Abstract
Since a Compton camera has high detection sensitivity due to electronic collimation and a good energy resolution, it is a potential imaging system for nuclear medicine. In this study, we investigated the feasibility of a Compton camera for multi-tracer imaging and proposed a rotating Compton camera to satisfy Orlov's condition for 3D imaging. Two software phantoms of 140 and 511 keV radiation sources were used for Monte-Carlo simulation and then the simulation data were reconstructed by listmode ordered subset expectation maximization to evaluate the capability of multi-tracer imaging in a Compton camera. And the Compton camera rotating around the object was proposed and tested with different rotation angle steps for improving the limited coverage of the fixed conventional Compton camera over the field-of-view in terms of histogram of angles in spherical coordinates. The simulation data showed the separate 140 and 511 keV images from simultaneous multi-tracer detection in both 2D and 3D imaging and the number of valid projection lines on the conical surfaces was inversely proportional to the decrease of rotation angle. Considering computation load and proper number of projection lines on the conical surface, the rotation angle of 30 degree was sufficient for 3D imaging of the Compton camera in terms of 26 min of computation time and 5 million of detected event number and the increased detection time can be solved with multiple Compton camera system. The Compton camera proposed in this study can be effective system for multi-tracer imaging and is a potential system for development of various disease diagnosis and therapy approaches.
References
1. Gambhir SS, Barrio JR, Herschman HR, Phelps ME. Assays for noninvasive imaging of reporter gene expression. Nucl Med Biol. 26:481–490. 1999.


2. Phelps ME. Nuclear medicine, molecular imaging, and molecular medicine. J Nucl Med. 43:13N–14N. 2002.
3. Rudin M, Weissleder R. Molecular imaging in drug discovery and development. Nat Rev Drug Discov. 2:123–131. 2003.


4. Cherry S. In vivo molecular and genomic imaging: new challenges for imaging physics. Phys Med Biol. 49:R13–R48. 2004.
5. Fullerton GD, Hazle JD. The development of technologies for molecular imaging should be driven principally by biological questions to be addressed rather than by simply modifying existing imaging technologies. For the proposition. Med Phys. 32:1231–1233. 2005.
7. Cherry SR, Sorenson JA, Phelps ME. Physics in Nuclear Medicine. 3rd ed.;Saunders;2003.
8. Phelps ME. PET – molecular imaging and its biological applications. Springer;New York: 2004.
9. Bailey DL, Townsend DW, Valk PE, Maisey MN. Positron emission tomography – basic sciences. Springer Longdon;2005.
10. Todd RW, Nightingale JM, Everett DB. A proposed Gamma camera. Nature. 251:132–134. 1974.
11. Singh M. An electronically collimated gamma camera for single photon emission computed tomography: Part1 and 2. Med Phys. 10:421–427. 1983.
13. Yang YF, Gono Y, Motomura S, Enomoto S, Yano Y. A Compton camera for multitracer imaging. IEEE Trans Nucl Sci. 48:656–661. 2001.


14. Motomura S, Kanayama Y, Haba H, Watanabe Y, Enomoto S. Multiple molecular simultaneous imaging in a live mouse using semiconductor Compton camera. J Anal AT Spectrom. 23:1089–1092. 2008.


15. Motomura S, Fukuchi T, Kanayama Y, Haba H, Watanabe Y, Enomoto S. Three-dimensional tomographic imaging by semiconductor Compton camera GREI for multiple molecular simultaneous imaging. Nucl Sci Symp Conf Rec.2009. Orlando, FL, USA, pp.3330–3332.
16. Seo H, Kim CH, Park JH, et al. Multitracing capability of double-scattering Compton imager with NaI(Tl) scintillator absorber. IEEE Trans Nucl Sci. 57:1420–1425. 2010.


17. Uche CZ, Round WH, Cree MJ. Evaluation of detector material and radiation source position on Compton camera's ability for multitracer imaging. Australas Phys Eng Sci Med. 35:357–364. 2012.


18. Motomura S, Kanayama Y, Hiromura M, et al. Improved imaging performance of a semiconductor Compton camera GREI makes for a new methodology to integrate bio-metal analysis and molecular imaging technology in living organisms. J Anal At Spectrom. 28:934–939. 2013.


