Abstract
Background
Bioactive glass-ceramics have the ability to directly bind to bones and have been widely used as bone graft substitutes due to their high osteoconductivity and biocompatibility. CaO-SiO2-P2O5-B2O3 glass-ceramics are known to have good osteoconductivity and are used as bone graft extenders.
Methods
This study aimed to evaluate the effects of the resorbing properties of glass-ceramics in bone fusion after producing and analyzing three types of CaO-SiO2-P2O5-B2O3 glass-ceramics with high osteoconductivity that had enhanced resorption by having an increased B2O3 content. The three types of CaO-SiO2-P2O5-B2O3 glass-ceramics with B2O3 contents of 8.0, 9.0, and 9.5 weight % were designated and grouped as P20B80, P10B90, and P5B95, respectively. Glass-ceramic types were tested for fusion rates and bone formation by employing the lumbar 5-6 intertransverse process fusion model in 51 New Zealand male rabbits. Bioactivity was assessed by soaking in simulated body fluid (SBF).
Results
In vitro study results showed sufficient hydroxycarbonate apatite layer formation occurred for P20B80 in1 day, for P10B90 in 3 days, and for P5B95 in 5 days after soaking in SBF. For the rabbit lumbar spine posterolateral fusion model, the autograft group recorded a 100% fusion rate with levels significantly higher than those of P20B80 (29.4%), P10B90 (0%), and P5B95 (14.3%), with high resorbing properties. Resorbing property differences among the three glass-ceramic groups were not significant. Histological results showed new bone formation confirming osteoconductivity in all three types of glass-ceramics. Radiomorphometric results also confirmed the resorbing properties of the three glass-ceramic types.
Conclusions
The high resorbing properties and osteoconductivity of porous glass-ceramics can be advantageous as no glass-ceramics remain in the body. However, their relatively fast rate of resorption in the body negatively affects their role as an osteoconductive scaffold as glass-ceramics are resorbed before bony fusion.
Although grafting with autogenous iliac bone graft is one of the most commonly performed surgical methods used to create successful bone fusion, various types of artificial bone graft extenders have also been developed due to donor-site morbidity and supply problems.1,2) Artificial bone graft extenders have been widely used as these products pose no risk of disease transmission, are non-immunogenic, and can be produced in large quantities. Notably, ceramic bone graft extenders account for the largest segment of artificial bone graft extenders. Bioactive glass-ceramics bind directly to bone as they form a hydroxycarbonate apatite (HCA) layer.3,4) In addition, bioactive glass-ceramics have been widely used as bone graft substitutes because of their high osteoconductivity and biocompatibility,5,6) and Kitsugi et al.7) and Kokubo et al.8) have created new apatite-wollastonite glass-ceramics with both high mechanical strength and osteoconductivity.
CaO-SiO2-P2O5-B2O3 glass-ceramics are known to be non-toxic,3) provide more favorable osteoblastic differentiation for hMSC,4) and can be used as a coating material to enhance osseointegration between bones and implants.9,10) We reported that some compositions of CaO-SiO2-P2O5-B2O3 glass ceramics provide almost the same or better fusion rates and better resorption properties compared with those of apatite-wollastonite glass-ceramic (A-W GC).11) Ceramic bone graft substitutes with low resorption remain in the body for a long time even after fusion, and some compositions are nearly semipermanent. These ceramics remain in the body for a long time, are not remodeled and work as foreign materials in the long-term. As a result, if they become infected, the inflammation cannot be controlled without their removal and this can provoke long-term toxicity. Because of these reasons, glass-ceramics with high osteoconductivity and resorption which dissolve after fusion are considered to be ideal. A previous study revealed that higher B2O3 rates were associated with higher resorption rates,12) and in rabbit lumbar posterolateral fusion models, CaO-SiO2-P2O5-B2O3 glass-ceramics with a relatively low B2O3 rate recorded similar bone fusion rates and higher resorption compared to A-W GC known to have osteoconductivity.11) Against this background, we developed for this study new CaO-SiO2-P2O5-B2O3 glass-ceramics with higher B2O3 rates to improve resorption. To determine the effect of the new composition with increased resorption on bony fusion, an in vitro study using simulated body fluid (SBF) and an in vivo study using a rabbit lumbar posterolateral fusion model were conducted. Fusion rates and characteristics of the fused areas were analyzed.
