Abstract
Chemical Exchange Saturation Transfer (CEST) imaging is a method to detect solutes based on the chemical exchange of mobile protons with water. The solute protons exchange with three different patterns, which are fast, slow, and intermediate rates. The CEST contrast can be obtained from the exchangeable protons, which are hydroxyl protons, amine protons, and amide protons. The CEST MR imaging is useful to evaluate tumors, strokes, and other diseases. The purpose of this study is to review the mathematical model for CEST imaging and for measurement of the chemical exchange rate, and to measure the chemical exchange rate using a 3T MRI system on several amino acids. We reviewed the mathematical models for the proton exchange. Several physical models are proposed to demonstrate a two-pool, three-pool, and four-pool models. The CEST signals are also evaluated by taking account of the exchange rate, pH and the saturation efficiency. Although researchers have used most commonly in the calculation of CEST asymmetry, a quantitative analysis is also developed by using Lorentzian fitting. The chemical exchange rate was measured in the phantoms made of asparagine (Asn), glutamate (Glu), γ-aminobutyric acid (GABA), glycine (Gly), and myoinositol (MI). The experiment was performed at a 3T human MRI system with three different acidity conditions (pH 5.6, 6.2, and 7.4) at a concentration of 50 mM. To identify the chemical exchange rate, the “lsqcurvefit” built-in function in MATLAB was used to fit the pseudo-first exchange rate model. The pseudo-first exchange rate of Asn and Gly was increased with decreasing acidity. In the case of GABA, the largest result was observed at pH 6.2. For Glu, the results at pH 5.6 and 6.2 did not show a significant difference, and the results at pH 7.4 were almost zero. For MI, there was no significant difference at pH 5.6 or 7.4, however, the results at pH 6.2 were smaller than at the other pH values. For the experiment at 3T, we were only able to apply 1 s as the maximum saturation duration due to the limitations of the MRI system. The measurement of the chemical exchange rate was limited in a clinical 3T MRI system because of a hardware limitation.
REFERENCES
1.Guivel-Scharen V.., Sinnwell T.., Wolff S.D.., Balaban R.S.". Detection of proton chemical exchange between metabolites and water in biological tissues. ,". J Magn Reson. 133:36–45. 1998.


2.Ward K.M.., Aletras A.H.., Balaban R.S.". A new class of contrast agents for MRI based on proton chemical exchange dependent saturation transfer (CEST). ,". J Magn Reson. 143:79–87. 2000.


3.Millet O.., Loria J.P.., Kroenke C.D.., Pons M.., Palmer A.G.". The Static Magnetic Field Dependence of Chemical Exchange Linebroadening Defines the NMR Chemical Shift Time Scale. ,". Journal of the American Chemical Society. 122:2867–2877. 2000.


4.Spencer R.G.., Fishbein K.W.". Measurement of spin-lattice relaxation times and concentrations in systems with chemical exchange using the one-pulse sequence: breakdown of the Ernst model for partial saturation in nuclear magnetic resonance spectroscopy,. ". J Magn Reson. 142:120–135. 2000.


5.van Zijl P.C.., Jones C.K.., Ren J.., Malloy C.R.., Sherry A.D.". MRI detection of glycogen in vivo by using chemical exchange saturation transfer imaging (glycoCEST),. ". Proc Natl Acad Sci U S A. 104:4359–4364. 2007.


6.Ling W.., Regatte R.R.., Navon G.., Jerschow A.". Assessment of glycosaminoglycan concentration in vivo by chemical exchange-dependent saturation transfer (gagCEST),. ". Proc Natl Acad Sci U S A. 105:2266–2270. 2008.


7.Walker-Samuel S.., Ramasawmy R.., Torrealdea F.., Rega M.., Rajkumar V.., Johnson S.P.., Richardson S.., Goncalves M.., Parkes H.G.., Arstad E.., Thomas D.L.., Pedley R.B.., Lythgoe M.F.., Golay X.". In vivo imaging of glucose uptake and metabolism in tumors,. ". Nat Med. 19:1067–1072. 2013.


