical method, such as PS.
The results of the present study demonstrated that PS is more sensitive than MSP for determining methylation in epigenetic research, and that combined PS and MSP results correlate significantly with clinicopathological factors, such as depth of invasion, lymph node metastasis, and distant metastasis. The current study also clearly showed that COX-2 methylation status revealed significant differences in patients' overall survival by univariate analysis of Kaplan-Meier survival curves. The clinical applicability of the present study is that the GC characteristic of COX-2 methylation status can be assessed in routine diagnostic biopsy specimens and used as a valuable preoperative biomarker to tailor treatment modalities at the time of surgery to patients with GC. To date, no such preoperative biomarker is available in GC.
In conclusion, aberrant methylation of COX-2 may play a role in the regulation of gene expression. Furthermore, COX-2 methylation could be a significant prognostic factor predicting a favorable effect on outcomes in patients with GC when downregulated.
Figures and Tables
Fig. 1
Representative results from methylation-specific PCR of COX-2 promoter methylation in normal gastric tissues and gastric carcinomas using 2 primer sets (A and B). EGC, early gastric carcinoma; AGC, advanced gastric carcinoma; SM, size marker; M, methylated; U, unmethylated.
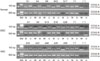
Fig. 2
Survival curve for patients with gastric carcinoma, according to COX-2 expression by immunohistochemistry (P = 0.383).
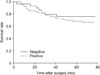
Fig. 3
Survival curve for patients with gastric carcinoma, according to methylation of COX-2 as determined by combined methylation-specific PCR and pyrosequencing (P = 0.005). M, methylated; UM, unmethylated case.
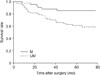
Table 1
Primer sequences and PCR conditions for methylation-specific PCR and pyrosequencing analysis

Table 2
Proportion of COX-2 methylation in normal gastric tissues and carcinomas by methylation-specific PCR and pyrosequencing

Table 3
Pyrosequencing analysis shows a mean methylation level for each CpG across COX-2 promoter regions in gastric carcinoma and normal tissue

Table 4
Correlation between immunohistochemical expression of COX-2 protein and COX-2 methylation in gastric carcinomas
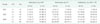
MSP, methylation-specific polymerase chain reaction; PS, pyrosequencing; M, methylated case; U, unmethylated case; EGC, early gastric carcinoma; AGC, advanced gastric carcinoma; (−), negative expression of COX-2 protein; (+), positive expression of COX-2 protein; IHC, immunohistochemistry.
a)P = 0.054. b)P = 0.403. c)P = 0.371.
References
1. Toyota M, Ahuja N, Suzuki H, Itoh F, Ohe-Toyota M, Imai K, et al. Aberrant methylation in gastric cancer associated with the CpG island methylator phenotype. Cancer Res. 1999; 59:5438–5442.
4. O'Neill RJ, O'Neill MJ, Graves JA. Undermethylation associated with retroelement activation and chromosome remodelling in an interspecific mammalian hybrid. Nature. 1998; 393:68–72.
5. Baylin SB, Herman JG, Graff JR, Vertino PM, Issa JP. Alterations in DNA methylation: a fundamental aspect of neoplasia. Adv Cancer Res. 1998; 72:141–196.
6. Esteller M. CpG island hypermethylation and tumor suppressor genes: a booming present, a brighter future. Oncogene. 2002; 21:5427–5440.


7. Kang GH, Lee S, Kim WH, Lee HW, Kim JC, Rhyu MG, et al. Epstein-barr virus-positive gastric carcinoma demonstrates frequent aberrant methylation of multiple genes and constitutes CpG island methylator phenotype-positive gastric carcinoma. Am J Pathol. 2002; 160:787–794.


8. Zimmermann KC, Sarbia M, Weber AA, Borchard F, Gabbert HE, Schror K. Cyclooxygenase-2 expression in human esophageal carcinoma. Cancer Res. 1999; 59:198–204.
9. DeWitt DL. Prostaglandin endoperoxide synthase: regulation of enzyme expression. Biochim Biophys Acta. 1991; 1083:121–134.


