Abstract
The aim of this study was to evaluate the in vitro anti-inflammatory and utero-relaxant effect of α-bisabolol on the pregnant human myometrium. Samples from the pregnant human myometrium were used in functional tests to evaluate the inhibitory effect of α-bisabolol (560, 860, 1,200 and 1,860 µM) on spontaneous myometrial contractions. The intracellular cyclic adenosine monophosphate (cAMP) levels generated in response to α-bisabolol in human myometrial homogenates were measured by ELISA. The anti-inflammatory effect of α-bisabolol was determined through the measurement of two pro-inflammatory cytokines, tumor necrosis factor-α (TNFα) and interleukin (IL)-1β, and the anti-inflammatory cytokine IL-10, in pregnant human myometrial explants stimulated with lipopolysaccharide (LPS). Forskolin was used as a positive control to evaluate the cAMP and cytokine levels. α-Bisabolol was found to induce a significant inhibition of spontaneous myometrial contractions at the highest concentration level (p<0.05). α-Bisabolol caused a concentration-dependent decrease in myometrial cAMP levels (p<0.05) and a concentration-dependent decrease in LPS-induced TNFα and IL-1β production, while IL-10 production did not increase significantly (p>0.05). The anti-inflammatory and utero-relaxant effects induced by α-bisabolol were not associated with an increase in cAMP levels in pregnant human myometrial samples. These properties place α-bisabolol as a potentially safe and effective adjuvant agent in cases of preterm birth, an area of pharmacological treatment that requires urgent improvement.
Currently, there is growing interest in the potential for inflammation to play an adverse role in preterm birth and fetal health [1]. Pathological processes that have been linked with preterm birth infection and intrauterine inflammation are most frequently found to be associated with the induction of labor [2]. During the inflammation associated with infection, prostaglandins are simultaneously released with Nitric oxide, and their overproduction could be detrimental [2]. The secretion of inflammatory cytokines by fetal and maternal tissues, such as interleukin 1β (IL-1β) and tumor necrosis factor α (TNF-α), are associated with the release of prostaglandins, which promote uterine contractions, thus contributing to embryonic and fetal expulsion [3]. Some mechanisms involved in uterine relaxation are associated with the production of some second messengers, such as cyclic adenosine monophosphate (cAMP), which actives the cAMP-dependent protein kinase A (PKA). PKA is responsible for the phosphorylation and inhibition of key proteins involved in uterine contractions [3]. Currently, tocolytic drugs, such as nitric oxide donors, calcium channel blockers, oxytocin receptor blockers, beta-adrenoceptor agonists and cyclo-oxygenase inhibitors [4], are used for the treatment of preterm birth as a first-line therapy [24]. These drugs have a different mechanism of action, such as reducing the numbers of some inflammatory mediators, inhibiting calcium channels, and increasing the intracellular concentrations of cAMP through the activation of adenylyl cyclase (AC) [4]. Moreover, they are widely used for tocolysis, despite the fact that their use has not been approved by pharmaceutical agencies or ones from the United States, and that their use has been strongly associated with several serious adverse effects in both mother and fetus [56]. Due to the growing importance of the discovery and development of new drugs for the pharmacological treatment of preterm birth associated with inflammation and infection, it is necessary to identify new molecular targets involved in uterine contractions and inflammation [37]. (−)-α-Bisabolol (IUPAC name: (2R)-6-methyl-2-[(1R)-4-methylcyclohex-3-en-1-yl] hept-5-en-2-ol) is an unsaturated, optically active sesquiterpene alcohol obtained by the direct distillation of essential oil from plants, such as Vanillosmopsis erythropappa and Matricaria chamomilla and has been associated with anti-inflammatory and antibacterial properties, and is thought to be beneficial for skin-care [89]. Some researchers have suggested that the anti-inflammatory effect of α-bisabolol might be linked to the inhibition of some proteins, such as inducible nitric oxide synthase (iNOS), cyclooxygenase-2 (COX-2) and the transcription factors NF-kB, ERK and p38 [10]. Recently, new findings have shown that α-bisabolol is involved in the inhibition of the cAMP response element (CRE) by reducing intracellular cAMP levels in melanocyte cells [11]; however, it has also been demonstrated that α-bisabolol could act as an inhibitor of voltage-dependent calcium channels by promoting the inhibition of smooth muscle contractions in isolated rat arteries, as reported by [12]. To date, no studies have been conducted on the relaxing, immunomodulatory and anti-inflammatory properties of α-bisabolol in the pregnant human uterus. In order to corroborate such properties, this study first carried out research on the relaxing effect of α-bisabolol on spontaneous contractions in the pregnant human myometrium. It then assessed its immunomodulatory and anti-inflammatory effect on the production of pro- and anti-inflammatory cytokines induced by lipopolysaccharides (LPS) in the pregnant human myometrium, an effect which has not been previously reported. Therefore, the aim of the present study was to evaluate and corroborate the relaxant and anti-inflammatory effects of α-bisabolol on the pregnant human uterus.