19. Lee SH, Park JH, Park SH, et al. CIS – a GUI-based software system for Monte Carlo simulation of Compton camera. Nucl Technol. 168:55–60. 2009.
20. Wilderman SJ, Rogers WL, Knoll GF, Engdahl JC. Fast algorithm for list mode back-projection of Compton scatter camera data. IEEE Trans Nucl Sci. 45:957–962. 1998.


21. Kim SM, Lee JS, Lee CS, et al. Fully three-dimensional OSEM-based image reconstruction for Compton imaging using optimized ordering schemes. Phys Med Biol. 55:5007–5027. 2010.


22. Orlov SS. Theory of three-dimensional reconstruction. 1. Conditions for a complete set of projections. Sov Phys-Crystallogr. 20:312–314. 1975.
23. Nguyen V-G, Lee S-J, Lee MN. GPU-accelerated 3D Bayesian image reconstruction from Compton scattered data. Phys Med Biol. 56:2817–2836. 2011.


24. Lowe VJ, Greer KL, Hanson MW, Jaszczak RJ, Coleman RE. Cardiac phantom evaluation of simultaneously acquired dual-isotope rest thallium-201/stress technetium-99m SPECT images. J Nucl Med. 34:1998–2006. 1993.
25. Berman DS, Kiat H, Friedman JD, et al. Separate acquisition rest thallium-201/stress technetium-99m sestamibi dual-isotope myocardial perfusion single-photon emission computed tomography: a clinical validation study. J American College of Cardio. 22:1455–1464. 1993.


26. Cao ZJ, Chen CC, Maunoury C, Holder LE, Abraham TC, Tehan A. Phantom evaluation of simultaneous thallium-201/technetium-99m aquisition in single-photon emission tomography. European J Nucl Med. 23:1514–1520. 1996.


27. Siebelink HM, Natale D, Sinusas AJ, Wackers F. Quantitative comparison of single-isotope and dual-isotope stressrest single-photon emission computed tomographic imaging for reversibility of defects. J Nucl Cardio. 3:483–493. 1996.


28. Hannequin P, Weinmann P, Mas J, Vinot S. Preliminary clinical results of photon energy recovery in simultaneous rest TI-201/stress Tc-99m sestamibi myocardial SPECT. J Nucl Cardio. 8:144–151. 2001.
29. Segall G. Assessment of myocardial viability by positron emission tomography. Nucl Med Commun. 23:323–330. 2002.


