Abstract
Objective
Orthodontic root resorption (ORR) due to orthodontic tooth movement is a difficult treatment-related adverse event. Caspases are important effector molecules for apoptosis. At present, little is known about the mechanisms underlying ORR and apoptosis in the cementum. The aim of the present in vivo study was to investigate the expression of tartrate-resistant acid phosphatase (TRAP), caspase 3, caspase 8, and receptor activator of nuclear factor kappa-B ligand (RANKL) in the cementum in response to a heavy or an optimum orthodontic force.
Methods
The maxillary molars of male Wistar rats were subjected to an orthodontic force of 10 g or 50 g using a closed coil spring. The rats were sacrificed each experimental period on days 1, 3, 5, and 7 after orthodontic force application. And the rats were subjected to histopathological and immunohistochemical analyses.
Results
On day 7 for the 50-g group, hematoxylin and eosin staining revealed numerous root resorption lacunae with odontoclasts on the root, while immunohistochemistry showed increased TRAP- and RANKL-positive cells. Caspase 3- and caspase 8-positive cells were increased on the cementum surfaces in the 50-g group on days 3 and 5. Moreover, the number of caspase 3- and caspase 8-positive cells and RANKL-positive cells was significantly higher in the 50-g group than in the 10-g group.
Orthodontic root resorption (ORR) due to orthodontic tooth movement (OTM) is considered one of the most difficult treatment-related adverse events that has the potential to result in permanent root structure loss. In an epidemiological study by Kaley and Phillips,1 all patients receiving comprehensive orthodontic treatment exhibited shortened roots, with 3% showing severe root resorption (shortening of > 25% of the root length) and shortening of the maxillary central incisor roots. Previous studies have reported that the type of orthodontic appliance,2 the magnitude of force, the duration of force application,345 the type of tooth movement (reciprocating movement or “jiggling” orthodontic forces),6 the presence of local and systemic disease, age-related genetic factors, root abnormalities, a history of previous trauma, and ethnicity are associated with the severity of root resorption. The application of light orthodontic forces causes direct resorption of the alveolar bone, while the application of heavy orthodontic forces results in strong pressure that induces local ischemia, tissue healing, and cell death in the periodontal ligament (PDL).
Tumor necrosis factor alpha (TNF-α) plays an important role in the mediation of immune and inflammatory responses and the control of proliferation, differentiation, and apoptosis.7
Apoptosis refers to the process of programmed cell death. Several components are required for its execution. Recent studies involving experiments in rat teeth have suggested that osteocytes in alveolar bone adjacent to hyaline-treated PDL causes cell death through apoptosis during OTM.8 Apoptosis peaks at 3 days after the application of orthodontic forces, and factors influencing cyclin and/or cyclin-dependent kinases may cause cell cycle arrest.9 In addition, when the cycle continues while the damaged DNA in cells remains intact, an apoptotic mechanism is triggered and apoptotic cell death occurs.10
Caspases constitute a family of cysteine proteases that cleave proteins after aspartic acid residues and play a major role in apoptotic cell death. Caspases participate in the cascade as triggers of cell death, as elements regulating the process of cell death, and as a function of cell death itself. Caspases 2, 8, 9, and 10 are classified as initiator (apical) caspases, while caspases 3, 6, and 7 are classified as effector (performer) caspases. Their activation leads to characteristic morphological changes in cells, such as contraction, chromatin condensation, DNA fragmentation, and the development of plasma membrane vesicles, eventually leading to apoptosis.11 In the present study, we focused on the exogenous factor caspase 8 and the execution factor caspase 3.
The cementum, which covers the root surface, is composed of thin calcified tissue produced by cementoblasts. It was recently reported that human primary cementoblasts (HPCBs) undergo apoptosis under pressure in vitro.11 However, little is known about the relationship between root resorption during OTM and the apoptosis of cementoblasts.
We previously reported that receptor activator of nuclear factor kappa-B ligand (RANKL) is detected in resorbed roots and PDL tissues exposed to excessive orthodontic forces.12 Moreover, Hatai et al.13 suggested that apoptosis occurs on the side of alveolar bone compression during OTM. Therefore, apoptosis and RANKL expression may affect ORR during OTM.
The aim of the present in-vivo study was to investigate the expression of tartrate-resistant acid phosphatase (TRAP), caspase 3, caspase 8, and RANKL in the cementum in response to a heavy or an optimum orthodontic force.