10. Loll PJ, Gravito RM. The isoforms of cyclooxygenase: structure and function. Expert Opin Investig Drugs. 1994; 3:1171–1180.
12. Inoue H, Tanabe T. Transcriptional role of the nuclear factor kappa B site in the induction by lipopolysaccharide and suppression by dexamethasone of cyclooxygenase-2 in U937 cells. Biochem Biophys Res Commun. 1998; 244:143–148.
13. Prescott SM, Fitzpatrick FA. Cyclooxygenase-2 and carcinogenesis. Biochim Biophys Acta. 2000; 1470:M69–M78.


14. Tsujii M, DuBois RN. Alterations in cellular adhesion and apoptosis in epithelial cells overexpressing prostaglandin endoperoxide synthase 2. Cell. 1995; 83:493–501.


15. Oshima M, Dinchuk JE, Kargman SL, Oshima H, Hancock B, Kwong E, et al. Suppression of intestinal polyposis in Apc delta716 knockout mice by inhibition of cyclooxygenase 2 (COX-2). Cell. 1996; 87:803–809.
16. Herman JG, Graff JR, Myohanen S, Nelkin BD, Baylin SB. Methylation-specific PCR: a novel PCR assay for methylation status of CpG islands. Proc Natl Acad Sci U S A. 1996; 93:9821–9826.


17. Colella S, Shen L, Baggerly KA, Issa JP, Krahe R. Sensitive and quantitative universal Pyrosequencing methylation analysis of CpG sites. Biotechniques. 2003; 35:146–150.


18. Kikuchi T, Itoh F, Toyota M, Suzuki H, Yamamoto H, Fujita M, et al. Aberrant methylation and histone deacetylation of cyclooxygenase 2 in gastric cancer. Int J Cancer. 2002; 97:272–277.


19. Yu J, Leung WK, Lee TL, Tse PC, To KF, Sung JJ. Promoter hypermethylation of cyclooxygenase-2 in gastric carcinoma. Int J Oncol. 2003; 22:1025–1031.


20. Edge SB, Byrd DR, Compton CC, Fritz AG, Greene FL, Trotti A. AJCC cancer staging manual. 7th ed. New York: Springer-Verlag;2010.
21. Goelz SE, Hamilton SR, Vogelstein B. Purification of DNA from formaldehyde fixed and paraffin embedded human tissue. Biochem Biophys Res Commun. 1985; 130:118–126.


22. Ogino S, Odze RD, Kawasaki T, Brahmandam M, Kirkner GJ, Laird PW, et al. Correlation of pathologic features with CpG island methylator phenotype (CIMP) by quantitative DNA methylation analysis in colorectal carcinoma. Am J Surg Pathol. 2006; 30:1175–1183.


23. Kang GH, Lee S, Kim JS, Jung HY. Profile of aberrant CpG island methylation along the multistep pathway of gastric carcinogenesis. Lab Invest. 2003; 83:635–641.


24. Huang JQ, Sridhar S, Chen Y, Hunt RH. Meta-analysis of the relationship between Helicobacter pylori seropositivity and gastric cancer. Gastroenterology. 1998; 114:1169–1179.


25. Uemura N, Okamoto S, Yamamoto S, Matsumura N, Yamaguchi S, Yamakido M, et al. Helicobacter pylori infection and the development of gastric cancer. N Engl J Med. 2001; 345:784–789.
26. Wambura C, Aoyama N, Shirasaka D, Sakai T, Ikemura T, Sakashita M, et al. Effect of Helicobacter pylori-induced cyclooxygenase-2 on gastric epithelial cell kinetics: implication for gastric carcinogenesis. Helicobacter. 2002; 7:129–138.


27. Romano M, Ricci V, Memoli A, Tuccillo C, Di Popolo A, Sommi P, et al. Helicobacter pylori up-regulates cyclooxygenase-2 mRNA expression and prostaglandin E2 synthesis in MKN 28 gastric mucosal cells in vitro. J Biol Chem. 1998; 273:28560–28563.

