Abstract
In pediatric living-related liver transplantation, preoperative evaluation of the recipient is important for surgical planning, while the accurate diagnosis of postoperative complications is essential for graft salvage. Multiplanar and three-dimensional imaging using multi-slice spiral CT can be used for preoperative vascular imaging, as well as for evaluating postoperative complications. In this essay, we describe the usefulness of multi-slice CT, combined with a variety of different reconstruction techniques, for the preoperative evaluation of transplant recipients. In addition, we demonstrate the multi-slice CT findings of postoperative complications, including vascular stenosis or thrombosis, bile duct leak or stricture, and extrahepatic fluid collection.
For pediatric patients with end-stage liver disease, living-related liver transplantation has become not only an important therapeutic option but a life-saving procedure (1). The precise preoperative evaluation of anatomic variations in the vascular structure is essential for successful transplantation (2). In addition, the early detection of postoperative complications, including vascular stenosis and thrombosis, stricture of the biliary-enteric anastomosis, extrahepatic fluid collection such as biloma or hematoma and rejection, is crucial for graft salvage (3). The generally recognized advantage of CT is the accurate evaluation of hepatic parenchymal and extrahepatic abnormalities. Recently, there have been several reports which suggested that multi-slice spiral CT may allow the noninvasive creation of an accurate vascular map, and may be an alternative to conventional angiography in children (4), although conventional angiography is still necessary in cases where the imaging findings are not conclusive or prior to interventional procedures.
In this article, we illustrate the usefulness of multi-slice CT with variable reconstruction techniques, including multiplanar reformatting (MPR), maximum intensity projection (MIP), minimum intensity projection (MinIP), and volume rendering (VR), for the preoperative evaluation of hepatic vascular structures and for the evaluation of postoperative complications.
Four-slice spiral CT (Lightspeed QX/i; GE Medical Systems, Milwaukee, WI) was performed using the following parameters: 1.25-mm collimation, 3.75-7.5-mm table feed and 0.5-0.7-mm reconstruction interval. Sixteen-slice spiral CT (SOMATOM Sensation 16; Siemens, Forchheim, Germany) was performed using the following parameters: 0.75-1.5-mm collimation, 12-24-mm table feed, and 0.5-0.7-mm reconstruction interval. According to the recommendation of the 'As Low As Reasonably Achievable' (ALARA) concept in pediatric CT, a weight-based low-dose CT protocol (80-100 kVp and 60-140 mAs) with on-line CT dose modulation was used in all CT examinations. Contrast enhanced CT scanning was performed with a biphasic technique, following the intravenous injection of 2 ml/kg iopromide (Ultravist 370; Schering, Berlin, Germany) using a power injector at the maximum allowable rate given the capacity of the catheter. An automatic bolus-tracking technique was used to determine the proper timing of the arterial phase. Portal venous phase CT images were obtained 50-65 seconds after the beginning of contrast injection and were based on the body weight of the patient in each case. The multi-slice CT protocol is summarized in Table.
The acquired images were transferred to a commercial workstation (Advantage Windows 4.1; GE Medical Systems), and post-processing was performed using a variety of different reconstruction techniques. Among these reconstruction techniques, MIP and MPR were used mainly for evaluating the hepatic vascular structures. MPR seemed to be superior to MIP for measuring the diameter of the hepatic vessels and for detecting small portal vein thromboses or intra-stent abnormalities. Curved planar reformation was used to delineate the entire length of the complex and tortuous structures, including varices. MinIP from portal venous phase CT data was used to evaluate the biliary tree. VR, the most complex reconstruction technique, may give surgeons useful presurgical information on the three-dimensional (3D) relationships of the vessels of interest. Overall postprocessing took about 30 minutes per patient.
Sedation was necessary in children younger than 6 years of age or uncooperative patients. For children less than 1 year old, oral chloral hydrate was usually administered, most commonly at an initial dose of 50 mg/kg, with additional doses of 25-50 mg/kg as needed. Intravenous sedatives, including midazolam (0.02-0.05 mg/kg) and ketamine (0.5-1.0 mg/kg) were used as the initial drug in children more than 1 year old.