Raw materials used to form the glass-ceramics were over 99.9% purity in SiO2, CaCO3, CaHPO4·2H2O, H3PO4, MgO, and CaF2, and 200 g of the composition of CaO 44.9 weight percent (wt%), SiO2 34.2 wt%, P2O5 16.3 wt%, MgO 4.6 wt%, and CaF2 0.5 wt% were batched. The batched sample underwent ball milling for 24 hours with absolute methanol as a solvent and was dried. After drying in the mixer, each batch was placed in a platinum crucible and melted at 1,500℃-1,600℃ for two hours. The glass-ceramic was manufactured through rapid cooling by pouring the material into a water bath and grounding the contents using spex milling for 15 hours to make around 1-2 µm sized glass powders. As a result, A-W GC was manufactured.
In addition, high purity CaCO3 (99.99%, Kojundo Chemical Co., LTD, Sakado-shi, Japan), SiO2 (99.9%, Kojundo Chemical Co., LTD, Sakado-shi, Japan) and B2O3 (99.9%, Kojundo Chemical Co., LTD, Sakado-shi, Japan) were used, and the composition of CaO 45.7%, SiO2 45.7% and B2O3 8.6% in the mole ratio was named as CS10B. Glass powders were made and then porous implants were manufactured. The ratios of A-W GC and CS10B were 1:4, 1:9 and 1:19 in P20B80, P10B90, and P5B95, respectively and the composition was CaO 45.54 wt%, SiO2 43.40 wt%, P2O5 3.26 wt%, B2O3 8.00 wt%, MgO 0.92 wt% and CaF2 0.10 wt% in P20B80 and CaO 43.57 wt%, SiO2 45.30 wt%, P2O5 1.62 wt%, B2O3 9.00 wt%, MgO 0.46 wt% and CaF2 0.05 wt% in P20B90 and CaO 43.51 wt%, SiO2 45.92 wt%, P2O5 0.81 wt%, B2O3 9.50 wt%, MgO 0.23 wt% and CaF2 0.025 wt% in P5B95. P20B80, P10B90, and P5B95 were maintained at 1,450℃ for two hours, and glass powders were produced by melting and cooling them rapidly. Sponge infiltration slurry was used to manufacture a sponge-type porous implant by mixing polyvinyl alcohol and the glass powders. The slurry was infiltrated three times by using 10 g of four appropriate-sized sponges (4 × 5 cm2) once each time. After making both the inner and outer parts infiltrated evenly, blocked pores were pierced with an air gun before the infiltration solution dried. The glass-ceramics manufactured through this method were dried by open air, and sponges were burnt at 600℃ to make sponge-type porous implants.
Fifty-one New Zealand white male rabbits with an average weight of 3.3 ± 0.3 kg were kept individually in standard cages under 12 hours on/off light cycles. Room temperature and humidity were maintained at 20℃ ± 10℃ and 40% ± 10%, respectively. All animals were divided into four groups by implant materials, i.e., P20B80 (18 rabbits), P10B90 (16 rabbits), P5B95 (9 rabbits), with added autografts (8 rabbits). The study was approved by the Institutional Animal Care and Use Committee at the Clinical Research Institute Seoul National University Hospital. Care was taken to avoid unnecessary stress and discomfort to the animals throughout the experimental period. Surgical procedures were performed to the lumbar vertebra (L5-L6) on both sides of intertransverse processes under general anesthesia as described by Boden et al.13) Three milliliters of glass-ceramics were implanted on each side of the intertransverse processes. This procedure was described in our previous reports.11,12) After the placement of the glass-ceramics, fascial tissue layers were repositioned and sutured after irrigation. Cefazoline 330 mg and penicillin G 400 mg were administered intramuscularly perioperatively for prophylaxis. All animals were euthanized 12 weeks postsurgery. The lumbar vertebrae were harvested, and all soft tissues were completely removed. Manual palpation to determine the fusion status was performed by stressing across the fusion site.
Bioactivity was investigated by in vitro testing using SBF. Mirror-polished specimens were soaked in SBF for 1-5 days. Formation of HCA on the surface of the specimens was observed by scanning electron microscopy (JSM5600, Jeol, Akishima, Japan).
