Abstract
Radiotherapy for the treatment of cancer in companion animals is currently administered by using megavoltage X-ray machines. Because these machines are expensive, most animal hospitals do not perform radiotherapy. This study evaluated the ability of relatively inexpensive kilovoltage X-ray machines to treat companion animals. A simulation study based on a commercial treatment-planning system was performed for tumors of the brain (non-infectious meningoencephalitis), nasal cavity (malignant nasal tumors), forefoot (malignant muscular tumors), and abdomen (malignant intestinal tumors). The results of kilovoltage (300 kV and 450 kV) and megavoltage (6 MV) X-ray beams were compared. Whereas the 300 kV and 6 MV X-ray beams provided optimal radiation dose homogeneity and conformity, respectively, for brain tumors, the 6 MV X-rays provided optimal homogeneity and radiation conformity for nasal cavity, forefoot, and abdominal tumors. Although megavoltage X-ray beams provided better radiation dose distribution in most treated animals, the differences between megavoltage and kilovoltage X-ray beams were relatively small. The similar therapeutic effects of the kilovoltage and 6 MV X-ray beams suggest that kilovoltage X-ray beams may be effective alternatives to megavoltage X-ray beams in treating cancers in companion animals.
Radiotherapy is important in cancer treatment [6], including the treatment of cancer in companion animals. For example, radiotherapy has prolonged survival in dogs with nasal adenocarcinomas, when compared with results from surgery or chemotherapy [12]. Moreover, radiotherapy, both alone and in combination with surgery, has produced good therapeutic outcomes in animals with intracranial meningiomas [2], and radiotherapy has provided excellent therapeutic efficacy for animals with difficult-to-treat mandibular squamous cell carcinomas and genitourinary carcinomas [34711132326].
Although the incidence of cancer is generally higher in companion animals than in humans [892527], methods of treating cancers in companion animals are limited [20]. Radiotherapy of companion animals usually involves the use of a linear accelerator designed to treat humans. Clinical linear accelerators accelerate electrons by using high-frequency electromagnetic waves, producing high-energy X-ray beams when the electrons hit their target. Although these X-rays are effective in treating deeply located tumors, the use of these devices to treat cancers in companion animals is limited by their large size, high cost, and complicated protocols. Moreover, these devices require shielding to protect people in the vicinity against high-energy radiation. At present, few animal hospitals in the USA, Europe, and Japan use linear accelerators to treat companion animals.
Tumors are generally located at a shallower depth in companion animals than in humans, largely because these animals are smaller. Because X-ray beams in animals are not required to penetrate as deeply as the high-energy high-penetration beams used to treat human cancers, lower energy X-ray beams may be sufficient to treat cancers in these animals. Kilovoltage X-ray beams of 150 to 500 kV may be effective for treating cancers in animals. These beams require less energy than conventional megavoltage X-ray beams used to treat human cancers and do not require a device to create microwaves, making kilovoltage devices easier to manufacture and more cost-effective. In addition, kilovoltage devices are smaller and require less shielding than megavoltage devices, making kilovoltage devices easier to install. In this study, a commercial treatment-planning system was used to simulate treatment of various tumors in dogs with 300 kV, 450 kV, and 6 MV X-ray beams. The ability of kilovoltage X-ray machines to treat companion animals was evaluated by comparing the results of kilovoltage and megavoltage X-ray beam treatment.
GATE is an advanced, open-source software used for numerical simulations in medical imaging and radiotherapy [5162930]. To simulate radiation treatment using kilovoltage X-ray beams, the dependence of radiation dose distribution on depth, a parameter called percentage depth dose (PDD) [19], was calculated for the 300 kV and 450 kV X-ray beams by using GATE software (ver. 7.2). X-ray beams were generated by 300 kV [21] and 450 kV [22] X-ray point sources and passed through a filter and collimator into a water phantom. A 2-mm aluminum filter was used for the 300 kV X-ray beam and a Thoraeus III filter (0.6 mm tin + 0.25 mm copper + 1 mm aluminum) for the 450 kV X-ray beam. The water phantom had dimensions of 100 cm × 100 cm × 100 cm, and the PDD was calculated for five irradiation field sizes (3 cm × 3 cm, 6 cm × 6 cm, 10 cm × 10 cm, 15 cm × 15 cm, and 20 cm × 20 cm).