The protocols for the animal experiments performed in the present study were approved by the Animal Experimental Ethics Committee of Matsudo School of Dentistry at Nihon University (Approval No. AP15MD006). Sixty 6-week-old male Wistar rats (body weight, 180 ± 10 g; Sankyo Labo Service, Tokyo, Japan) were randomly assigned to three groups as follows: control group, where no force was applied (n = 20); 10-g group, where an optimum force of 10 g was applied (n = 20); and 50-g group, where a heavy force of 50 g was applied (n = 20).
For fixation of the orthodontic appliances, a mixture of three anesthetic agents (medetomidine hydrochloride, 1.875 mg/kg; midazolam, 2 mg/kg; butorphenol tartrate, 2.5 mg/kg) was intraperitoneally administered with physiological saline (1.8625 mg/kg). The methods used to achieve tooth movement have been discussed by Asano et al.14 Briefly, mesial movement of the maxillary right first molar was achieved with a closed coil spring ligated to the maxillary first molar using a 0.008-inch stainless steel ligation (Tommy International, Inc., Tokyo, Japan) wire (wire size: 0.005 inch, diameter: 1/12 inch; Accurate Sales Co., Tokyo, Japan). Forces of 10 g and 50 g were applied in the 10-g and 50-g groups, respectively. The other side of the coil spring was ligated using the same ligation wire to a hole drilled with a #1/4 round bur in the maxillary incisor, just above the gingival papilla. Prepared specimens were subjected to histopathological and immunohistochemical analyses on days 1, 3, 5, and 7 after orthodontic force application (Figure 1).
The experimental periods were set at 1, 3, 5, and 7 days after tooth movement was initiated. The animals were deeply anesthetized using the above-described mixture of the three anesthetic agents (0.1 M). Immediately after transnasal perfusion with 4% paraformaldehyde, the maxilla was dissected and immersed in the same fixative at 4℃ for 18 hours. The sample was decalcified in a 10% disodium ethylenediaminetetraacetate solution (EDTA, pH 7.4) in Tris buffered saline (TBS) for 4 weeks. Then, the ashed specimen was dehydrated in a stepwise manner by a series of ethanol washes and embedded in paraffin. Each sample was sequentially sliced into 4-µm horizontal sections and prepared for hematoxylin and eosin (H&E) and immunohistochemical staining. The periodontal tissue in the mesial portion of the distobuccal roots was subjected to observations. For detailed observations, we set microscopic regions of interest measuring 300 µm in the area close to the furcation on the mesial surface of the distal buccal root, which was the side of compression during tooth movement (Figure 2). The number of positively stained cells was counted by the first author, the corresponding author, and two clinical pathologists.
Tissue sections were deparaffinized and quenched by endogenous peroxidase activity via incubation in 3% H2O2 in methanol for 30 minutes at room temperature. After washing in TBS, the sections were incubated with polyclonal anti-goat TRAP (working dilution, 1:50; Santa Cruz Biotechnology, Inc., Santa Cruz, CA, USA), polyclonal anti-rabbit caspase 3 (working dilution, 1:300; Cell Signaling Technology, Inc., Danvers, MA, USA), polyclonal anti-rabbit caspase 8 (working dilution, 1:1,000; Novus Biologicals, Inc., Littleton, CO, USA), and polyclonal anti-goat RANKL (working dilution, 1:50; Santa Cruz Biotechnology) for 18 hours at 4℃. TRAP, caspase 3, caspase 8, and RANKL were stained using the Histofine Simple Stain MAX-Po kit (multi; Nichirei, Co., Tokyo, Japan) in accordance with the manufacturer's protocol. The sections were rinsed with TBS, and the final color reactions were performed using the 3, 3′-diaminobenzidine tetrahydrochloride substrate reagent and aminoethyl carbazole. Then, the sections were counterstained with Mayer's hematoxylin. Immunohistochemistry negative tissue controls were prepared by the incubation of several sections with nonimmune rabbit immunoglobulin G or 0.01 M phosphate-buffered saline instead of the primary antibody. Negative reactivity was observed in all sections.
All values are expressed as means ± standard deviations. Mean values were compared among the three groups using Kruskal-Wallis and Steel-Dwass tests. The p-values of < 0.05 and < 0.01 were considered statistically significant. The statical analysis was performed with Excel Analysis (Seiun, Tokyo, Japan).
The body weight of the rats in the three groups showed no significant changes during the experiment, with no significant differences among the three groups (data not shown).
PDL specimens obtained from the control group (0 g) on days 1, 3, 5, and 7 showed fibroblasts that were horizontally aligned with connective tissue fibers of a higher relative density in the cementum. The root surfaces were relatively smooth, with few mononuclear and polynuclear osteoclasts and no resorption lacunae (panels a–d in Figure 3).