Before tensile testing, all remaining muscle and ligaments including the intervertebral discs, posterior longitudinal ligaments, and the thecal sac were removed. Holes were made in the L5 and L6 vertebral bodies with a 3.2-mm-diameter drill bit, and then a 3.0-mm-diameter steel rod was inserted.11,12) An Instron electromechanical materials testing system (Instron 5582, Instron Co., Norwood, MA, USA) was used to test the L5 and L6 uniaxial tensile forces at a displacement rate of 0.5 cm per minute11,12) after fitting with a calibrated load-cell of 10 N. The ultimate tensile load, i.e., the break point, was read from the peak load to failure in the load-displacement curve.
A posteroanterior radiograph was completed for each animal immediately postsurgery at 45 kV for 12 milliseconds.11,12) Additional plain radiographs were obtained for all surviving animals at 2, 4, 6, 8, 10, 12 weeks postsurgery.
We examined whether clear opacity in the border of blocks of each implant immediate postsurgery became vague compared to adjacent areas, whether clear radioopacity among blocks presurgery became similar with that of neighboring blocks, and whether fusion mass connecting adjacent transverse processes was formed. We calculated the degradation rate of the glass-ceramics radiomorphometrically by comparing changes in the area occupied by the glass-ceramics between the initial and final radiographs which was described in previous reports.11,12) The percentage of the area of glass-ceramics was evaluated in the final radiographs (Fig. 1).
Histological sections were carried out in the same way as reported in previous studies.11,12) Lumbar spines from each group were fixed for 24 hours in 10% formalin. A sagittal section from the right and left sides was taken for processing and evaluated separately. Each section included the graft site and adjacent transverse processes. Undecalcified tissues were embedded in methacrylate and sectioned 4 µm in width parallel to the fusion mass with a diamond cutter (Exakt300cp, Exakt Co., Norderstedt, Germany).13) Slides were stained using hematoxylin and eosin. Satisfactory osteoconductivity was defined as new bone was detected in pores or adjacent to the ceramics.
The degree and the pattern of bony fusion between bone and implant and the surface change of the fusion mass were observed with a light microscope. Moreover, the degree of bone growth was evaluated by classifying parts of the fusion mass into an implant, new bone, and soft tissues.
Of the fifty-one rabbit specimens initially used in this study, eight experimental animals were lost immediately after surgery and during the healing phase. The remaining forty-three animals were completely evaluated. Four groups were designated according to the implanted material or autograft and the number of specimens were evaluated per group. Group names and their numbers are as follows: P20B80 group, 17 specimens; P10B90 group, 11 specimens; P5B95 group, 7 specimens; and the autograft group, 8 specimens.
After soaking in SBF for 1 day, an HCA layer covered the whole surface of 25% of the specimens in the P20B80 group. However, an HCA layer appeared 3 days after soaking in SBF in the P10B90 group and 5 days after soaking in SBF for the P5B95 group. Moreover, the aggregate of the HCA layer for groups P10B90 and P5B95 was smaller in comparison with that of the P20B80 group (Fig. 2).
Based on manual palpation, solid bone fusion was detected in 5 of 17 specimens (29.4%) in the P20B80 group, 0 of 11 (0.0%) in the P10B90 group, 1 of 7 (14.3%) in the P5B95 group, and 8 of 8 (100.0%) in the autograft group. The autograft group recorded significantly higher fusion rates than all the implanted materials groups of P20B80, P10B90, and P5B95 (p < 0.0001, 0.0016 and 0.0014, respectively). The fusion rate for the P20B80 group was higher than those of the P10B90 and P5B95 groups. However, the difference was statistically insignificant.
The uniaxial tensile test showed 214.9 ± 46.8 N of the ultimate tensile load for the P20B80 group, 187.1 ± 44.9 N in the P10B90 group, and 230.3 ± 13.9 N in the P5B95 group. The ultimate tensile load of the control group, i.e., the autograft, was slightly higher than those of the other groups although there was no significant difference.