Fig. 1.
Two-dimensional circular disk software phantom containing two radiation sources of 140 and 511 keV energies for multi-tracer imaging simulation.
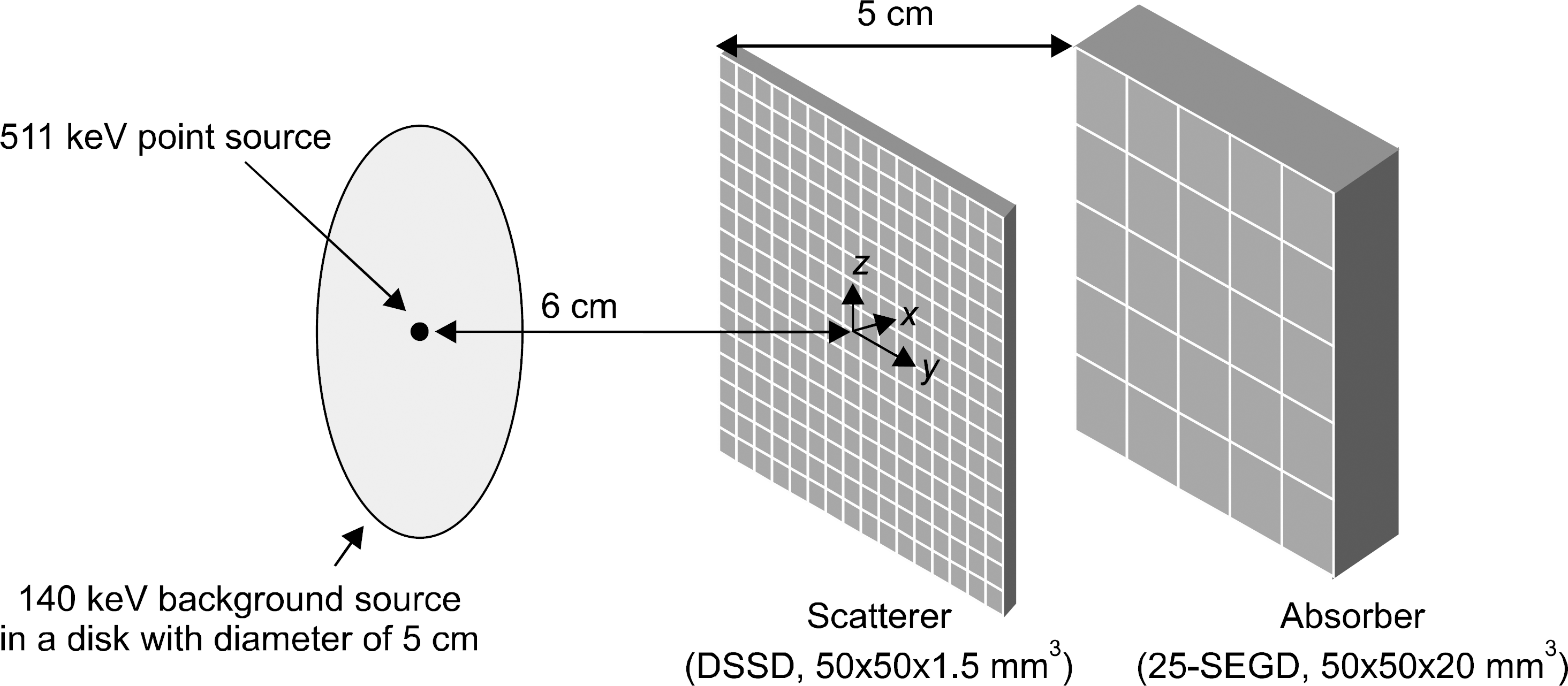
Fig. 2.
Three-dimensional cylindrical software phantom and 4 spheres (dia.