In the 10-g group, a coarse arrangement of fibers with dilated capillaries was observed on day 3. On days 5, the arrangement of the fibers and fibroblasts appeared coarse and irregular, while the capillaries were constricted (panels f and g in Figure 3). On day 7, several root resorption lacunae with odontoclasts were found on the root surfaces (panel h in Figure 3).
In the control group, TRAP-positive multinuclear osteoclasts were not found on the root surfaces on days 1, 3, 5, and 7; furthermore, no resorption lacunae were observed (panels a–d in Figure 4A). In the 10-g group, root resorption lacunae with TRAP-positive multinuclear osteoclasts were sparsely distributed on the root surfaces on day 7 (panel h in Figure 4A). In contrast, in the 50-g group, numerous root resorption lacunae with multinucleated TRAP-positive dentin chromosomes were observed on the root surfaces on day 7 (panel l in Figure 4A).
Quantitative evaluations revealed that the number of TRAP-positive odontoblasts on day 7 was significantly higher in the 50-g group than in the 10-g group (p < 0.01). Moreover, the number of TRAP-positive odontoblasts remained higher in the 10-g group than in the control group from days 1 to 7, although the difference was not statistically significant (Figure 4B).
In the control group, caspase 3-, caspase 8-, and RANKL-positive cells were barely observed on the root surfaces from days 1 to 7 (panels a–d in Figures 5A, 6A, and 7A).
In the 10-g group, caspase 3-positive cells appeared on the root surfaces on day 3 and increased in a timedependent manner up to day 7 (Figure 5B), whereas the number of caspase 8-positive cells peaked on day 5 (Figure 6B). In the 50-g group, caspase 8-positive cells appeared on day 1, although they remained within the PDL (panel i in Figure 6A). The number of caspase 3- and caspase 8-positive cells increased on day 3 and peaked on day 5 (Figures 5B and 6B).
RANKL-positive cells were barely observed on the root surfaces in the 10-g group until day 7 (panels e–h in Figure 7A). In contrast, in the 50-g group, RANKL-positive cells were barely observed on the root surfaces until day 5, following which they increased up to day 7 (panels k–1 in Figure 7A and 7B).
Quantitative evaluations revealed that the number of caspase 3- and caspase 8-positive cells in the 50-g group was significantly higher than that in the 10-g group (p < 0.01) and control group (p < 0.01) on days 3 and 5, with no significant differences among groups on days 1 and 7 (Figures 5B and 6B). The number of RANKL-positive cells in the 50-g group was significantly higher than that in the 10-g group (p < 0.01) on day 7, whereas it showed no significant differences between the control and 10-g groups from days 1 to 7 (Figure 7B).
The present study demonstrated that caspases 3 and 8 and RANKL induced by a heavy orthodontic force exacerbate odontoclastogenesis in the cementum. Apoptosis mainly involves an endogenous (mitochondrial) and an exogenous (receptor-related) pathway. Caspases, which are cysteine proteases that cleave a set of proteins to initiate and promote apoptotic signaling, are common to both pathways. While caspase 8 is involved in the extrinsic pathway and is activated by Fas-Fas ligand signaling, caspase 9 is a mediator of the intrinsic pathway and is activated by changes in oxidative phosphorylation, hypoxia, and oxidative stress.15 In the present study, we found that the number of caspase 3- and caspase 8-positive cells was the highest at 5 days after the application of a 50-g orthodontic force (Figures 5B, 6B). Furthermore, we found that TRAP-positive cells (osteoclasts/odontoclasts) appeared at 7 days after the application of a 50-g orthodontic force (Figure 4B). These findings suggest that caspase 3- and caspase 8-positive cells emerge before osteoclasts/odontoclasts under heavy compression forces. Mabuchi et al.16 and Hatai et al.13 noted the existence of apoptotic cells on the compression side after the activation of orthodontic appliances fixed in rat models. Our study adds to these findings by demonstrating that the cementoblasts on root surfaces undergo a greater degree of apoptosis when a force that is heavier than the optimum force is applied. In addition, Zheng et al.17 reported that TNF-α induced the expression of cleaved caspase 3 (a marker of apoptosis) in osteoblasts. We consider that these findings are closely associated with the relationship between caspase and TNF-α. We previously studied the mRNA expression of TNF-α and found that it was significantly increased after the application of compression forces.18 The relationship between ORR and TNF-α has been evaluated in rat models of tooth movement, with the findings suggesting that heavy orthodontic forces result in ORR via the production of TNF-α.19
RANKL induces the production of osteoclasts/osteoclasts. In previous studies, it was reported that an orthodontic force of 50 g induced the production of inflammatory cytokines such as RANKL in the PDL in an intensity-dependent manner.12 In the present study, we focused on RANKL expression in the rat cementum during OTM and found that the number of RANKL-positive cells on the root surfaces was significantly higher in the 50-g group than in the 10-g group on day 7 (Figure 7A and 7B). This finding suggests that RANKL is also present in cementoblasts.