The commercial Core-PLAN (Seoul C&J, Korea) treatment-planning system [1718] calculates predicted radiation doses using the equivalent tissue-air ratio and collapsed cone convolution algorithms. In the present study, Core-PLAN (ver. 3.5.0.5) was used to calculate the predicted radiation doses for four tumor locations in dogs: brain (non-infectious meningoencephalitis), nasal cavity (malignant nasal tumors), forefoot (malignant muscular tumors), and abdomen (malignant intestinal tumors). The clinical target volume (CTV) was determined for each tumor, and the planning target volume (PTV) was defined as the CTV with margins of 0.1 cm. The CTV was composed of demonstrated tumors and all other tissues presumed to be tumors, and the PTV included the CTV and an additional volume accounting for patient movements and setup inaccuracies [1415]. Treatment plans were determined for application of three-dimensional conformal radiation therapy. In calculating doses, an inhomogeneity correction algorithm was applied to all treatment plans. For comparison, optimal plans based on the energy of the X-ray beams and tumor locations were designed. For example, plans for treating nasal and forefoot tumors with 6 MV X-ray beams included three 15° wedges and 1.5 cm thick boluses, respectively, whereas plans for all other tumors did not include wedges and boluses. Detailed information on treatment plans for each tumor site and X-ray beam energy are presented in Table 1. The same conditions were used for planning treatments with the 300 kV and 450 kV X-ray beams and were imported into CorePLAN by using GATE software. For comparison purposes, treatment planning was normalized so that treatment with all three X-ray beam energies resulted in coverage of the tumor with the same radiation dose, defined as > 95% of the prescribed dose being delivered to the PTV.
For quantitative comparisons, conventional comparative tools, including dose-volume histograms (DVH) [110], radiation dose homogeneity index (HI) of the tumor, and the radiation dose conformity index (CI) of the tumor, were calculated and compared with the results obtained for the 300 kV, 450 kV, and 6 MV X-ray beams. The DVH shows the relationship between radiation dose and tissue volume and is most frequently used to compare doses from different radiation treatments. The DVH column height represents the volume of the structure receiving greater than or equal to that dose. The DVH for each organ forms a single curve, providing quantitative information on the dose-volume relationship. Moreover, lower DVH column heights for normal organs indicate better radiation treatment.
HI is calculated using the formula: HI = (D2 − D98)/Dp × 100, where D2 and D98 are the doses received at 2% and 98% of the PTV, respectively, and Dp is the prescribed dose [31]. A lower HI therefore indicates better uniformity of the prescribed radiation dose to the tumor.
CI is calculated by using the formula: CI = PI/PTV, where PI corresponds to the prescription dose volume receiving doses above the prescribed dose [28]. A CI value close to one indicates that the prescribed dose was accurately delivered to the PTV. An X-ray beam energy device with a lower HI (or CI) than another X-ray beam energy device is therefore clinically superior.
Fig. 1 shows the PDDs for the 300 kV and 450 kV X-ray beams, obtained by using GATE, and for the 6 MV X-ray beam, obtained by using CorePLAN. At a field size of 20 cm × 20 cm, the build-up depths, representing the distance between the surface dose and the maximum dose [24], were 0.4, 0.8, and 1.6 cm for the 300 kV, 450 kV, and 6 MV X-ray beams, respectively, indicating that build-up distances were smaller for the 300 kV and 450 kV X-ray beams than for the 6 MV X-ray beam. In general, a greater build-up depth results in a greater skin sparing effect during radiotherapy. However, beams with a greater build-up depth also have a disadvantage, as they are less appropriate for the treatment of tumors at shallower depths. Compared with the 6 MV X-ray beam, the kilovoltage X-ray beams provided sharply lower radiation doses at depths of 0 to 10 cm. This depth-associated reduction in radiation dose was greatest for the 300 kV X-ray beam, with the difference in doses at various depths being slight for the 300 kV and 450 kV X-ray beams. The 300 kV and 450 kV X-ray beams showed a maximum difference of 7.08% and an average difference of 1.35%.
Figs. 2 and 3 show the simulation results for the treatment of nasal tumors and upper forefoot tumors, respectively, at various X-ray beam energies. A colored curve, often called the isodose, represents a contour area with the same radiation dose inside the animal. The colored scale bars for the various isodose boundaries are included in Figs. 2 and 3, respectively. The treatment simulation results were evaluated in the axial and sagittal planes after creating treatment plans using the 300 kV, 450 kV, and 6 MV X-ray beams. Although the differences were not significant, the radiation doses to normal tissues and tumors were better with the 6 MV X-ray beam than with the 300 kV and 450 kV X-ray beams.
Fig. 4 presents a summary of the DVHs of the tumor (PTV), the whole body, and the adjacent important organs for the treatment of nasal tumors. Although treatment of nasal cancers with the 6 MV X-ray beam provided better DVH results for the PTV than the 300 kV and 450 kV X-ray beams, all three provided similar DVHs for the whole body and adjacent organs (brain, eye, and lens). Table 2 presents the detailed DVH results for whole body and organ regions adjacent to the nasal tumors (brain, eye, and lens) that the percent of volume receiving at least 30% (V30), 60% (V60), and 90% (V90) of the prescribed dose. Lower V30, V60, and V90 values for normal organs are indicative of better radiation therapy.