8.Haris M.., Cai K.., Singh A.., Hariharan H.., Reddy R.". In vivo mapping of brain myo-inositol,. ". Neuroimage. 54:2079–2085. 2011.


9.Oh J.-H.., Kim H.-G.., Woo D.-C.., Jeong H.-K.., Lee S.Y.., Jahng G.-H.". Chemical-exchange-saturation-transfer magnetic resonance imaging to map gamma-aminobutyric acid, glutamate, myoinositol, glycine, and asparagine: Phantom experiments,. ". Journal of the Korean Physical Society. 70:545–553. 2017.


10.Haris M.., Nath K.., Cai K.., Singh A.., Crescenzi R.., Kogan F.., Verma G.., Reddy S.., Hariharan H.., Melhem E.R.., Reddy R.". Imaging of glutamate neurotransmitter alterations in Alzheimer's disease,. ". NMR Biomed. 26:386–391. 2013.


11.Yan G.., Zhang T.., Dai Z.., Yi M.., Jia Y.., Nie T.., Zhang H.., Xiao G.., Wu R.". A Potential Magnetic Resonance Imaging Technique Based on Chemical Exchange Saturation Transfer for In Vivo gamma-Aminobutyric Acid Imaging,. ". PLoS One 11, e0163765. 2016.
12.Kogan F.., Haris M.., Singh A.., Cai K.., Debrosse C.., Nanga R.P.., Hariharan H.., Reddy R.". Method for high-resolution imaging of creatine in vivo using chemical exchange saturation transfer,. ". Magn Reson Med. 71:164–172. 2014.


13.van Zijl P.C.., Zhou J.., Mori N.., Payen J.F.., Wilson D.., Mori S.". Mechanism of magnetization transfer during on-resonance water saturation. A new approach to detect mobile proteins, peptides, and lipids," Magn Reson Med. 49:440–449. 2003.
14.Zhou J.., Payen J.F.., Wilson D.A.., Traystman R.J.., van Zijl P.C.". Using the amide proton signals of intracellular proteins and peptides to detect pH effects in MRI,. ". Nat Med. 9:1085–1090. 2003.


15.McConnell H.M.". Reaction Rates by Nuclear Magnetic Resonance. ,". The Journal of Chemical Physics. 28:430–431. 1958.


16.Zhou J.., Wilson D.A.., Sun P.Z.., Klaus J.A.., Van Zijl P.C.". Quantitative description of proton exchange processes between water and endogenous and exogenous agents for WEX, CEST, and APT experiments,. ". Magn Reson Med. 51:945–952. 2004.


17.Zhou J.., van zijl P.Chemical exchange saturation transfer imaging and spectroscopy. 2006.
18.Baguet E.., Roby C.". Fast Inversion-Recovery Measurements in the Presence of a Saturating Field for a Two-Spin System in Chemical Exchange,. ". Journal of Magnetic Resonance, Series A. 108:189–195. 1994.


19.Baguet E.., Roby C.". Off-resonance irradiation effect in steady-state NMR saturation transfer,. ". J Magn Reson. 128:149–160. 1997.


20.Sun P.Z.., van Zijl P.C.., Zhou J.". Optimization of the irradiation power in chemical exchange dependent saturation transfer experiments,. ". J Magn Reson. 175:193–200. 2005.


21.Woessner D.E.., Zhang S.., Merritt M.E.., Sherry A.D.". Numerical solution of the Bloch equations provides insights into the optimum design of PARACEST agents for MRI,. ". Magn Reson Med. 53:790–799. 2005.


22.Desmond K.L.., Stanisz G.J.". Understanding quantitative pulsed CEST in the presence of MT,. ". Magn Reson Med. 67:979–990. 2012.


23.Li A.X.., Hudson R.H.., Barrett J.W.., Jones C.K.., Pasternak S.H.., Bartha R.". Four-pool modeling of proton exchange processes in biological systems in the presence of MRI-paramagnetic chemical exchange saturation transfer (PARACEST) agents,. ". Magn Reson Med. 60:1197–1206. 2008.


