Abstract
Proton therapy is increasingly being actively used in the treatment of cancer. In contrast to photons, protons have the potential advantage of delivering higher doses to the cancerous tissue and lower doses to the surrounding normal tissue. However, a range shifter is needed to degrade the beam energy in order to apply the pencil beam scanning technique to tumors located close to the minimum range. The secondary neutrons are produced in the beam path including within the patient's body as a result of nuclear interactions. Therefore, unintended side effects may possibly occur. The research related to the secondary neutrons generated during proton therapy has been presented in a variety of studies worldwide, since 2007. In this study, we measured the magnitude of the secondary neutron dose depending on the location of the detector and the use of a range shifter at the beam nozzle of the proton scanning mode, which was recently installed. In addition, the production of secondary neutrons was measured and estimated as a function of the distance between the isocenter and detector. The neutron dose was measured using WENDI-II (Wide Energy Neutron Detection Instruments) and a Plastic Water phantom; a Zebra dosimeter and 4-cm-thick range shifter were also employed as a phantom. In conclusion, we need to consider the secondary neutron dose at proton scanning facilities to employ the range shifter reasonably and effectively.
REFERENCES
2.Olsen DR, et al. Proton therapy –A systematic review of clinical effectiveness. Radiother Oncol 83: 123. 2007.
3.Particle Therapy Co-Operative Group. Particle therapy facilities under construction [Internet]. [place un known]: Particle Therapy Co-Operative Group; Available from:. http://www.ptcog.ch/index.php/facilities-under-construction.
4.Shin JS, et al. Analysis of changes in dose distribution due to respiration during IMRT. Radiat Oncol J 29: 206. 2011.


5.Schneider U., Lomax A., Lombriser N. Comparative risk assessment of secondary cancer incidence after treatment of Hodgkin's disease with photon and proton radiation. Radiat Res. 154(4):): 382–388. 2000.


6.Paganetti H. Nuclear interactions in proton therapy: Dose and relative biological effect distributions originating from primary and secondary particles. Phys Med Biol. 47:747–764. 2002.


7.Zacharatou JC., Paganetti H. Risk of developing second cancer from neutron dose in proton therapy as function of field characteristics: organ and patient age. Int J Radiat Oncol Biol Phys. 82:228–35. 2008.
8.Kim JS, et al. Feasibility study of neutron dose for real-time image-guided proton therapy: a Monte Carlo study. JKPS. 67(1):142–146. 2015.
9.Yonai S, et al. Measurement of neutron ambient dose equivalent in passive carbon-ion and proton radiotherapies. Med Phys. 35:4782–4792. 2008.


10.Hall EJ. Intensity modulated radiation therapy, protons, and the risk of second cancers Int J Radiat Oncol Biol Phys. 65:1–7. 2006.
11.Chung KZ, et al. The first private-hospital based proton therapy center in Korea; Status of the Proton Therapy Center at Samsung Medical Center. Radiat Oncol J. 33(4):1–7. 2015. . DOI: doi: 10.3857/roj.2015.33.4.337.