=3.2, 4.2, 5.4, 7.0 mm) containing two radiation sources of 140 and 511 keV energies for multi-tracer imaging simulation.
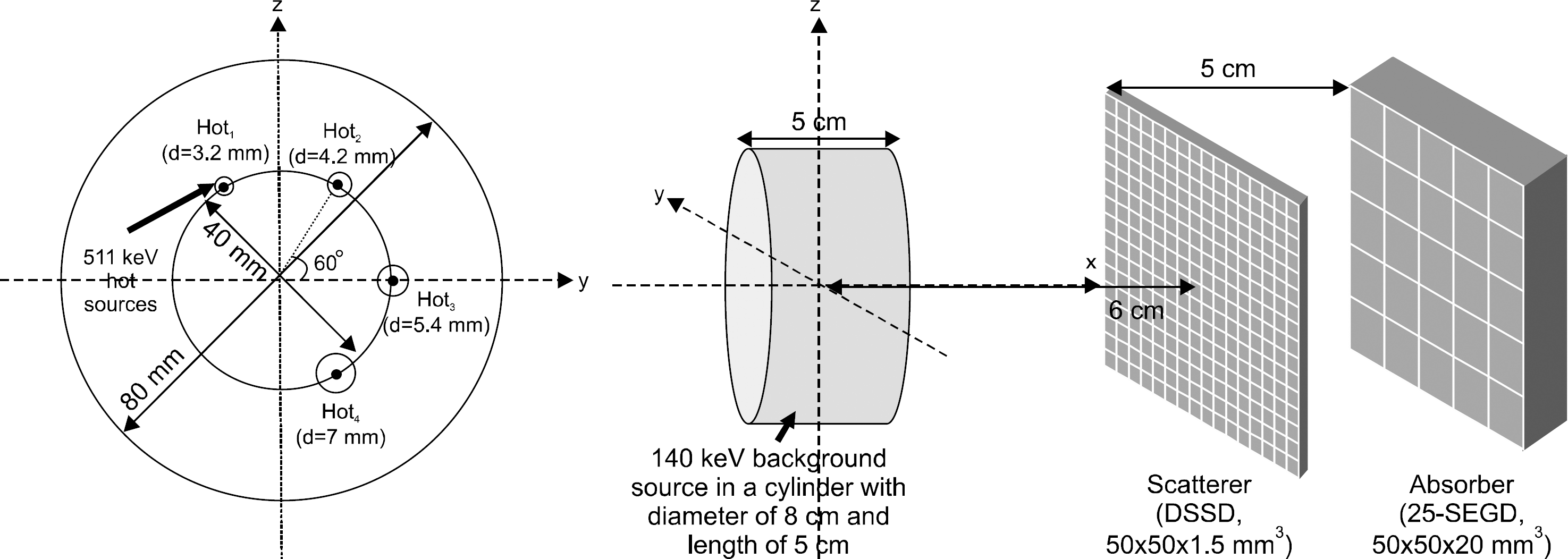
Fig. 4.
Sampling technique of lines on conical surface defined with two detection positions and a scattering angle and the corresponding angles and ϕ of the sampled lines in spherical coordinate.
