Abstract
Prior to the introduction of a medical apparatus based on heavy-ion medical accelerator in Korea, a study is needed on quality control in clinical operation for the safe and appropriate usage of the instrument. Data relevant for the study were obtained via information sharing sessions and visits by the Particle Therapy Co-Operative Group (PTCOG) and other related academic associations. Furthermore, investigative analysis of the European and Japanese performance evaluation guidelines for heavy ion, as well as research on relevant literature, were conducted. In addition, instrumental standards were analyzed through an investigation of the current usage status of the heavy-ion medical accelerator, and further analysis was conducted on the evaluation methods for the performance, safety, and significance of the instrument. Based on these analyses, regular quality control procedures for heavy-ion medical accelerators in hospitals and other institutes were extrapolated. It is hoped that the results of this study will facilitate hospitals that have introduced heavy-ion medical accelerators, or are considering the implementation of the instrument, in their understanding of the fundamental standards and capabilities of the treatment system, as well as in establishing and carrying out quality control procedures for clinical operations such that it will contribute to the safety of patients and the efficiency of medical practitioners.
REFERENCES
1. Kubota N, Suzuki M, Furusawa Y, et al. A comparison of biological effects of modulated carbon-ions and fast neutrons in human osteosarcoma cells. Int J Radiat Oncol. 1995; 33:135–41.


2. Ishizaki A, Ishii K, Kanematsu N, et al. Development of an irradiation method with lateral modulation of SOBP width using a cone-type filter for carbon ion beams. Med Phys. 2009; 36:2222–7.


3. Tessonnier T, Boehlen TT, Cerutti F, et al. Dosimetric verification in water of a Monte Carlo treatment planning tool for proton, helium, carbon and oxygen ion beams at the Heidelberg ion beam therapy center. Phys Med Biol. 2017.


4. Martisikova M, Jakel O. Dosimetric properties of Gafchromic(R) EBT films in medical carbon ion beams. Phys Med Biol. 2010; 55:5557–67.
5. Tessonnier T, Mairani A, Brons S, Haberer T, Debus J, Parodi K. Experimental dosimetric comparison of 1H, 4He, 12C and 16O scanned ion beams. Phys Med Biol. 2017; 62:3958–82.
6. Torikoshi M, Minohara S, Kanematsu N, Komori M, et al. Irradiation system for HIMAC. J Radiat Res. 2007; 48(Suppl A):A15–25.


7. Pavlovic M. Oblique gantry: An alternative for heavy-ion cancer therapy, Strahlentherapie und Onkologie: Organ der Deutschen Rontgengesellschaft. 1999; 175:Suppl. 2:24–6.
8. Gueulette J, Wambersie A. comparison of the methods of specifying carbon ion doses at NIRS and GSI. J Radiat Res. 2007; 48:Suppl A, A97-A102.


9. Lu HM, Kooy H. Optimization of current modulation function for proton spread-out Bragg peak fields. Med Phys. 2006; 33:1281–7.


10. Yeboah C, Sandison GA, Chvetsov AV. Intensity and energy modulated radiotherapy with proton beams: Variables affecting optimal prostate plan. Med Phys. 2002; 29:176–189.


11. Moreno JO, Pullia MG, Priano C, Lante V, Necchi MM, Savazzi S. Study of the magnets used for a mobile isocenter carbon ion gantry. J Radiat Res. 2013; 54:Suppl. 1:i147–54.


12. Blattmann H. Beam delivery systems for charged particles. Radiat Environ Biophys. 1992; 31:219–31.


13. Chen CC, Chang C, Moyers MF, Gao M, Mah D. Technical note: Spot characteristic stability for proton pencil beam scanning. Med Phys. 2016; 43:777–82.
14. Heeg P, Eickhoff H, Haberer T. Conception of heavy ion beam therapy at Heidelberg University (HICAT), Zeitschrift fur Medizinische Physik. 2004; 14:17–24.
15. Cao Y, Li JQ, Sun LT, et al. An all permanent magnet electron cyclotron resonance ion source for heavy ion therapy. Rev Sci Instrum. 2014; 85:02A960.


16. Muramatsu M, Hojo S, Iwata Y, et al. Development of a compact ECR ion source for various ion production. Rev Sci Instrum. 2016; 87:02C110.