Szymczyk et al.20 reported that caspase 3 activity is required for RANKL-induced osteoclast differentiation. In their study, the number of osteoclasts in the long bones of procaspase 3 knockout mice exhibited a decrease, and RANKL did not promote the differentiation of isolated prechromosomes. Furthermore, neither wild-type nor RAW 264.7 cells expressed TRAP, and they did not multinucleate following treatment with caspase 3 inhibitors. The findings of this study suggest that caspase 3 activity is necessary for RANKL-induced osteoclast/odontoclast differentiation for root resorption.
Cementum is a special mineralized tissue that covers the dental roots. It is classified into two types: acellular cementum, which does not contain cellular components and is distributed on the dental side of the root, and cellular cementum, which contains cementum cells in lacunae. Cementoblasts are located adjacent to the cementum and exhibit a circular or polygonal morphology. These cells have numerous cell processes throughout the canaliculus and are used for communication with adjacent cementum cells as well as bone cells.2122 Cementoblasts also share matrix proteins with bone cells, such as type I collagen, osteopontin, and osteocalcin.23 Hamaya et al.8 reported that osteocytes adjacent to hyaline-treated PDL caused cell death by apoptosis, which could be associated with subsequent bone resorption. Considering the biological similarity between cementum and bone, similar apoptotic changes may occur in the cementoblasts on the compression side of the PDL. In a recent study, Matsuzawa et al.24 demonstrated that the apoptotic cell death of cementocytes was involved in root resorption during OTM; this finding strongly supported the results of the present study.
In the present study, we investigated not only caspase 3 expression but also caspase 8 and RANKL expression in the cementum. In addition, we compared the effects of a heavy orthodontic force with those of an optimum force in rat models of OTM. Interestingly, we found that caspase and RANKL expressions were greater with the heavy force than with the optimum force. Moreover, the expression of caspase 8-positive cells strongly suggested the involvement of the extrinsic pathway of apoptosis. We also focused on the difference in the peak time of increase between caspase- and RANKL-positive cells and found that the number of caspase-positive cells peaked earlier than the number of RANKL-positive cells. Collectively, our findings indicate that, under a heavy orthodontic force, cementoblasts produce caspase 3 and caspase 8, which initiate the activation of the apoptotic pathway. Subsequently, osteoclast production is induced by RANKL, which leads to root resorption. Thus, the causal relationship between root resorption and apoptosis was emphasized in the present study.
Diercke et al.11 reported that the compression of cementoblasts increased the expression of caspases. These gene ontology analyses showed that the compression of HPCBs upregulates the genes associated with apoptosis. The expression of apoptosis-related genes (pre- and antiapoptotic genes) in cementoblasts suggests the involvement of apoptosis in the regulation of cementoblasts during OTM. Because apoptosis is induced in HPCBs after compression and inflammation, it is believed that the death of HPCBs contributes to root resorption through the loss of cementoblast repair activity. Thus, root resorption after OTM may be irreversible. The pathogenesis of ORR is associated with the removal of necrotic tissue from areas of the PDL and cementum under orthodontic compression.2526 Previous studies have also shown that ORR is caused by the removal of necrotic hyalinized tissue.2728 TRAP staining highlighted the involvement of TRAP-positive macrophages and multinucleated giant cells in the removal of hyalinized tissue in a rat model of tooth movement.26 In our quantitative evaluations, the number of TRAP-positive odontoclasts on day 7 was found to be significantly higher in the 50-g group than in the 10-g group; this finding also supports the conclusions of the present study.
In conclusion, the findings of the present study suggest that cementoblasts produce caspase 3 and caspase 8 under heavy orthodontic forces, and this initiates the activation of the apoptotic pathway. Subsequently, osteoclast production is induced by RANKL, which leads to root resorption. Further studies should be conducted to clarify the factors influencing the induction of apoptosis before root resorption in order to develop therapeutic strategies to prevent root shortening due to OTM.
Figures and Tables
Figure 1
The rat model of orthodontic tooth movement used in the present study. Tooth movement is reproduced with a closed coil spring ligated to the maxillary first molar using a 0.008-inch stainless steel ligation wire (wire size, 0.005 inch; diameter, 1/12 inch). The other side of the coil spring is ligated using the same ligation wire to a hole in the maxillary incisor, which is drilled in the cleft just above the gingival papilla using a 1/4 round bur. The maxillary right first molar was moved in the mesial direction via the application of a force of 10 g or 50 g by the sealed coil spring.