In contrast, all three X-ray beam energies provided similar DVH results for the brain tumors PTV (Fig. 5). Although the DVHs of the 6 MV X-ray beam to the body and adjacent organs (brain, oral cavity, eye, and lens) were lowest, those values did not differ significantly from those of the 300 kV and 450 kV X-ray beams, suggesting that the three energies provided similar radiation results for the treatment of brain tumors.
Table 3 shows the HI and CI results for the four types of tumors. Whereas the 300 kV X-ray beam yielded the optimal (lowest) HI for the treatment of brain tumors, the 6 MV X-ray beam yielded optimal HI results for the treatment of nasal, forefoot, and abdominal tumors. In contrast, the 6 MV X-ray beam yielded optimal (lowest) CI results, regardless of the treatment site.
The present study compared the results of 300 kV, 450 kV, and 6 MV X-ray beams for radiotherapy of tumors in companion animals. The comparisons included assessment of radiation doses (via DVH evaluation) delivered to tumor and adjacent organs, as well as assessment of the associated HI and CI results.
The build-up distances for the 300 kV (0–0.4 cm) and 450 kV (0–0.8 cm) X-ray beams were much shorter than that for the 6 MV X-ray beam (1.6 cm), which may result in weaker skin protection. Analyses based on DVHs and V30, V60, and V90 doses suggested that all three X-ray beam energies yielded similar dose distributions when treating brain and nasal tumors. For brain tumors, the 300 kV X-ray beam yielded the optimal HI (6.3), whereas the 6 MV X-ray beam yielded the optimal CI (3.37). For the nasal, forefoot, and abdominal tumors, the 6 MV X-ray beam provided the optimal HI (9.1, 10.0, and 6.4, respectively) and CI (2.38, 1.17, and 1.22, respectively) values. The differences among the various beam energies for abdominal tumors may be associated with their relative depth; as well, abdominal tumors are larger (170.2 cm3) than brain (1.9 cm3), nasal (7.4 cm3), and forefoot (142.7 cm3) tumors.
Compared with the results of the kilovoltage X-ray beams, the megavoltage X-ray beam generally delivered lower doses to surrounding organs. These differences, however, were not statistically significant and may have negligible effect in the treatment of cancers in animals. The results indicate that radiotherapy using kilovoltage X-ray beams may be feasible in companion animals. Our results suggest that the manufacturing, management, and shielding advantages of kilovoltage X-ray beams could contribute to the more widespread use of radiotherapy for companion animals.
The present study was limited by the small number of tumors examined, suggesting the need for further research on the effects and effectiveness of kilovoltage X-ray beam treatment for companion animals.
Figures and Tables
Fig. 1
Percentage depth doses (PDD) of 300 kV, 450 kV, and 6 MV X-ray beams at field sizes of (A) 3 cm × 3 cm, (B) 6 cm × 6 cm, (C) 10 cm × 10 cm, (D) 15 cm × 15 cm, and (E) 20 cm × 20 cm.
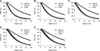
Fig. 2
Percent isodose distributions in nasal tumors (non-infectious meningoencephalitis or necrotizing meningoencephalitis). (A and B) Axial (A) and sagittal (B) views using a 300 kV X-ray beam; (C and D) axial and sagittal (D) views using a 450 kV X-ray beam; and (E and F) axial (E) and sagittal (F) views using a 6 MV X-ray beam. Colored scale bars indicate isodose levels normalized to the prescribed dose.
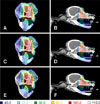
Fig. 3
Percent isodose distributions in forefoot tumors (malignant muscular tumor or anaplastic rhabdomyosarcoma). (A and B) Axial (A) and sagittal (B) views using a 300 kV X-ray beam; (C and D) axial (C) and sagittal (D) views using a 450 kV X-ray beam; and (E and F) axial (E) and sagittal (F) views using a 6 MV X-ray beam. Colored scale bars indicate isodose levels normalized to the prescribed dose.
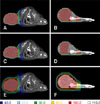
Fig. 4
Dose-volume histogram results for nasal tumors (malignant nasal tumor or transitional cell sarcoma) from the 300 kV, 450 kV, and 6 MV X-ray beams. Results are shown for (A) the planning target volume of the tumor, as well as for the (B) body, (C) brain, (D) left eye, (E) right eye, (F) left lens, and (G) right lens.
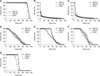
Fig. 5
Dose-volume histogram results for brain tumors (non-infectious meningoencephalitis or necrotizing meningoencephalitis) from the 300 kV, 450 kV, and 6 MV X-ray beams. Results are shown for (A) the planning target volume of the tumor, as well as for the (B) body, (C) oral cavity, (D) brain, (E) left eye, (F) right eye, (G) left lens, and (H) right lens.
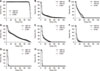
Table 1
Treatment plan information, including the numbers of fields and the use of wedges and boluses, for each tumor site and X-ray beam energy
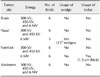
Acknowledgments
This work was supported by the National Nuclear R&D Program through the National Research Foundation of Korea (NRF), funded by the Ministry of Education, Science and Technology (NRF-2015M2A2A7A02045273 and NRF-2017M2A2A7081416); and the Ministry of Science and ICT (NRF-2017M2A2A6A01071189), Republic of Korea.
References
1. Austin-Seymour MM, Chen GT, Castro JR, Saunders WM, Pitluck S, Woodruff KH, Kessler M. Dose volume histogram analysis of liver radiation tolerance. Int J Radiat Oncol Biol Phys. 1986; 12:31–35.