The common hepatic artery arises from the celiac trunk in 83% of individuals (5). After the first major branch, namely the gastroduodenal artery, it continues as the proper hepatic artery. The classic hepatic arterial branching pattern, consisting of the right, left and middle hepatic arteries originating from the common hepatic artery, is present in 55% of individuals. The middle hepatic artery may arise from the left (45%) or right (45%) hepatic artery or from other sites (10%) (5) (Fig. 1).
Preoperative recognition of hepatic arterial variation is important for surgical planning. Anatomic variations in the hepatic artery may require complex surgical reconstruction or vascular graft interposition during anastomosis, especially when the artery is of insufficient length or small size (1). Variations in the hepatic artery are accurately delineated on 3D images (Figs. 2, 3, 4).
The patency and diameter of the extrahepatic portal vein are the most important parameters obtained from the preoperative evaluation (6). Infants and young children with end-stage liver disease, particularly those with biliary atresia, may have diminutive portal veins (Fig. 5), which may necessitate operative dissection back to the confluence of the splenic and superior mesenteric veins for the purpose of anastomosis with the donor portal vein or the interposition of a portal venous graft. If the diameter of the main portal vein is less than 4 mm, the vein should be traced back to its origin and measured at the confluence of the splenic and superior mesenteric veins (6). Portal vein thrombosis is a common complication of liver cirrhosis. It may complicate surgical procedures during transplantation, especially when the proximal portion of the portal vein is involved. The extents of portal vein thrombosis and periportal collateral formation are accurately delineated on 3D reconstructed images (Fig. 6).
Less frequently encountered are anomalies of the inferior vena cava (IVC), including absent or hypoplastic IVC with azygos continuation (Fig. 7). Although these anomalies are relatively unimportant in the case of the piggyback operation, they may complicate liver transplants with outflow anastomoses (7).
Spontaneous portosystemic varices are commonly found in cirrhotic patients and may have detrimental effects on graft perfusion. Based on the preoperative evaluation, varices can either be ligated during surgery or embolized to increase graft perfusion and to reduce the risk of intraoperative bleeding (7). Multi-slice CT can accurately demonstrate the size and distribution of varices (Figs. 8, 9, 10, 11).
Recently, liver transplantation has been reported in children with such hepatic tumors as hepatocellular carcinoma and hepatoblastoma (8). In cases of hepatocellular carcinoma, liver transplantation is feasible in patients with stage I or II disease, as determined by the American Liver Tumor Study Group Modified Tumor-Node-Metastasis staging classification (6). Extrahepatic metastases are an absolute contraindication for liver transplantation. Therefore, the CT scan has an important role to play in the detection of malignant hepatic tumors (Fig. 12) and can be used to assess their extent prior to liver transplantation.
Hepatic artery thrombosis is the most common vascular complication, occurring in 9% to 42% of children receiving transplants (9). Hepatic artery thrombosis most often occurs within the first 2 months after transplantation and frequently results in graft failure, leading to increased morbidity and mortality. Recent technical advances, including microscopic surgery, the use of anticoagulants such as aspirin and low molecular weight heparin, and the use of intraoperative Doppler ultrasound (US), have reduced the incidence of hepatic arterial thrombosis (10). Doppler US is commonly used to detect hepatic artery stenosis or thrombosis through the detection of hemodynamic changes. However, Doppler US may not directly visualize the stenosed hepatic artery and, unlike CT, the accuracy of its findings may be dependent on the skill of the individual sonographer and on the patient's body habitus. In contrast, CT angiography using multi-slice CT can accurately depict the location and extent of stenosis and thrombosis of the hepatic artery with excellent spatial resolution (11) (Figs. 13A, 13B). In addition, CT may be able to better demonstrate any parenchymal abnormality of the transplanted liver. On CT, hepatic infarction is well delineated as an irregular and wedge-shaped low-attenuation lesion located at the periphery of the liver (Fig. 13C). However, the CT findings need to be carefully evaluated, because CT angiography tends to overestimate stenosis and is limited by poor visualization of the distal small branches. In addition, surgical clips at the anastomosis site of the hepatic artery can cause beam hardening artifacts, which may complicate the assessment of the patency of the arterial anastomosis site. Targeted reformatting with a thin slab thickness may mitigate such artifacts around the anastomosis site.