17. Souda H, Yamada S, Kanai T, et al. Operation status of the electron cyclotron resonance ion source at Gunma University. Rev Sci Instrum. 2014; 85:02A934.


18. Tinschert K, Iannucci R, Lang R. Electron cyclotron resonance ion sources in use for heavy ion cancer therapy. Rev Sci Instrum. 2008; 79:02C505.


19. Winkelmann T, Cee R, Haberer T, et al. Electron cyclotron resonance ion source experience at the Heidelberg Ion Beam Therapy Center. Rev Sci Instrum. 2008; 79:02A331.


20. Schulz-Ertner D, Nikoghosyan A, Hof H, et al. Carbon ion radiotherapy of skull base chondrosarcomas. Int J Radiat Oncol. 2007; 67:171–7.


21. Ferlay J, Steliarova-Foucher E, Lortet-Tieulent J, et al. Cancer incidence and mortality patterns in Europe: eEstimates for 40 countries in 2012. Eur J Cancer. 2013; 49:1374–403.
22. Kamada T, Tsujii H, Blakely EAJ, et al. Carbon ion radiotherapy in Japan: An assessment of 20 years of clinical experience. Lancet Oncology. 2015; 16:e93–e100.


23. Tsujii H, Mizoe JE, Kamada T, et al. Overview of clinical experiences on carbon ion radiotherapy at NIRS. Radiother Oncol. 2004; 73(Suppl 2):S41–9.


24. Kamada T. Clinical evidence of particle beam therapy (carbon). Int J Radiat Oncol. 2012; 17:85–8.


25. Oh CM, Won YJ, Jung KW, et al. Community of population-based regional cancer, cancer statistics in Korea: Incidence, mortality, survival, and prevalence in 2013. Cancer Research and Treatment: Official Journal of Korean Cancer Association. 2016; 48:436–50.
26. Kamo K, Sobue T. Cancer statistics digest: Mortality trend of prostate, breast, uterus, ovary, bladder and kidney and other urinary tract cancer in Japan by birth cohort. Japanese Journal of Clinical Oncology. 2004; 34:561–3.
27. Saika S, Sobue T. [Cancer statistics in the world], Gan to kagaku ryoho. Cancer Chemother. 2013; 40:2475–80.
28. Hata M, Tokuuye K, Sugahara S, et al. Proton beam therapy for aged patients with hepatocellular carcinoma. Int J Radiat Oncol. 2007; 69:805–12.


29. Hata M, Tokuuye K, Kagei K, et al. Hypofractionated high-dose proton beam therapy for stage I non-small-cell lung cancer: preliminary results of a phase I/II clinical study. Int J Radiat Oncol. 2007; 68:786–93.


30. Yonai S. [Guideline for the handling and management of radioactive materials in ion beam radiotherapy facility], Igaku butsuri: Nihon Igaku Butsuri Gakkai kikanshi. Japanese Journal of Medical Physics. 2012; 32:81–5.
31. Sheehan M, Timlin C, Peach K, et al. Position statement on ethics, equipoise and research on charged particle radiation therapy. J Med Ethics. 2014; 40:572–5.


32. Durante M, Orecchia R, Loeffler JS. Charged-particle therapy in cancer: Clinical uses and future perspectives. Nat Rev Clin Oncol. 2017.


33. Ui-Jung Hwang YGL, Kim DW, Shin DO, et al. Study on staffing of medical physicist in the field of radiation therapy. Progress in Medical Physics. 2012; 23:10.
34. Klein EE. A grid to facilitate physics staffing justification. J Appl Clin Med Phys. 2009; 11:2987.