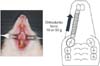
Figure 2
A schematic diagram showing a survey area (shaded box) at the mesial center of the distal root of the maxillary first molar obtained from a rat model of orthodontic tooth movement. The periodontal tissue in the region of compression was defined as the tissue connecting the center of the distobuccal (DB) root and the center of the mesial root (M) of the maxillary first molar, and it comprises one-fourth of the mesial region facing the DB root. The large arrows indicate the direction of force. Root resorption is investigated in 300-µm sections (shaded box) from the area close to the furcation on the mesial surface of the DB root, which was the side of compression during tooth movement.
MP, Mesial palatal root; DP, distal palatal root; MB, mesial buccal root.
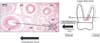
Figure 3
Light microscopic images showing the effects of various orthodontic forces (0, 10, and 50 g) on the expression of multinuclear osteoclasts (hematoxylin and eosin staining, ×400) at 1, 3, 5, and 7 days after force application in a rat model of orthodontic tooth movement. The expression of odontoclasts (arrow) on the root surfaces in the 50-g group is greater than that on the root surfaces in the 10-g and control groups on days 5 and 7. The arrows indicate the sites of root resorption. Scale bar = 50 µm.
PDL, Periodontal ligament; C, cementum; D, dentin.
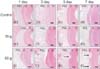
Figure 4
A, Effects of the different orthodontic forces (0, 10, and 50 g) on the expression of tartrate-resistant acid phosphatase (TRAP)-positive odontoclasts at 1, 3, 5, and 7 days after force application in a rat model of orthodontic tooth movement. The arrows indicate TRAP-positive cells (×400). Scale bar = 50 µm. B, A graph showing the quantitative evaluation of cellular changes at 1, 3, 5, and 7 days after force application (0, 10, and 50 g) in a rat model of orthodontic tooth movement.
PDL, Periodontal ligament; C, cementum; D, dentin.
*p < 0.01, significant difference when compared with the control group; †p < 0.01, significant difference when compared with the 10-g group.
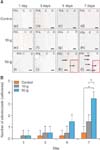
Figure 5
A, Effects of different orthodontic forces (0, 10, and 50 g) on the expression of caspase 3-positive cells at 1, 3, 5, and 7 days after force application in a rat model of orthodontic tooth movement. The arrows indicate caspase 3-positive cells (×400). Scale bar = 50 µm. B, A graph showing the quantitative evaluation of cellular changes at 1, 3, 5, and 7 days after force application (0, 10, and 50 g) in a rat model of orthodontic tooth movement.
PDL, Periodontal ligament; C, cementum; D, dentin.
*p < 0.01, significant difference when compared with the control group; †p < 0.01, significant difference when compared with the 10-g group.
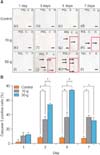
Figure 6
A, Effects of different orthodontic forces (0, 10, and 50 g) on the expression of caspase 8-positive cells at 1, 3, 5, and 7 days after force application in a rat model of orthodontic tooth movement. The arrows indicate caspase 8-positive cells (×400). Scale bar = 50 µm. B, A graph showing the quantitative evaluation of cellular changes at 1, 3, 5, and 7 days after force application (0, 10, and 50 g) in a rat model of orthodontic tooth movement.
PDL, Periodontal ligament; C, cementum; D, dentin.
*p < 0.05 and **p < 0.01, significant difference when compared with the control group; †p < 0.01, significant difference when compared with the 10-g group.
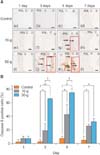
Figure 7
A, Effects of different orthodontic forces (0, 10, and 50 g) on the expression of receptor activator of nuclear factor kappa-B ligand (RANKL)-positive cells at 1, 3, 5, and 7 days after force application in a rat model of orthodontic tooth movement. The arrows indicate RANKL-positive cells (×400). Scale bar = 50 µm. B, A graph showing the quantitative evaluation of cellular changes at 1, 3, 5, and 7 days after force application (0, 10, and 50 g) in a rat model of orthodontic tooth movement.
PDL, Periodontal ligament; C, cementum; D, dentin.
*p < 0.01, significant difference when compared with the control group; †p < 0.05 and ††p < 0.01, significant difference when compared with the 10-g group.
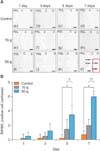
ACKNOWLEDGEMENTS
This research was supported, in part, by a Grant-in-Aid for Scientific Research from the Japan Society for the Promotion of Science (16K11795, 16K11796, 16K20656, 17K17339, 15H06648).
References
1. Kaley J, Phillips C. Factors related to root resorption in edgewise practice. Angle Orthod. 1991; 61:125–132.
2. Linge BO, Linge L. Apical root resorption in upper anterior teeth. Eur J Orthod. 1983; 5:173–183.