24.McMahon M.T.., Gilad A.A.., Zhou J.., Sun P.Z.., Bulte J.W.., van Zijl P.C.". Quantifying exchange rates in chemical exchange saturation transfer agents using the saturation time and saturation power dependencies of the magnetization transfer effect on the magnetic resonance imaging signal (QUEST and QUESP): Ph calibration for poly-L-lysine and a starburst dendrimer,. ". Magn Reson Med. 55:836–847. 2006.


25.van Zijl P.C.., Yadav N.N.". Chemical exchange saturation transfer (CEST): what is in a name and what isn't?,. ". Magn Reson Med. 65:927–948. 2011.


26.Ward K.M.., Balaban R.S.". Determination of pH using water protons and chemical exchange dependent saturation transfer (CEST),. ". Magn Reson Med. 44:799–802. 2000.


27.Zong X.., Wang P.., Kim S.G.., Jin T.". Sensitivity and source of amine-proton exchange and amide-proton transfer magnetic resonance imaging in cerebral ischemia,. ". Magn Reson Med. 71:118–132. 2014.


28.McVicar N.., Li A.X.., Goncalves D.F.., Bellyou M.., Meakin S.O.., Prado M.A.., Bartha R.". Quantitative tissue pH measurement during cerebral ischemia using amine and amide concentration-independent detection (AACID) with MRI,. ". J Cereb Blood Flow Metab. 34:690–698. 2014.


29.Zaiss M.., Schmitt B.., Bachert P.". Quantitative separation of CEST effect from magnetization transfer and spillover effects by Lorentzian-line-fit analysis of z-spectra,. ". J Magn Reson. 211:149–155. 2011.
30.Goerke S.., Zaiss M.., Kunz P.., Klika K.D.., Windschuh J.D.., Mogk A.., Bukau B.., Ladd M.E.., Bachert P.". Signature of protein unfolding in chemical exchange saturation transfer imaging,. ". NMR Biomed. 28:906–913. 2015.


31.Desmond K.L.., Moosvi F.., Stanisz G.J.". Mapping of amide, amine, and aliphatic peaks in the CEST spectra of murine xenografts at 7 T,. ". Magn Reson Med. 71:1841–1853. 2014.


32.Liu D.., Zhou J.., Xue R.., Zuo Z.., An J.., Wang D.J.". Quantitative characterization of nuclear overhauser enhancement and amide proton transfer effects in the human brain at 7 tesla,. ". Magn Reson Med. 70:1070–1081. 2013.


33.Bryant R.G.". The dynamics of water-protein interactions,. ". Annu Rev Biophys Biomol Struct. 25:29–53. 1996.