The degree of resorption increased at 12 weeks compared to that immediately postsurgery in the implanted material P20B80, P10B90, and P5B95 groups, and the implants formed one mass (Fig. 3). In addition, the radio-opaque area was inside the fusion mass, and it was, therefore, considered that new bone grew into it and bony fusion was in the process. The proportions of the area occupied by the glass-ceramics at the final radiographs to the area occupied by the glass-ceramics at the initial radiographs were 76.6% ± 7.0% in the P20B80 group, 74.2% ± 9.0% in the P10B90 group, and 75.8% ± 5.8% in the P5B95 group. The radiomorphometry of the three kinds of CaO-SiO2-P2O5-B2O3 glass-ceramic areas revealed no statistical difference with time.
According to the histological study, satisfactory osteoconductivity was found in all of the three types of CaO-SiO2-P2O5-B2O3 glass-ceramics. In the P20B80, P10B90, and P5B95 groups, new bone was formed around the ceramics inserted between the upper and lower transverse processes continuously. Undecalcified examination revealed that the glass-ceramic implants had very low opacity, and the brown ceramic implant was absorbed losing nearly all its original pattern. New bone had osteoblasts and osteocytes in the adjacent and central areas, respectively, and the formation of lamellar bone was observed with both osteocytes arranged to be in concentric circles and mature bone matrices (Fig. 4). The border of the composite plane between implants and bone became vague when the implants were absorbed. It is assumed that the glass-ceramics were absorbed during the formation of bone tissues on the ceramic implants, and the absorption occurred mainly in areas in contact with bone.
One representative of osteoconductive glass-ceramics used for bone graft extenders is hydroxyapatite. Hydroxyapatite is clinically applied in cases of bone defects and spinal fusion.13-15) However, its resorbing properties and mechanical strength are considered relatively low. Many studies have been conducted to show enhanced resorption and better mechanical strength. Although calcium phosphate ceramics with tricalcium phosphate are known to have high resorbing properties,16) their low mechanical strengths have been commented as a distinct disadvantage. Because bioactive glass-ceramics can control resorption and mechanical strength according to their composition, this study also tried to regulate their resorbing properties with B2O3 content. The correlation that a higher B2O3 content leads to higher resorption was displayed in our previous study. In the in vitro study using SBF, P20B80 (P2O5 3.26 wt%) formed an HCA layer after soaking for 1 day, while P10B90 (P2O5 1.62 wt%) and P5B95 (P2O5 0.81 wt%), with their relatively low P2O5 contents, began to form a layer in proportion to the P2O5 content and its amount was also proportional to the P2O5 content. However, the difference in the amount of the HCA layer produced after five days was not significantly different between the two compounds. The difference in the time in forming the HCA layer was notably considered to be attributed to the P2O5 content of each glass-ceramic. Although P2O5 is inessential for the nucleation of HCA, it can earlier increase the amount of the HCA layer as it accelerates HCA formation. With regards to this point, decreased P2O5 content could influence osteoconductivity.
For these reasons, the increased B2O3 and decreased P2O5 content that were used to improve the resorption of the glass-ceramics was considered to decline eventually in fusion rates as displayed in the in vivo study. This study measured the two-dimensional area of the implanted glass-ceramics in radiographic images to assess their resorption. Because the connection of trabecular bone found in complete fusions was unclear in groups P20B80, P10B90, and P5B95, those groups were assessed to achieve partial fusion. In comparison, the autograft group was found to have bony bridging in the upper and lower transverse processes at 12 weeks; and, thus, solid bony fusion formed. The rate of the absorbed area was only around 25% and, thus, it was considered an inadequate resorption rate. When observing the measured border of the radioopaque lesions, the implanted glass-ceramic material remained as debris after the area of the absorption of the porous structure became radio-opaque. As a result, it was evaluated as not being absorbed. Gross inspection of the glass-ceramics implants revealed that the porous structure was nearly lost, and the absorption of the glass-ceramics used in this study was much higher than that in previous studies.
