Abstract
In respiratory-induced proton therapy, the accuracy of tracking system and beam controlling is more important than photon therapy. Therefore, a high accuracy motion tracking system that can track internal marker and external surrogate is needed. In this research, our team has installed internal and external marker tracking system at our institution's proton therapy system, and tested the scanning with gating according to the position of marker. The results demonstrate that the developed in-house external/internal marker based gating system can be clinically used for proton therapy system for moving tumor treatment.
REFERENCES
1. Pedroni E, Bacher R, Blattmann H, Böhringer H T, Coray A, Lomax A, et al. The 200 MeV proton therapy project at PSI: Conceptual design and practical realization. Med Phys. 1995; 22:37–53.
2. Chiba T, Tokuuye K, Matsuzaki Y, Sugahara S, Chunganji Y, Kagei K, et al. Proton beam therapy for hepatocellular carcinoma: a retrospective review of 162 patients. Clin Cancer Res. 2005; 11:3799–805.


3. Chang JY, Zhang X, Wang X, Kang Y, Riley B, Bilton S, et al. Significant reduction of normal tissue dose by proton radiotherapy compared with proton radiotherapy with three-dimensional conformal or intensity modulated radiation therapy in stage I or stage III non-small cell lung cancer. Int J Radiat Oncol Biol Phys. 2006; 65:1087–96.
4. Minohara S, Kanai T, Endo M, Noda K, Kanazawa M. Respiratory gated irradiation system for heavy-ion radiotherapy. Int J Radiat Oncol Biol Phys. 2000; 47:1097–103.


5. Mori S, Chen GTY, Endo M. Effect of intrafractional motion on water equivalent path length in respiratory-gated heavy charged particle beam radiotherapy. Int J Radiat Oncol Biol Phys. 2007; 70:308–17.
6. Mori S, Yanagi T, Hara R, Sharp GC, Asakura H, Kumagai M, et al. Comparison of respiratory-gated and respiratory un-gated planning in scattered carbon ion treatment of the pancreas using four-dimensional computed tomography. Int J Radiat Oncol Biol Phys. 2010; 76:303–12.
7. Inada T, Tsujii H, Hayakawa Y, Maruhashi A, Tsujii H. Proton irradiation synchronized with respiratory cycle. Nippon Igaku Housyasen Gkkai Zasshi. 1992; 52:1161–7.
8. Kang Y, Zhang X, Chang JY, Wang H, Wei X, Liao Z, et al. 4D Proton treatment planning strategy for mobile lung tumors. Int J Radiat Oncol Biol Phys. 2007; 67:906–14.


9. Woolard A. Vicon 512 User Manual. Tustin CA: Vicon Motion Systems. 1999.
10. Cho J, Cheon W, Ahn S, Jung H, Sheen H, Park HC, et al. Development of a real-time internal and external marker tracking system for particle therapy: a phantom study using patient tumor trajectory data. J Radiat Res. 2017. 1–10.