Arterioportal shunts following liver biopsy tend to occur quite frequently, but most resolve spontaneously. In addition, symptomatic patients may present with gastrointestinal bleeding, hemoperitoneum or hemobilia, all of which require endovascular coil embolization. Pseudoaneurysm (Fig. 14) after liver transplantation is an uncommon but potentially fatal complication. It usually occurs at the vascular anastomosis, although it may also occur following percutaneous biopsy, biliary procedures or local infection.
Portal vein complications following liver transplantation are relatively uncommon, with an incidence of only 1% to 6% (12). They may result from the use of faulty surgical techniques, the excessive length of the interposed vascular graft, vessel misalignment, hypercoagulable states, previous portal vein surgery or previous portal vein thrombosis (9). Significant portal vein stenosis (Fig. 15) may be difficult to diagnose with CT or other noninvasive imaging techniques, because mild or insignificant narrowing of portal venous anastomosis is a common finding, which may result from discrepancies between the calibers of the donor and recipient portal veins or from a plication deformity. In the absence of portal hypertension, this finding has no clinical significance (13). Significant portal vein stenosis can be suspected, however, if the poststenotic dilatation of the portal vein is found to have progressed on a follow up CT scan. In addition, portal hypertension may be suspected when a follow up CT scan reveals increased spleen size, the development or increase of spontaneous collaterals, or an increase in the amount of ascites. Thus, initial CT angiography obtained during the early postoperative period should be used as the baseline for comparison with follow-up CT angiography. By comparing the initial and follow-up MPR images obtained in the same plane, subtle changes in the diameter of the portal vein can be detected. In addition, the effect of the growth of the transplanted liver should be considered when evaluating the portal vein, because the enlarged transplanted liver may compress the intrahepatic portion of the intact portal vein, thereby resulting in a false diagnosis of portal vein stenosis. On the other hand, portal vein thrombosis can be observed as a low-attenuation filling defect in the portal vein during contrast-enhanced CT. In some instances, the stenosed extrahepatic portal vein may be completely occluded (Fig. 16), and its early detection is important for successful interventional reopening of the occluded portal vein. Multi-slice CT with variable reconstruction techniques can provide excellent visualization of stenosis, thrombosis and obstruction of the portal vein (11).
Balloon angioplasty, with or without stent placement, is an effective treatment for significant portal vein stenosis. The patency of the inserted stent may be difficult to evaluate with US or MR imaging. Although beam hardening artifacts may disturb the evaluation of the intra-stent portion, the intraluminal patency of the stent can be assessed via contrast enhancement in the intra- and post-stent portions of the portal vein on CT (Fig. 17).
Hepatic vein and IVC complications are rare, occurring in as little as 1% to 4% of transplants (14). These complications usually result from technical problems or compression of the vessel caused by fluid collection (Fig. 18). Although hepatic venous anastomotic stenoses may occur more frequently following living-related liver transplantation than following whole liver transplantation, the incidence of these complications has been found to have decreased following the introduction of improved surgical techniques, including the triangulation technique (15). A decrease in the pulsatility of the waveform from the same hepatic vein, accompanied by an increase in the amount of ascites, on follow-up US suggests significant stenosis between the hepatic veins and IVC. Multi-slice CT can clearly delineate an anastomotic narrowing between the hepatic vein and IVC (Fig. 19), which may not be demonstrated on US, due to poor sonographic windows during the early postoperative periods, as well as liver parenchymal changes due to hepatic congestion. The multi-slice CT results must be correlated with both the US findings and the clinical signs, in order to determine their real significance.