2. Axlund TW, McGlasson ML, Smith AN. Surgery alone or in combination with radiation therapy for treatment of intracranial meningiomas in dogs: 31 cases (1989–2002). J Am Vet Med Assoc. 2002; 221:1597–1600.


3. Berlato D, Schrempp D, Van Den Steen N, Murphy S. Radiotherapy in the management of localized mucocutaneous oral lymphoma in dogs: 14 cases. Vet Comp Oncol. 2012; 10:16–23.


5. Buvat I, Lazaro D. Monte Carlo simulations in emission tomography and GATE: an overview. Nucl Instrum Methods Phys Res A. 2006; 569:323–329.


7. DeBoer DJ, Turrel JM, Moore PF. Mycosis fungoides in a dog: demonstration of T-cell specificity and response to radiotherapy. J Am Anim Hosp Assoc. 1990; 26:566–572.
8. Dobson JM, Samuel S, Milstein H, Rogers K, Wood JL. Canine neoplasia in the UK: estimates of incidence rates from a population of insured dogs. J Small Anim Pract. 2002; 43:240–246.


9. Dorn CR, Taylor DO, Schneider R, Hibbard HH, Klauber MR. Survey of animal neoplasms in Alameda and Contra Costa Counties, California. II. Cancer morbidity in dogs and cats from Alameda County. J Natl Cancer Inst. 1968; 40:307–331.
10. Drzymala RE, Mohan R, Brewster L, Chu J, Goitein M, Harms W, Urie M. Dose-volume histograms. Int J Radiat Oncol Biol Phys. 1991; 21:71–78.