Nineteen biopsies of human myometrial tissue were obtained at caesarian section in the last two weeks of pregnancy (39–40 weeks of gestation; n=19). None of the pregnant woman had been administered a tocolytic drug prior to the caesarian. Myometrial biopsies were excised from the longitudinal layer at the antiplacental site in the uterine body after the delivery of the child. The tissues were placed immediately in cold physiological salt solution and the sample then transported to the laboratory and kept refrigerated (4–8℃) for 24 h. The myometrial samples were dissected free of serosa at a distance from any macroscopic abnormality. Written informed consent was obtained from all donors. The present study was approved by the Ethics Committee at the Hospital General de los Servicios de Salud de Hidalgo (the Health Services of Hidalgo General Hospital), Pachuca, Hidalgo, Mexico, and was performed in accordance with the Declaration of Helsinki.
α-Bisabolol (anti-irritant and anti-inflammatory agent), forskolin (direct AC activator), Escherichia coli LPS (serotype 055:B5), and dimethyl sulfoxide (DMSO) were purchased from Sigma-Aldrich (St. Louis, MO, USA). Antibiotics, penicillin-streptomycin (10,000 U/ml), Dulbecco's modified Eagle's medium and Dulbecco phosphate buffered saline (PBS 10×) were purchased in Mexico from Gibco, ThermoFisher Scientific.
Isolated myometrial biopsies were placed in a Ringer's physiological solution (containing, in mM: NaCl 118, KCl 5, CaCl2 2, MgSO4 0.5, K2SO4 1, NaHCO3 25, glucose 10; pH 7.4) bath in order to clean them of excess blood. Each myometrial sample was cut into at least six strips (10×3 mm) and vertically mounted in chambers containing 3 ml of Ringer solution. After mounting, the Ringer solution was changed repeatedly until the basal tension record was equilibrated to 1 g. The tissues were maintained in the Ringer solution bath at 37℃, with constant bubbling of 5% CO2 in O2 before the contractility assays, which were undertaken once the spontaneous contractions presented uniform functional activity. The changes in the contractile activity of the isometric tension were recorded using a Grass FT03 tension transducer coupled to an RPS-312 RM model polygraph (Grass Telefactor, RI, USA). The data were analyzed using PolyVIEW software, version 2.5, while the uterine contractions (integral activity) were measured using the area under the curve (AUC) defined by the graphic isometric record over a 20 min period after stabilization. The inhibitory effect of α-bisabolol on spontaneous uterine contractions was expressed as follows:
AUCr is the AUC which remains after the exposure of uterine tissue to the drug, while AUCi is the AUC of the integral activity prior to the addition of any compound. A period of 20 min, before and after exposition to drugs, was considered sufficient to obtain stable and representative biological activity. The utero-relaxant effect was graphed in columns using the following concentrations of α-bisabolol: 560, 860, 1,200 and 1,860 µM.