35. Van Loon R. The role and contribution of a medical physicist in a radiology department. Journal belge de radiologie. 1997; 80:12–6.
36. Mills MD. Analysis and practical use: The Abt Study of medical physicist work values for radiation oncology physics services, round II. Journal of the American College of Radiology. 2005; 2:782–9.
37. Kayama T, Nemoto K. [Current Status and Future Prospects of Charged Particle Radiation Therapy]. No shinkei geka: Neurological Surgery 44;2016. p. 449–54.
Fig. 1.
Organizational chart for safe operation of radiation therapy system. Top-most box: Chief of heavy-ion medical treatment center, Second row – left: radiation oncology specialist, Second row – right: Medical practitioner (quality control personnel), Third row – left: Nurse, Third row – center: radiologic technician, Third row – right: Technician team (Engineers).
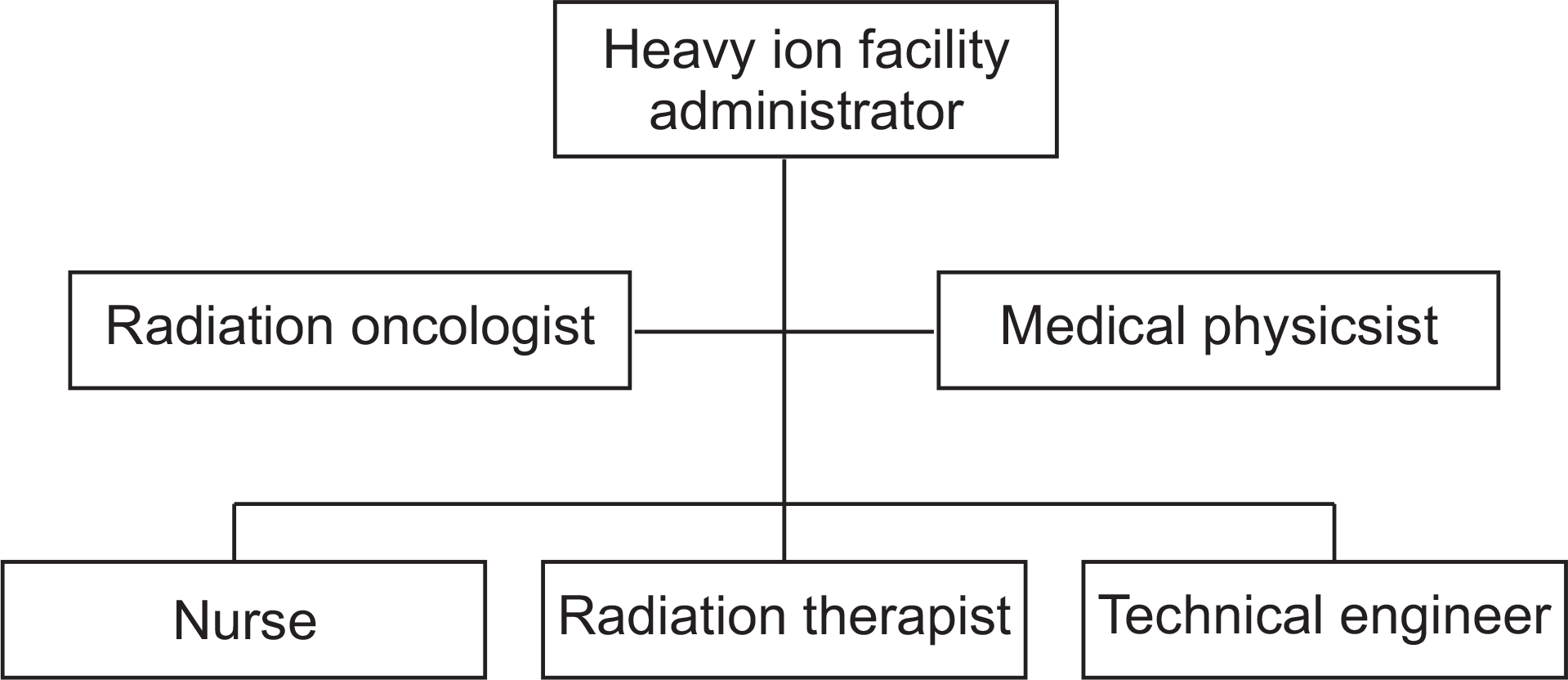
Table 1.
International/domestic trends in of heavy-ion medical accelerator usage (as of Dec 2014).
Table 2.
Capabilities of key heavy-ion medical accelerators currently operational.
Table 3.
Key capabilities of radiation therapy equipments based on heavy-ion medical accelerator.
Table 4.
Physical properties of radiation therapy treatment equipment based on heavy-ion medical accelerator.