Biliary complications in pediatric living-related liver transplantation are associated with increased morbidity and mortality and occur with incidences of 12% to 50% (16). These biliary complications include bile leak, bile duct stricture and dilatation, intrahepatic biloma, stone formation and pneumobilia (Fig. 20), with most occurring within 3 months of transplantation. Most biliary strictures occur at the anastomotic site and may be secondary to scar formation in the biliary tree. Although percutaneous dilatation with stenting may resolve such problems, repeat surgery is occasionall required. Nonanastomotic, intrahepatic and multiple strictures are generally caused by ischemia, due to hepatic artery stenosis or thrombosis or preservation injury. US and MR cholangiopancreatography constitute useful noninvasive methods of evaluating the biliary tree (16). Multi-slice CT, especially when combined with the MinIP technique, can also be used to demonstrate the presence of biliary complications. Mild intrahepatic bile duct dilatation is often observed in the transplanted liver and is not specific to anastomotic stenosis (17). When the CT scan reveals localized or progressive duct dilatation (Fig. 21), however, care should be taken to ensure timely diagnosis and appropriate intervention.
Localized extrahepatic fluid collections include hematomas, bilomas (Fig. 22), seromas and localized ascites. These are common early postoperative findings and usually diminish in size over a period several weeks without the need for any specific treatment (17). However, reoperation or percutaneous drainage may be required when ongoing bleeding, bile leakage, superinfection, or IVC or portal vein compression caused by large fluid collections is suspected (17).
Hematomas are easily detected on CT in the form of fluid with high attenuated. They can be caused by inadequate hemostasis, leaking vascular anastomoses, rupture of a hepatic pseudoaneurysm or intraperitoneal bleeding after liver biopsy.
Multi-slice CT with multiplanar and three-dimensional reconstruction allows excellent visualization of vascular and biliary morphologies in cases of pediatric living-related liver transplantation. These CT images, which accurately depict various anatomic details, are therefore helpful in establishing surgical plans and in evaluating a variety of postoperative complications. Although multi-slice CT cannot determine the functional significance of anastomotic vascular narrowing, it may help in the early detection of such complications and can play an important complementary role, as long as other noninvasive imaging findings and clinical signs are appropriately integrated.
Figures and Tables
Fig. 1
Normal anatomy of the hepatic artery in an 1-year-old girl. Oblique axial maximum intensity projection image shows the right hepatic artery (RHA), left hepatic artery (LHA), splenic artery (SA), and left gastric artery (LGA). Two middle hepatic arteries (arrows) arise from the right hepatic artery.
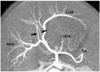
Fig. 2
Replaced right hepatic artery in an 1-year-old girl. Oblique coronal maximum intensity projection (A) and volume rendering (B) images show a replaced right hepatic artery (RHA) arising from the superior mesenteric artery (SMA). Unlike the maximum intensity projection image, the volume rendering image maintains the spatial relationships and depth information and demonstrates the left hepatic artery (long arrow, B) and left gastric artery (short arrow, B) as well.
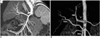
Fig. 3
Replaced left hepatic artery in a 6-year-old girl. Oblique coronal maximum intensity projection images show a replaced left hepatic artery (arrowheads) arising from the left gastric artery (long arrow). Note the artifact (short arrows) resulting from respiratory motion. RHA = right hepatic artery
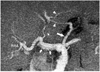
Fig. 4
Celiacomesenteric trunk in a 10-year-old boy. Volume rendering image shows the celiacomesenteric trunk (long arrow). The celiac and superior mesenteric arteries have a common origin. The splenic artery (short arrow) and the left gastric artery (arrowheads) arise from the celiac artery. RHA = right hepatic artery, LHA = left hepatic artery, GDA = gastrointestinal artery
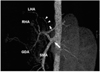
Fig. 5
Portal vein stenosis in an 1-year-old girl. Oblique axial maximum intensity projection image shows a small main portal vein (long arrow). Both the right and left portal veins have very small diameters (arrows). Prominent hepatic arteries (*) are noted at the hepatic hilum.