The uterine tissue (approx. 20–30 mg) was washed four times in PBS (1×) to remove excess blood and then incubated for 3 h on 24 well plates, which contained 1 ml of Dulbecco's serum-free modified Eagle's medium supplemented with 100 U/ml penicillin and 100 µg/ml streptomycin (Sigma-Aldrich, St. Louis, MO, USA) at 37℃ in a humidified atmosphere with 5% CO2. After 3 h of incubation, the culture medium was changed and the uterine tissues incubated, first with α-bisabolol at increasing concentrations of 560, 860 and 1,200 µM for 1 h, and then with forskolin at a concentration of 10 µM for 30 min. The concentrations were selected from a prior contractility study and the concentration of forskolin was selected from a prior study [13]. All compounds were dissolved in 10 µl of DMSO. After the stimulation, the samples were collected, frozen in liquid nitrogen and stored at −80℃, until being used as reported [14]. Frozen tissue samples were homogenized (20–30 mg/ml), with a biospec tissue-tearor laboratory homogenizer (BioSpec Products Inc., Bartlesville, OK, USA) in ice-cold homogenization buffer (2 mM MgSO4, 2 mM EDTA, 1 mM β-mercaptoethanol, 100 mM Tris/HCl, 10% glycerol; pH 7.4), and then supplemented with a protease inhibitor cocktail [14]. The cAMP assay was performed following the instructions of the direct cAMP ELISA Assay kit (Enzo Life Sciences, Inc., Exeter, UK). cAMP concentrations were determined at 405 nm using a Multiskan EX plate reader (Thermo Scientific, Vantaa, Finland) and expressed as pg/ml.
For cytokine measurement via enzyme-linked immunoassay experiments, the pregnant human uterine explants were cut into small ~20–30 mg pieces and washed 4 times with 1× PBS and then placed in 24 well plates, which contained 1 ml of culture medium per well (Dulbecco's modified Eagle's medium) supplemented with 100 U/ml penicillin and 100 µg/ml streptomycin. The explants were incubated at 37℃ in a 5% CO2 humidified atmosphere for 3 h, with the samples then transferred to 24 new well plates containing fresh culture medium. The uterine explants were incubated with increasing concentrations of 560, 860 and 1,200 µM of α-bisabolol and 10 µM of forskolin, both with and without 10 µg/ml of LPS for 24 h, as recommended [3]. All compounds were dissolved in DMSO. Each experiment was carried out in duplicate. After the incubation period, the supernatants were collected and stored at −20℃, until used as reported [14]. The concentrations of TNF-α, IL-1β and IL-10 in the supernatants of undiluted culture medium were determined using human TNF-α, IL-1β and IL-10 ELISA kits (Novex Life Technologies, Waltham, MA, USA), following the manufacturer's directions, with the samples read using a Multiskan EX plate reader. Cytokine concentrations were expressed as pg/ml.
Data for the effect of the drugs of interest on spontaneous uterine contractions were subjected to a concentration-response column analysis performed using Sigma Plot version 10 software (Systat Software Inc., San Jose, CA, USA) in order to obtain values for inhibitory concentration 50 (IC50) (a drug concentration that produces 50% of the maximum inhibitory effect), and Emax, a maximum inhibitory response that can be produced by the highest concentration of the tested compound. Data are expressed as the means±SEM taken from determinations for each concentration (n=5). The IC50 and Emax differences among the compounds were determined by a one-way ANOVA test followed by a post-hoc Student-Newman-Keuls test using Sigma Stat software, version 3.1 (Systat Software Inc., San Jose, CA, USA). In all cases, p<0.05 was considered statistically significant.
One of the aims of this research was to study the relaxation effects of α-bisabolol on spontaneous contractions of the pregnant human myometrium. Fig. 1A shows five columns related to the utero-relaxant effect induced by α-bisabolol in a concentration-dependent manner, reaching a maximum inhibition of 92% (Emax) at the concentration of 1.86 mM, the highest concentration tested. A statistically significant difference was found between the basal value obtained from DMSO and the values for the increasing concentrations of α-bisabolol, p<0.05. Fig. 1B shows a typical recording of the inhibition of the spontaneous uterine contractions of the pregnant human myometrium as induced by α-bisabolol.