In our previous study, the fusion rate of compositions with relatively higher P2O5 rates and A-W GC was 80%-90%.11) For this study, the fusion rate of P20B80 with the highest level of P2O5 was recorded as only 29.4%, and most of the P10B90 and P5B95 groups showed pseudarthrosis. Conversely, the histological examination showed excellent osteoconductivity found in all three glass-ceramics. In other words, newly formed bony tissues were bonded directly to the implanted glass-ceramics and remodeling also proceeded. Thus, it is believed that the decreased P2O5 content affected osteoconductivity to some degree. Nevertheless, the osteoconductivity of all three glass-ceramics types was outstanding. Therefore, a higher B2O3 content, rather than osteoconductivity itself, led to poorer early porous structures for increased resorption and was considered to be a major cause of pseudarthrosis. In general, for bone defect filling, stem cells are supplied in neighboring parts of the implanted glass-ceramics, and the vascular structure grows into it easily. Thus, this resorption characteristic is considered to be highly advantageous. In the case of intertransverse process fusions, because the transverse processes mainly consist of cortical bone with very small amounts of stem cells and the distance between transverse processes is relatively long, some parts close to the bone can form new bone by receiving stem cells. However, the middle area between the transverse processes is difficult to attain fusion as this area needs the connection of new bones produced from both transverse processes. Therefore, to obtain intertransverse process fusion, porous structures and osteoconductivity of the osteoconductive scaffolds should be maintained sufficiently during the period needed for newly formed bone to grow and be connected in neighboring areas. For intertransverse process fusion with regards to this study, the three types of glass-ceramics used had excellent osteoconductivity but could not maintain the porous structure fully to prevent pseudarthrosis.
Properties of the ideal biodegradable material include: (1) the ability to maintain strength and stability of porous structures during degradation until achieving fusion in the early stage, (2) the ability to be replaced completely into the new bone, (3) and having adequate reconciling resorption and new bone formation rates. However, materials meeting all of these conditions have yet to be invented. For one reason, the absorption of porous glass-ceramics is performed through a slow degradation of the debris made by the destruction of the porous structure rather than by the maintenance of the porous structure, which is critical for osteoconductivity. Unfortunately, the destruction of a porous structure actually negatively influences various aspects of osteoconductivity. Moreover, the environment for bone formation in the implanted area varies according to anatomical location. However, even when implants are made in the same anatomical location, large differences in time and success rates of bone formation can depend on age and the health condition of the recipients. Therefore, in a favorable environment for bone formation, glass-ceramics with high absorption rates can produce an ideal situation, obtaining not only bony fusion but also absorption. Conversely, the same glass-ceramics in an unfavorable environment for bone formation can experience pseudarthrosis due to absorption taking place before new bone formation can occur.
From all of these results, osteoconductivity and sufficient porous structure maintaining properties rather than resorbing properties are considered to be more advantageous for bony fusion in an environment unfavorable for new bone formation. Furthermore, resorption rates faster than new bone formation growth rates need careful attention as an imbalance may lead to failure. However, in cases using bone morphogenetic protein with high osteoinductivity and stem cells with properties of both osteoinductivity and osteogenesis, osteoconductive bio glass-ceramics with high resorbing properties are expected to be used as carriers. In conclusion, the high resorbing properties of osteoconductive porous glass-ceramics have a material advantage of completely dissolving within the human body. However, their ability to be rapidly resorbed before bony fusion in their role as osteoconductive scaffolds is a disadvantage that must be taken into consideration.
Figures and Tables
Fig. 1
Radiomorphometric analysis. The percentage of the area of glass-ceramics at 12 weeks postoperatively (PO) compared to that immediately postoperatively (IPO).
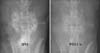
Fig. 2
In vitro results using simulated body fluid (SBF). Formation of the hydroxycarbonate apatite layer after soaking in SBF was observed in 1 day in the P20B80 group (A), in 3 days in the P10B90 group (B), and in 5 days in the P5B95 group (C).

Fig. 3
Radiological results of the P20B80, P10B90, P5B95, and autograft groups. The autograft group was considered to have fusion because the trabecular bridging between the upper and lower transverse processes was clear. In comparison, the volume of the graft with the three glass-ceramics types declined at 12 weeks postoperatively from the immediate postoperative (IPO) levels, and was, thus, thought to be resorbed.

Fig. 4
Undecalcified histologic findings of the three glass-ceramics implant types at 12 weeks postimplantation (H&E, ×12.5, ×40, ×100) in the P20B80 (A), P10B90 (B), and P5B95 (C) groups. Newly formed bony tissues were found in and around all three kinds of glass-ceramic types. Arrows: newly formed bone, Stars: implanted glass-ceramics.