12.https://www.thermofisher.com/order/catalog/product/FHT762WENDI2.
13.ICRP. 1977. Recommendations of the ICRP. ICRP Publication 26. Ann. ICRP. 1(3):
Fig. 5
Two-dimensional dosage distribution within phantom irradiated by four proton scanning methods to assess neutron dose of range shifter.
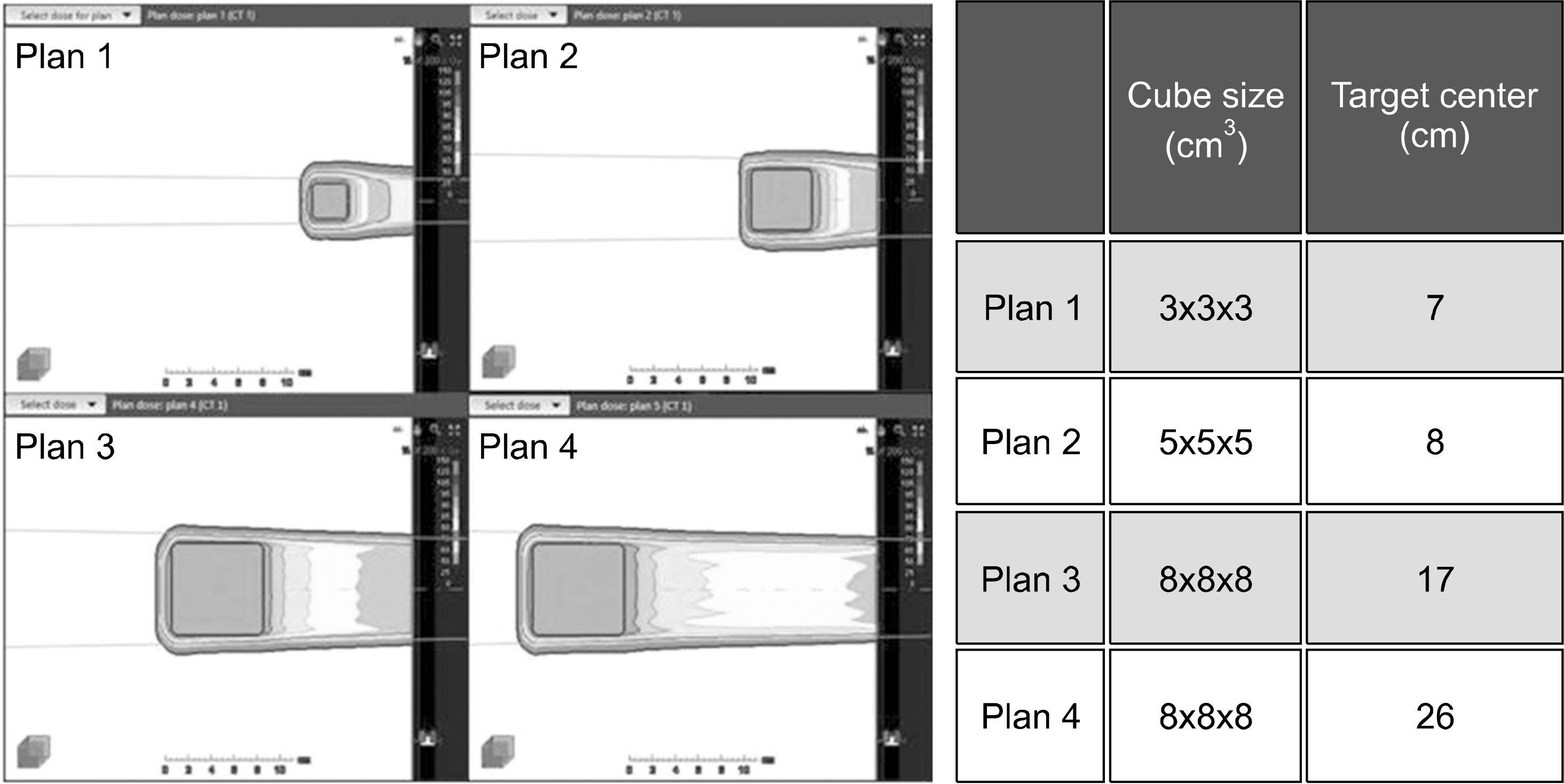
Fig. 6
Measurement of secondary neutron using WENDI-II. Upper left and right: neutron emission measurements taken with a range shifter; lower