34.Zaiss M.., Bachert P.". Chemical exchange saturation transfer (CEST) and MR Z-spectroscopy in vivo: a review of theoretical approaches and methods,. ". Phys Med Biol. 58:R221–269. 2013.
Fig. 1
Results of the exchange rate experiments with different pH values for asparagine (a), glutamate (b), GABA (c), glycine (d), and myoinositol (e) at 3T. The B1 amplitude was 3 μT and the concentration was 50 mM. Data points with different saturation durations are shown with a circle (pH 5.6), a cross (pH 6.2), and a triangle (pH 7.4). Results were plotted with a solid line at pH 5.6, a dashed line at pH 6.2, and a dotted line at pH 7.4.
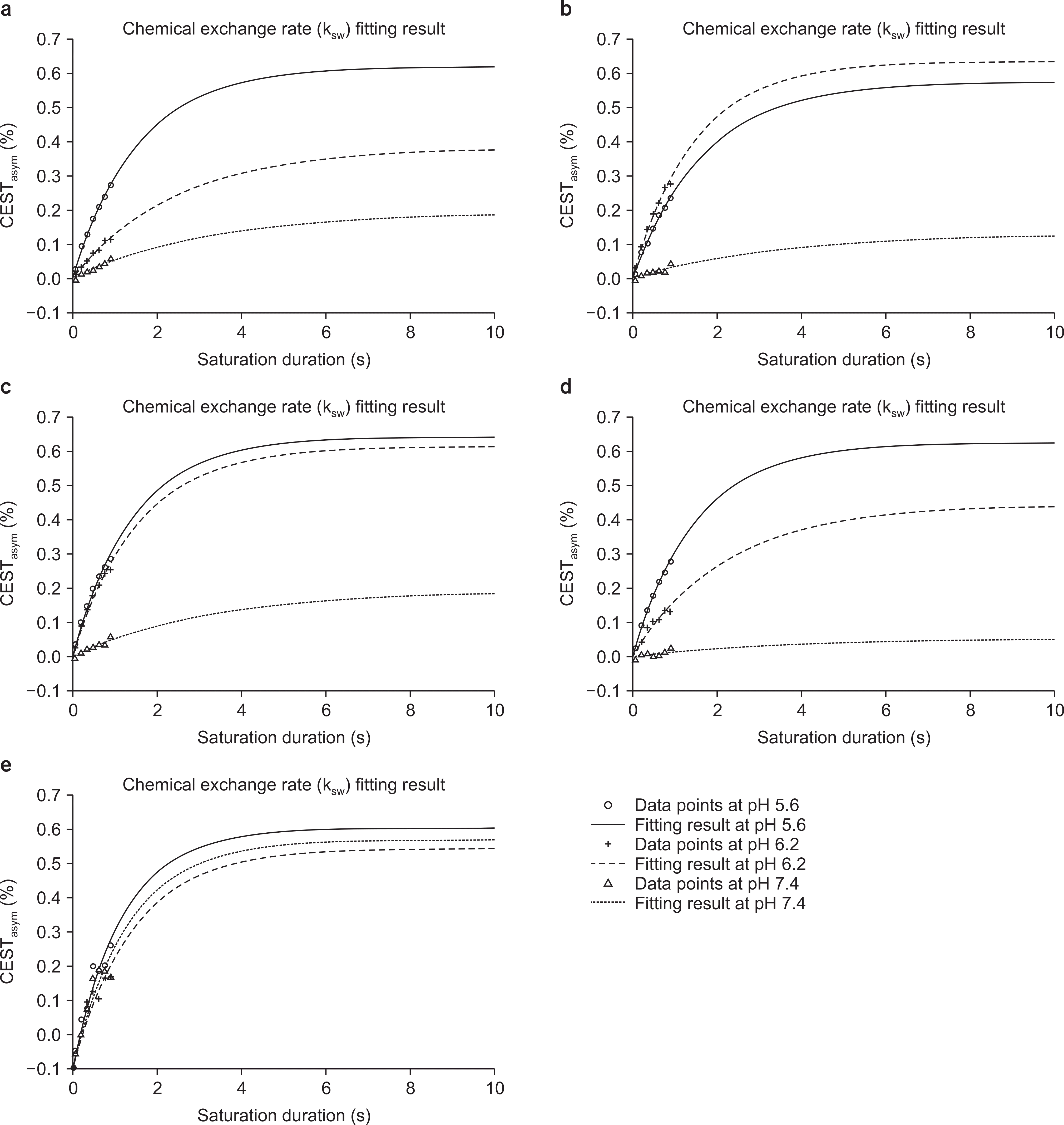
Table 1.
Initial values, the lower limit, and the upper limit of the amplitude, the full width of half maximum (FWHM), and the center frequency of the six pools for Lorentzian curve fitting.
The amplitude is no unit because of the normalization of the full Z spectrum signals by the reference signal. The frequency (ppm) is the center frequency of the exchangeable protons. The six pool protons were defined in the initial offset frequency values as water at 0 ppm, amide at 3.5 ppm, amine at 3.0 ppm, hydroxyl at 0.9 ppm, the nuclear overhauser effect (NOE) at −3.5 ppm, and the magnetization transfer (MT) at −1.5ppm.