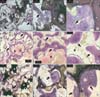
ACKNOWLEDGEMENTS
This work was supported by a clinical research grant-in-aid from the SMG-SNU Boramae Medical Center (No. 01-2013-11).
References
1. Schnee CL, Freese A, Weil RJ, Marcotte PJ. Analysis of harvest morbidity and radiographic outcome using autograft for anterior cervical fusion. Spine. 1997; 22(19):2222–2227.
2. Silber JS, Anderson DG, Daffner SD, et al. Donor site morbidity after anterior iliac crest bone harvest for single-level anterior cervical discectomy and fusion. Spine. 2003; 28(2):134–139.
3. Lee JH, Chang BS, Ryu HS, Lee CK. A 90-day subchronic toxicity study of beta-calcium pyrophosphate in rat. Drug Chem Toxicol. 2009; 32(3):277–282.
4. Lee JH, Lee KM, Jang SJ, Lee HS, Baek HR. Effects of bisphosphonate-treated bioactive ceramic grafts for the proliferation and osteoblastic differentiation of human bone marrow mesenchymal stem cells. Tissue Eng Regen Med. 2011; 8(1):69–77.
5. Ono K, Yamamuro T, Nakamura T, et al. Apatite-wollastonite containing glass ceramic-fibrin mixture as a bone defect filler. J Biomed Mater Res. 1988; 22(10):869–885.
6. Schepers E, de Clercq M, Ducheyne P, Kempeneers R. Bioactive glass particulate material as a filler for bone lesions. J Oral Rehabil. 1991; 18(5):439–452.
7. Kitsugi T, Yamamuro T, Nakamura T, et al. Bone bonding behavior of three kinds of apatite containing glass ceramics. J Biomed Mater Res. 1986; 20(9):1295–1307.
8. Kokubo T, Ito S, Huang ZT, et al. Ca,P-rich layer formed on high-strength bioactive glass-ceramic A-W. J Biomed Mater Res. 1990; 24(3):331–343.
9. Lee JH, Ryu HS, Lee DS, Hong KS, Chang BS, Lee CK. Biomechanical and histomorphometric study on the bone-screw interface of bioactive ceramic-coated titanium screws. Biomaterials. 2005; 26(16):3249–3257.
10. Lee JH, Nam H, Ryu HS, Seo JH, Chang BS, Lee CK. Bioactive ceramic coating of cancellous screws improves the osseointegration in the cancellous bone. J Orthop Sci. 2011; 16(3):291–297.
11. Lee JH, Park KW, Song KS, et al. Evaluation of osteosynthesis in CaO-SiO2-P2O5-B2O3 glass-ceramics by posterolateral fusion of rabbit lumbar vertebrae. J Korean Soc Spine Surg. 2005; 12(1):1–11.
12. Lee JH, Lee CK, Chang BS, et al. In vivo study of novel biodegradable and osteoconductive CaO-SiO2-B2O3 glass-ceramics. J Biomed Mater Res A. 2006; 77(2):362–369.
13. Boden SD, Schimandle JH, Hutton WC. An experimental lumbar intertransverse process spinal fusion model: radiographic, histologic, and biomechanical healing characteristics. Spine. 1995; 20(4):412–420.
14. Lee JH, Hwang CJ, Song BW, Koo KH, Chang BS, Lee CK. A prospective consecutive study of instrumented posterolateral lumbar fusion using synthetic hydroxyapatite (Bongros-HA) as a bone graft extender. J Biomed Mater Res A. 2009; 90(3):804–810.
15. Boden SD, Martin GJ Jr, Morone M, Ugbo JL, Titus L, Hutton WC. The use of coralline hydroxyapatite with bone marrow, autogenous bone graft, or osteoinductive bone protein extract for posterolateral lumbar spine fusion. Spine. 1999; 24(4):320–327.
16. Kraal T, Mullender M, de Bruine JH, et al. Resorbability of rigid beta-tricalcium phosphate wedges in open-wedge high tibial osteotomy: a retrospective radiological study. Knee. 2008; 15(3):201–205.