left: emission measurement taken without a range shifter; lower right: emission measurements taken from a phantom.
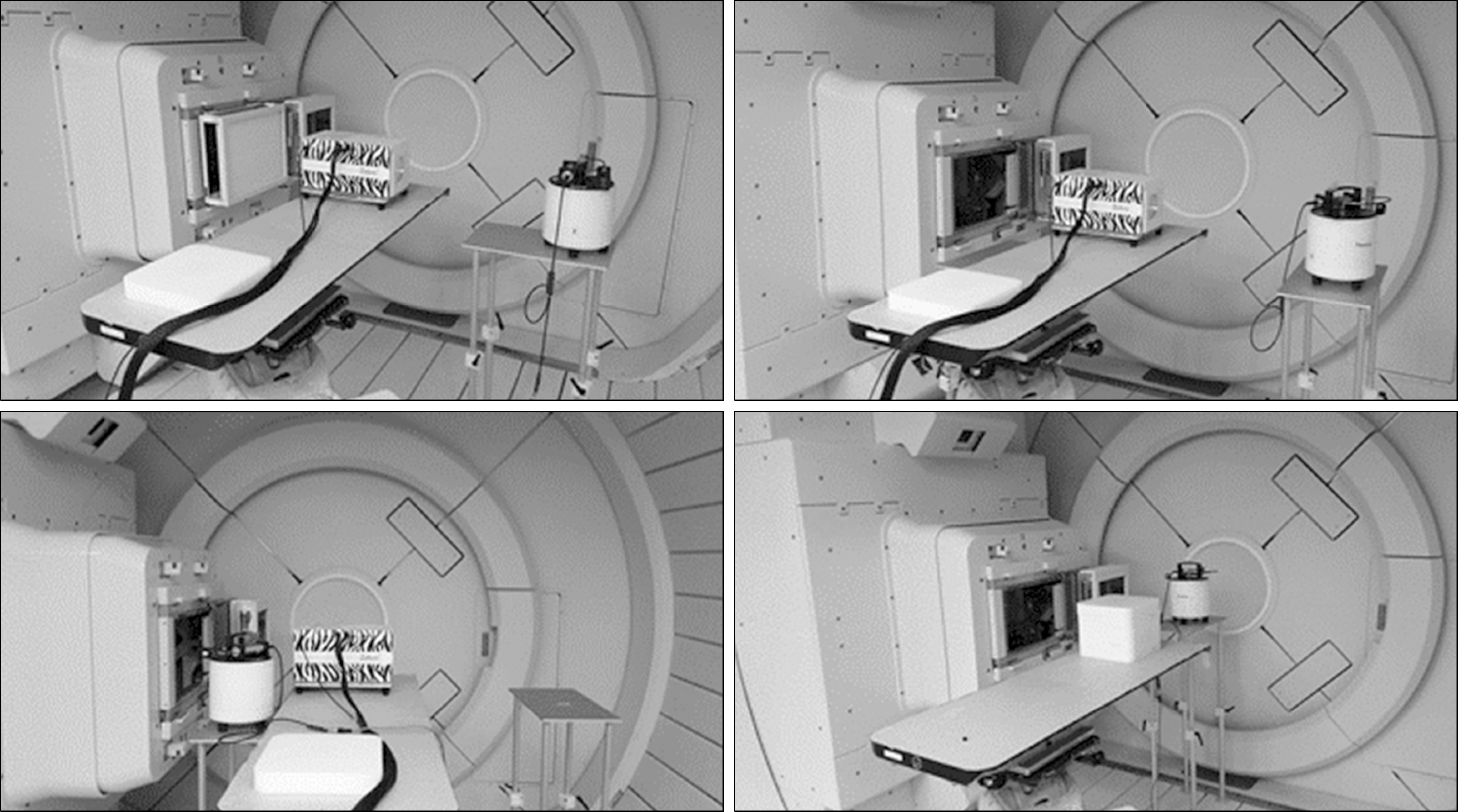
Table 1.
WENDI-II technical specification.
Table 2.
Secondary neutron dose of a plastic water phantom at varying distance.
Table 3.
Secondary neutron dose of dedicated scanning nozzle using range shifter position 1.
Table 4.
Secondary neutron dose of dedicated scanning nozzle using range shifter position 2.