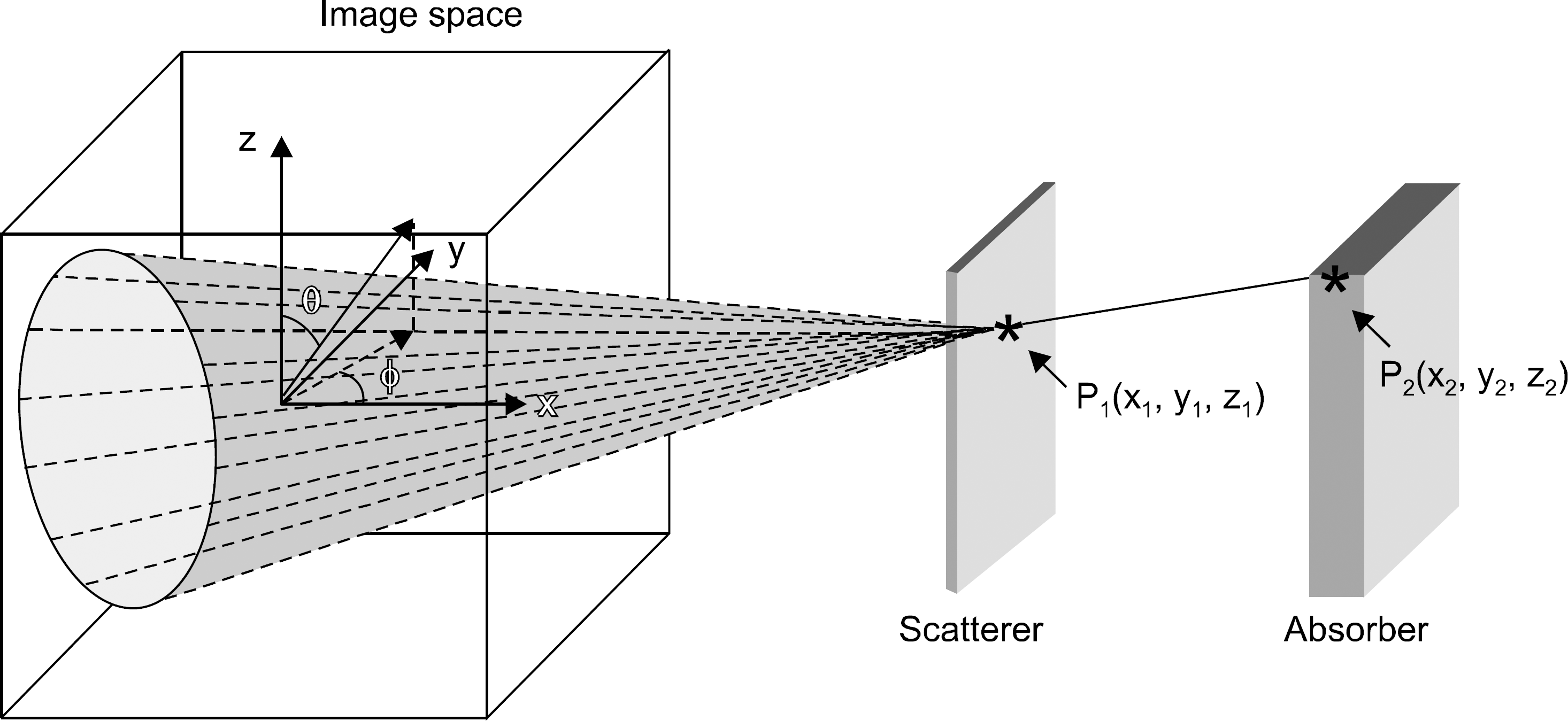
Fig. 5.
Multi-tracer images reconstructed from the Monte-Carlo simulation data of 2D circular disk of 140 keV and a point source of 511 keV: total event number of (a) 98×103 (98K) and (b) 980×103 (980K).
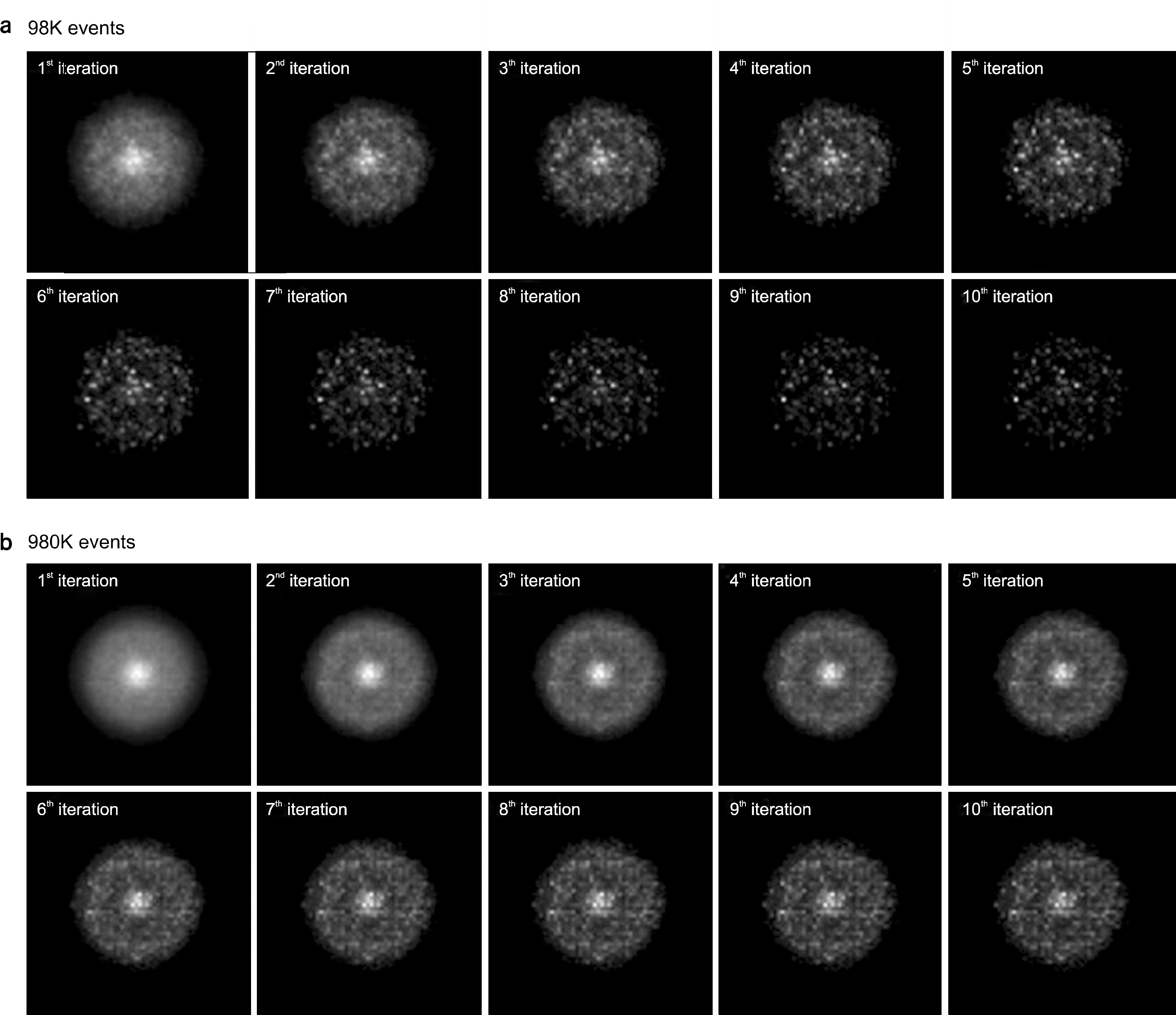
Fig. 6.