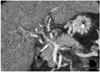
Fig. 6
Portal vein thrombosis in a 9-year-old boy. Oblique coronal multiplanar reformatted image shows portal vein thrombosis (arrow) in the right anterior portal branch with irregular periportal enhancement, which was not identified on Doppler sonography.
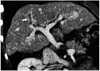
Fig. 7
Anomalies of the inferior vena cava.
A. Absent intrahepatic portion of the inferior vena cava in a 2-year-old girl. multiplanar reformatting image shows obliteration of the intrahepatic portion of the inferior vena cava (arrowheads). Note the intact suprahepatic portion of the inferior vena cava (arrow). Recognition of this portion can help in planning hepatic venous reconstruction during transplantation.
B. Hypoplastic inferior vena cava in a 14-year-old girl. Curved planar reformatted image shows diffuse narrowing of the suprarenal portion of the inferior vena cava (arrowheads) with engorged hemiazygos veins (arrows).
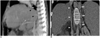
Fig. 8
Esophageal and coronary varices in a 6-year-old girl. Coronal multiplanar reformatting image reveals the presence of a coronary varix (short arrow). The esophageal varix (long arrow) is observed to communicate with the left gastric vein through the gastric fundal varix. L = liver, St = stomach, Sp = spleen
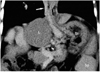
Fig. 9
Spontaneous splenorenal shunt in a 6-year-old girl. Oblique coronal maximum intensity projection image shows a prominent splenorenal shunt (arrows). LRV = left renal vein
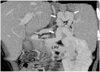
Fig. 10
Perihepatic collaterals in a 7-year-old boy. Oblique coronal maximum intensity projection image shows collateral vessels (arrows) at the perihepatic area. The collateral vessels adjacent to the liver can be ligated during surgery, because otherwise they can cause increased bleeding when the native liver is excised.
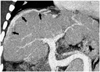
Fig. 11
Hemorrhoidal vein in a 6-month-old girl. Volume rendering image well delineates its continuation with the inferior mesenteric vein (arrowheads). Diffuse narrowing of the portal vein (arrows) is noted. RGV = right gastric vein, LGV = left gastric vein, SV = splenic vein, PEV = paraesophageal vein, SMV = superior mesenteric vein, IVC = inferior vena cava
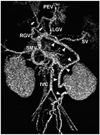
Fig. 12
Hepatocellular carcinoma in a 2-year-old boy.
A. Arterial-phase CT scan shows a hypervascular mass (arrow) in the right lobe of the liver. The underlying liver reveals a cirrhotic change.
B. Portal-phase CT scan at the same level shows wash-out of contrast agent from the tumor. Based on characteristic enhancement pattern of the mass on CT, hepatocellular carcinoma was diagnosed, and the diagnosis was confirmed on histopathology.
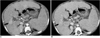
Fig. 13
Hepatic artery stenosis in an 11-year-old girl, 18 days after living-related liver transplantation to treat fulminant hepatitis.
A. Coronal multiplanar reformatting image shows severe multifocal stenoses of the hepatic artery at the anastomosis sites (arrowheads).
B. Hepatic arteriogram demonstrates the hepatic artery stenoses (arrowheads) with distal sluggish flow. Compared with conventional angiography, CT angiography seems to overestimate the stenosis.
C. Axial CT scan reveals multiple peripheral, wedge-shaped low-attenuation lesions due to hepatic infarction.
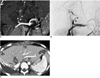
Fig. 14
Pseudoaneurysm of the hepatic artery in an 8-year-old boy, 3 years after living-related liver transplantation to treat biliary cirrhosis. Oblique axial maximum intensity projection image (A) and angiography (B) show a pseudoaneurysm (arrow) of the S3 segmental artery in the transplanted liver. We regarded this lesion as a pseudoaneurysm rather than a true aneurysm, because presurgical hepatic CT angiography of the donor was negative. The lesion probably developed as a result of a previous liver biopsy. Another saccular enhancing lesion (arrowhead) near the anastomosis site of the hepatic artery was detected and considered to be another pseudoaneurysm on CT angiography, but it was later identified as a focal varix in the right gastric vein (*, B) on the angiography. A portal vein stent is also noted on the angiography.