11. Vicon Motion Systems Ltd. Bonita, UK. http://www.virtalis.com/wp-content/uploads/bonita.pdf.
Fig. 2.
The concept of finding the 3D coordinates of marker in biplane images (The two blue rectangles represent FPD's biplane images).
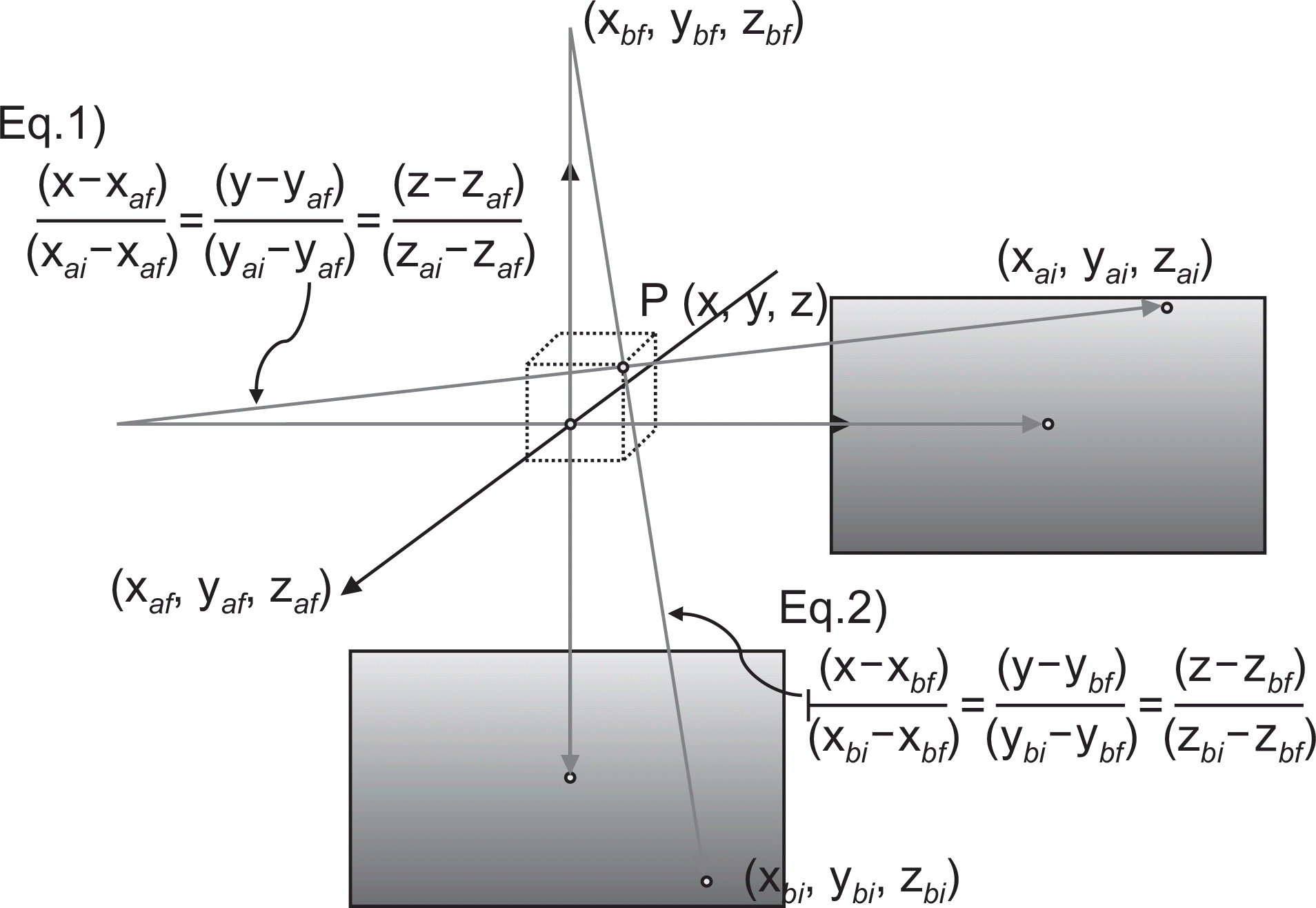
Fig. 4.
The real time output status of the ‘Co-registration Algorithm’. The boxes on the left side of the monitor show the coordinates of the three external markers, and the box on the right shows the 3D coordinates of the internal marker in millimeter scale.
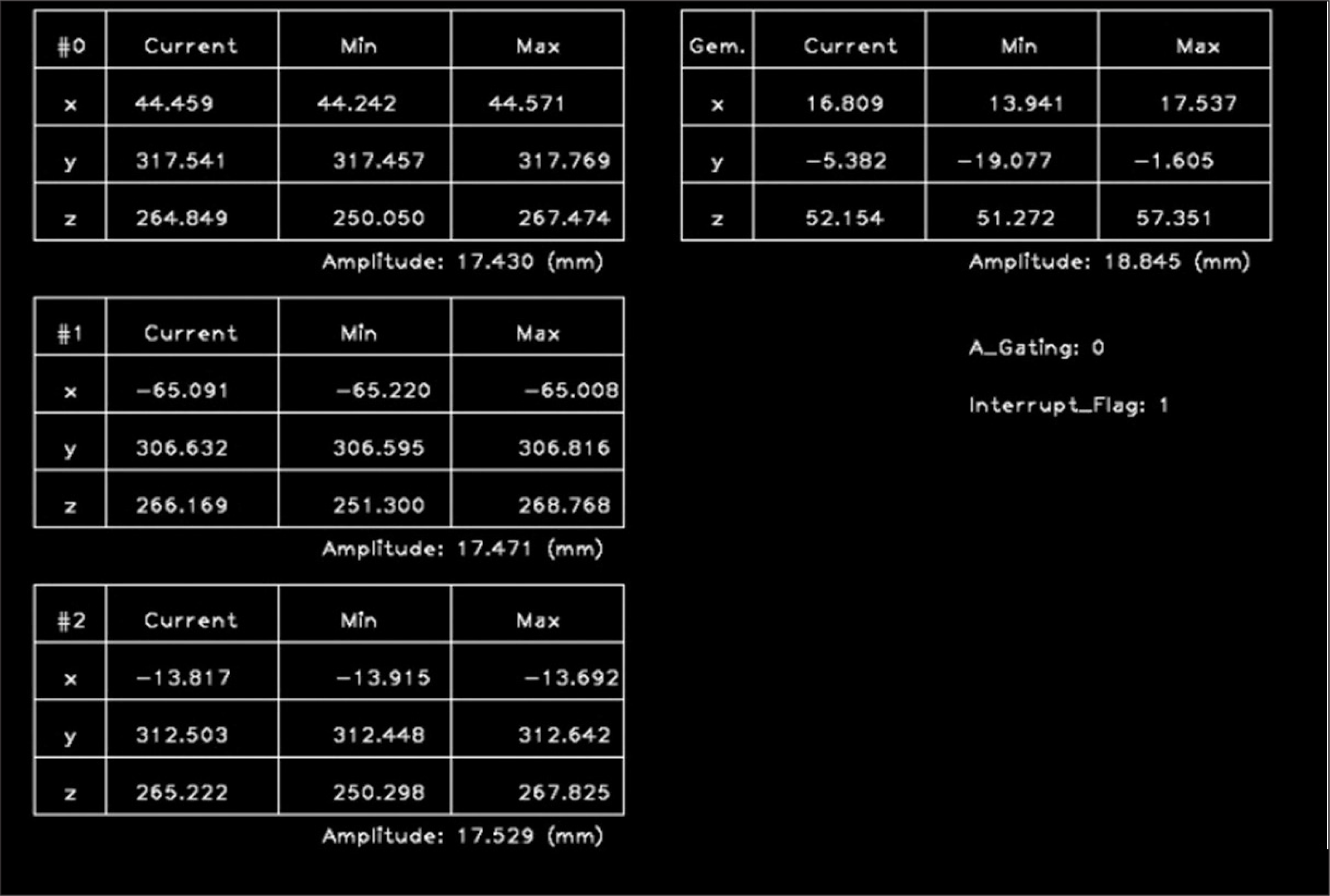