Multi-tracer images reconstructed from the Monte-Carlo simulation data of 3D cylindrical phantom: (a) four spheres of 511 keV, (b) uniform cylinder of 140 keV, (c) summed multi-tracer image of 3D cylindrical phantom (top: y-z planes, bottom: x-y planes of the reconstructed images).
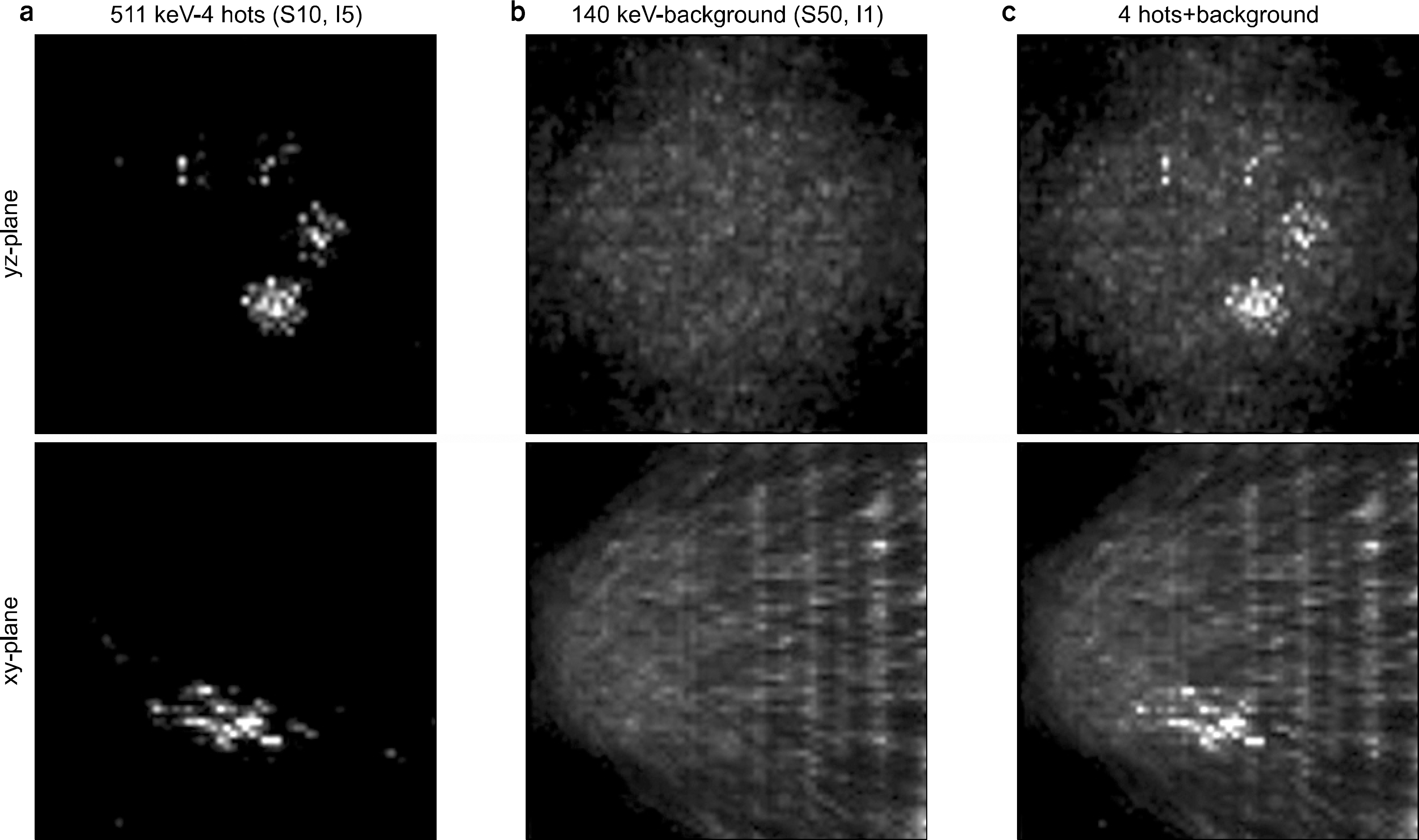