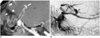
Fig. 15
Portal vein stenosis in a 2-year-old girl, 4 months after living-related liver transplantation to treat biliary atresia.
A. Oblique axial maximum intensity projection image obtained 5 days after transplantation shows an anastomosis site (arrows) of the portal vein and provides a baseline study for later comparison.
B. Oblique axial maximum intensity projection image performed 4 months later reveals the development of a severe anastomotic stenosis (arrows) of the portal vein. Based on this CT finding, a portal vein stent was placed for the treatment of the portal vein stenosis.
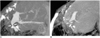
Fig. 16
Portal vein obstruction in a 7-year-old girl, 7 years after living-related liver transplantation to treat biliary atresia. Volume rendering image shows complete obstruction of the main portal vein (arrowheads). Multiple collateral vessels (*) arising from the superior mesenteric vein (SMV) reconstituted the intrahepatic portal veins (arrows). SV = splenic vein
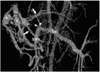
Fig. 17
Evaluation of the patency of portal vein stents.
A. Patent portal vein stent in a 9-year-old boy, 4 years after living-related liver transplantation to treat biliary cirrhosis. Curved planar reformatted image simultaneously demonstrates the patency of the proximal (*) and distal (arrows) portions of the stent.
B. Stenosis of the portal vein stent in an 1-year-old girl, 9 days after living-related liver transplantation to treat biliary atresia. Oblique axial multiplanar reformatting image shows the stenosis (arrow) of the portal vein stent. We were able to evaluate the patency of the stent, because portions of the portal vein distal to the stenosis were enhanced.
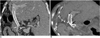
Fig. 18
Compression of the inferior vena cava caused by fluid collection in a 7-year-old boy, 18 days after living-related liver transplantation to treat biliary atresia. Oblique coronal multiplanar reformatting images show inferior vena cava compressed (arrows) by localized fluid collections (F).
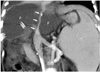
Fig. 19
Stenosis of the hepatic vein anastomosis in a 7-year-old boy, 22 days after living-related liver transplantation to treat biliary atresia.
A. Oblique coronal multiplanar reformatting image obtained 5 days after transplantation shows the anastomosis site (arrows) between the hepatic vein and the IVC and provides a baseline study for later comparison.
B. Initial postoperative Doppler sonography shows a pulsatile monophasic pattern of hepatic venous flow, which provides a baseline flow pattern of the hepatic vein for later comparison.
C. Follow-up Doppler sonography performed 22 days after transplantation reveals absence of previous pulsatility in the hepatic venous flow pattern. This sonographic finding and the observed increase in the amount of intraperitoneal fluid led to the diagnosis of hepatic vein stenosis. After confirming the significant trans-stenotic pressure gradient (14 mmHg) on catheter venography, a stent was successfully placed at the hepatic vein stenosis.
D. Oblique coronal multiplanar reformatting image after the placement of the hepatic vein stent demonstrates the patency of the placed stent (arrow).
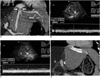
Fig. 20
Pneumobilia in a 3-year-old girl, 11 months after living-related liver transplantation to treat biliary cirrhosis. Oblique sagittal minimum intensity projection image demonstrates pneumobilia in the transplanted liver. An anastomosed site (arrow) with jejunum (J) is delineated in this patient.
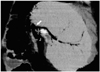
Fig. 21
Localized bile duct dilatation in a 5-year-old boy, 4 years after living-related liver transplantation to treat fulminant hepatitis. minimum intensity projection images show localized bile duct dilatations (arrows) in the transplanted liver. Secondary parenchymal atrophy of the involved segment of the transplanted liver is noted.