11. Gardner H, Fidel J, Haldorson G, Dernell W, Wheeler B. Canine oral fibrosarcomas: a retrospective analysis of 65 cases (1998–2010). Vet Comp Oncol. 2015; 13:40–47.


12. Henry CJ, Brewer WG Jr, Tyler JW, Brawner WR, Henderson RA, Hankes GH, Royer N. Survival in dogs with nasal adenocarcinoma: 64 cases (1981–1995). J Vet Intern Med. 1998; 12:436–439.


13. Hutson CA, Willauer CC, Walder EJ, Stone JL, Klein MK. Treatment of mandibular squamous cell carcinoma in cats by use of mandibulectomy and radiotherapy: seven cases (1987–1989). J Am Vet Med Assoc. 1992; 201:777–781.
14. International Commission on Radiation Units and Measurements. Prescribing, Recording, and Reporting Photon Beam Therapy. Bethesda: International Commission on Radiation Units and Measurements;1993.
15. International Commission on Radiation Units and Measurements. Prescribing, Recording, and Reporting Photon Beam Therapy. Bethesda: International Commission on Radiation Units and Measurements;1999.
16. Jan S, Benoit D, Becheva E, Carlier T, Cassol F, Descourt P, Frisson T, Grevillot L, Guigues L, Maigne L, Morel C, Perrot Y, Rehfeld N, Sarrut D, Schaart DR, Stute S, Pietrzyk U, Visvikis D, Zahra N, Buvat I. GATE V6: a major enhancement of the GATE simulation platform enabling modelling of CT and radiotherapy. Phys Med Biol. 2011; 56:881–901.


17. Jung JY, Cho W, Kim MJ, Lee JW, Suh TS. Evaluation of beam modeling using collapsed cone convolution algorithm for dose calculation in radiation treatment planning system. Prog Med Phys. 2012; 23:188–198.
18. Jung JY, Cho W, Lee JW, Suh TS. The experimental verification of collapsed cone convolution algorithm for dose calculation in radiation treatment planning system. IFMBE Proc. 2013; 39:1211–1214.


19. Khan FM, Gibbons JP. Khan's the Physics of Radiation Therapy. Philadelphia: Lippincott Williams & Wilkins;2014.
20. MacEwen EG. Spontaneous tumors in dogs and cats: models for the study of cancer biology and treatment. Cancer Metastasis Rev. 1990; 9:125–136.


21. Mesbahi A, Zakariaee SS. Effect of anode angle on photon beam spectra and depth dose characteristics for X-RAD320 orthovoltage unit. Rep Pract Oncol Radiother. 2013; 18:148–152.


22. Miceli A, Thierry R, Flisch A, Sennhauser U, Casali F, Simon M. Monte Carlo simulations of a high-resolution X-ray CT system for industrial applications. Nucl Instrum Methods Phys Res A. 2007; 583:313–323.


23. Nolan MW, Kogan L, Griffin LR, Custis JT, Harmon JF, Biller BJ, Larue SM. Intensity-modulated and image-guided radiation therapy for treatment of genitourinary carcinomas in dogs. J Vet Intern Med. 2012; 26:987–995.


24. Padikal TN, Deye JA. Electron contamination of a high-energy X-ray beam. Phys Med Biol. 1978; 23:1086–1092.


25. Pang LY, Argyle DJ. Veterinary oncology: biology, big data and precision medicine. Vet J. 2016; 213:38–45.


26. Poirier VJ, Bley CR, Roos M, Kaser-Hotz B. Efficacy of radiation therapy for the treatment of macroscopic canine oral soft tissue sarcoma. In Vivo. 2006; 20:415–419.
27. Schiffman JD, Breen M. Comparative oncology: what dogs and other species can teach us about humans with cancer. Philos Trans R Soc Lond B Biol Sci. 2015; 370:20140231.


28. Shaw E, Kline R, Gillin M, Souhami L, Hirschfeld A, Dinapoli R, Martin L. Radiation Therapy Oncology Group: radiosurgery quality assurance guidelines. Int J Radiat Oncol Biol Phys. 1993; 27:1231–1239.


29. Strulab D, Santin G, Lazaro D, Breton V, Morel C. GATE (geant4 application for tomographic emission): a PET/SPECT general-purpose simulation platform. Nucl Phys B Proc Suppl. 2003; 125:75–79.