3. Owman-Moll P, Kurol J, Lundgren D. The effects of a four-fold increased orthodontic force magnitude on tooth movement and root resorptions. An intra-individual study in adolescents. Eur J Orthod. 1996; 18:287–294.


4. Owman-Moll P, Kurol J, Lundgren D. Effects of a doubled orthodontic force magnitude on tooth movement and root resorptions. An inter-individual study in adolescents. Eur J Orthod. 1996; 18:141–150.


5. Chan E, Darendeliler MA. Physical properties of root cementum: Part 5. Volumetric analysis of root resorption craters after application of light and heavy orthodontic forces. Am J Orthod Dentofacial Orthop. 2005; 127:186–195.


6. Hikida T, Yamaguchi M, Shimizu M, Kikuta J, Yoshino T, Kasai K. Comparisons of orthodontic root resorption under heavy and jiggling reciprocating forces during experimental tooth movement in a rat model. Korean J Orthod. 2016; 46:228–241.


7. Baud V, Karin M. Signal transduction by tumor necrosis factor and its relatives. Trends Cell Biol. 2001; 11:372–377.


8. Hamaya M, Mizoguchi I, Sakakura Y, Yajima T, Abiko Y. Cell death of osteocytes occurs in rat alveolar bone during experimental tooth movement. Calcif Tissue Int. 2002; 70:117–126.