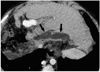
Fig. 22
Localized fluid collection - extrahepatic bile leak in a 7-year-old boy, 1 day after living-related liver transplantation to treat biliary atresia. Localized fluid collection (F) in the perihepatic space was confirmed as resulting from an extrahepatic bile leak. Hepatic venous infarction (arrows) is noted along the resection margin of the transplanted liver as a geographic low density lesion.
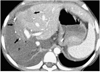
References
1. Hashikura Y, Kawasaki S, Terada M, et al. Long-term results of living-related donor liver graft transplantation: a single-center analysis of 110 transplants. Transplantation. 2001. 72:95–99.
2. Unsinn KM, Freund MC, Ellemunter H, et al. Spectrum of imaging findings after pediatric liver transplantation: part 1, posttransplantation anatomy. AJR Am J Roentgenol. 2003. 181:1133–1138.
3. Unsinn KM, Freund MC, Ellemunter H, et al. Spectrum of imaging findings after pediatric liver transplantation: part 2, posttransplantation complications. AJR Am J Roentgenol. 2003. 181:1139–1144.
4. Cheng YF, Chen CL, Jawan B, et al. Multislice computed tomography angiography in pediatric liver transplantation. Transplantation. 2003. 76:353–357.
5. Molmenti EP, Pinto PA, Klein J, Klein AS. Normal and variant arterial supply of the liver and gallbladder. Pediatr Transplant. 2003. 7:80–82.
6. Pannu HK, Maley WR, Fishman EK. Liver transplantation: preoperative CT evaluation. RadioGraphics. 2001. 21:S133–S146.
7. De Carlis L, Del Favero E, Rondinara G, et al. The role of spontaneous portosystemic shunts in the course of orthotopic liver transplantation. Transpl Int. 1992. 5:9–14.
8. Tatekawa Y, Asonuma K, Uemoto S, Inomata Y, Tanaka K. Liver transplantation for biliary atresia associated with malignant hepatic tumors. J Pediatr Surg. 2001. 36:436–439.
9. Wozney P, Zajko AB, Bron KM, Point S, Starzl TE. Vascular complications after liver transplantation: a 5-year experience. AJR Am J Roentgenol. 1986. 147:657–663.
10. Heffron TG, Pillen T, Welch D, Smallwood GA, Redd D, Romero R. Hepatic artery thrombosis in pediatric liver transplantation. Transplant Proc. 2003. 35:1447–1448.
11. Katyal S, Oliver JH 3rd, Buck DG, Federle MP. Detection of vascular complications after liver transplantation: early experience in multislice CT angiography with volume rendering. AJR Am J Roentgenol. 2000. 175:1735–1739.
12. Diem HV, Evrard V, Vinh HT, et al. Pediatric liver transplantation for biliary atresia: results of primary grafts in 328 recipients. Transplantation. 2003. 75:1692–1697.
13. Rollins NK, Sheffield EG, Andrews WS. Portal vein stenosis complicating liver transplantation in children: percutaneous transhepatic angioplasty. Radiology. 1992. 182:731–734.
14. Rossi AR, Pozniak MA, Zarvan NP. Upper inferior vena caval anastomotic stenosis in liver transplant recipients: Doppler US diagnosis. Radiology. 1993. 187:387–389.
15. Millis JM, Cronin DC, Brady LM, et al. Primary living-donor liver transplantation at the University of Chicago: technical aspects of the first 104 recipients. Ann Surg. 2000. 232:104–111.
16. Keogan MT, McDermott VG, Price SK, Low VH, Baillie J. The role of imaging in the diagnosis and management of biliary complications after liver transplantation. AJR Am J Roentgenol. 1999. 173:215–219.
17. Ametani F, Itoh K, Shibata T, Maetani Y, Tanaka K, Konishi J. Spectrum of CT findings in pediatric patients after partial liver transplantation. RadioGraphics. 2001. 21:53–63.