9. Rana MW, Pothisiri V, Killiany DM, Xu XM. Detection of apoptosis during orthodontic tooth movement in rats. Am J Orthod Dentofacial Orthop. 2001; 119:516–521.


10. Wu CC, Lin JP, Yang JS, Chou ST, Chen SC, Lin YT, et al. Capsaicin induced cell cycle arrest and apoptosis in human esophagus epidermoid carcinoma CE 81T/VGH cells through the elevation of intracellular reactive oxygen species and Ca2+ productions and caspase-3 activation. Mutat Res. 2006; 601:71–82.


11. Diercke K, Zingler S, Kohl A, Lux CJ, Erber R. Gene expression profile of compressed primary human cementoblasts before and after IL-1β stimulation. Clin Oral Investig. 2014; 18:1925–1939.


12. Nakano Y, Yamaguchi M, Fujita S, Asano M, Saito K, Kasai K. Expressions of RANKL/RANK and M-CSF/c-fms in root resorption lacunae in rat molar by heavy orthodontic force. Eur J Orthod. 2011; 33:335–343.


13. Hatai T, Yokozeki M, Funato N, Baba Y, Moriyama K, Ichijo H, et al. Apoptosis of periodontal ligament cells induced by mechanical stress during tooth movement. Oral Dis. 2001; 7:287–290.


14. Asano M, Yamaguchi M, Nakajima R, Fujita S, Utsunomiya T, Yamamoto H, et al. IL-8 and MCP-1 induced by excessive orthodontic force mediates odontoclastogenesis in periodontal tissues. Oral Dis. 2011; 17:489–498.


15. Boatright KM, Renatus M, Scott FL, Sperandio S, Shin H, Pedersen IM, et al. A unified model for apical caspase activation. Mol Cell. 2003; 11:529–541.


16. Mabuchi R, Matsuzaka K, Shimono M. Cell proliferation and cell death in periodontal ligaments during orthodontic tooth movement. J Periodontal Res. 2002; 37:118–124.


17. Zheng L, Wang W, Ni J, Mao X, Song D, Liu T, et al. Role of autophagy in tumor necrosis factor-α-induced apoptosis of osteoblast cells. J Investig Med. 2017; 65:1014–1020.


18. Mitsuhashi M, Yamaguchi M, Kojima T, Nakajima R, Kasai K. Effects of HSP70 on the compression force-induced TNF-α and RANKL expression in human periodontal ligament cells. Inflamm Res. 2011; 60:187–194.


19. Matsumoto Y, Sringkarnboriboon S, Ono T. Proinflammatory mediators related to orthodontically induced periapical root resorption in rat mandibular molars. Eur J Orthod. 2017; 39:686–691.


20. Szymczyk KH, Freeman TA, Adams CS, Srinivas V, Steinbeck MJ. Active caspase-3 is required for osteoclast differentiation. J Cell Physiol. 2006; 209:836–844.


21. Kagayama M, Sasano Y, Mizoguchi I, Takahashi I. Confocal microscopy of cementocytes and their lacunae and canaliculi in rat molars. Anat Embryol (Berl). 1997; 195:491–496.


22. Ayasaka N, Kondo T, Goto T, Kido MA, Nagata E, Tanaka T. Differences in the transport systems between cementocytes and osteocytes in rats using microperoxidase as a tracer. Arch Oral Biol. 1992; 37:363–369.


23. Sasano Y, Maruya Y, Sato H, Zhu JX, Takahashi I, Mizoguchi I, et al. Distinctive expression of extracellular matrix molecules at mRNA and protein levels during formation of cellular and acellular cementum in the rat. Histochem J. 2001; 33:91–99.
24. Matsuzawa H, Toriya N, Nakao Y, Konno-Nagasaka M, Arakawa T, Okayama M, et al. Cementocyte cell death occurs in rat cellular cementum during orthodontic tooth movement. Angle Orthod. 2017; 87:416–422.


25. Brudvik P, Rygh P. Root resorption beneath the main hyalinized zone. Eur J Orthod. 1994; 16:249–263.


26. Kurol J, Owman-Moll P. Hyalinization and root resorption during early orthodontic tooth movement in adolescents. Angle Orthod. 1998; 68:161–165.