Forskolin, a well-known AC activator (10 µM), increased more than twice the amount of cAMP in the pregnant human myometrium, when compared to the basal amount (p<0.05), which could not be modified by the vehicle DMSO used to dissolve the compounds (Fig. 2). α-Bisabolol (560, 860, 1,200 µM) decreased the basal and forskolin-induced myometrial cAMP levels in a concentration-dependent manner (Fig. 2) (p<0.05).
In order to determine the anti-inflammatory properties of α-bisabolol, the preventive effect on the production and release of the pro-inflammatory cytokines TNF-α (Fig. 3) and IL-1β (Fig. 4) were evaluated in LPS-stimulated human myometrial explants. LPS significantly increased the levels of both cytokines (Figs. 3 and 4) (p<0.05), while LPS-induced TNF-α and IL-1β levels were significantly decreased by α-bisabolol (560–1,200 µM). While the combination of forskolin and LPS significantly increased both pro-inflammatory cytokines, TNF-α and IL-1β (Figs. 3 and 4) (p<0.05), when α-bisabolol (560–1,200 µM) was combined with LPS and forskolin, the TNF-α and IL-1β levels decreased in a significant concentration-dependent manner (p<0.05).
In order to confirm the immunomodulatory properties of α-bisabolol, the production of the anti-inflammatory cytokine IL-10 was assessed (Fig. 5). While DMSO and LPS did not significantly elevate IL-10 concentrations (p>0.05), the combination of LPS with α-bisabolol (860 µM) showed a slight increase in IL-10 levels (p<0.05). Both forskolin used alone and the LPS-forskolin combination induced a significant increase in the concentration of IL-10 compared with the basal control (p<0.05). On the other hand, the combination of α-bisabolol (860 µM) with LPS and forskolin significantly decreased the concentration of IL-10 in contrast with the combination of LPS and forskolin (p<0.05).
Preterm birth, defined as a delivery occurring between 20 and 37 weeks of gestation, is a worldwide epidemic. The incidence of preterm birth is approximately of 15 million per year [15]. This condition is an important contributor to perinatal mortality and morbidity and an economic burden on society [16]. It is now clear that the causes of preterm labor are multifactorial; however, the most important common pathways leading to premature birth are systemic maternal genital tract infections, currently considered the main etiology. The use of tocolytic drugs is the main option for the treatment of preterm birth. Unfortunately, as their use has been frequently associated with low efficacy and adverse reactions [456], the development of new drugs for the treatment of premature birth is an area of growing interest. Therefore, new drugs or herbal extracts are being used in preterm birth models both in vitro and in vivo in order to explore their possible therapeutic benefits. Recently, some herbal extract compounds, such as the terpene and flavonoid classes, have been linked to properties with a relaxing effect on uterine smooth muscle [17]. α-Bisabolol is a natural unsaturated monocyclic sesquiterpene alcohol obtained mainly from Matricaria recutita and Myoporum crassifolium [89]. In this study, α-bisabolol was able to induce a significant utero-relaxant effect on the spontaneous contractions of strips isolated from the pregnant human myometrium. To date, this constitutes the first report on the ability of α-bisabolol to inhibit the spontaneous contractions of the pregnant human uterus. Such a property might be related to the blockade of calcium channels. The myometrium consists mainly of uterine smooth muscle cells, also called uterine myocytes, whose main function is to induce spontaneous uterine contractions [18]. In fact, the specialized smooth muscle cells that regulate the spontaneous electrical behavior of the uterus during the pregnancy are known as pacemaker cells [19]. Myometrial smooth muscle cells predominantly possess L-type calcium channels on the cell surface [20], which regulate the transport of calcium from the extracellular space into the intracellular compartment [2021]. It has been reported that α-bisabolol is able to inhibit the smooth muscle contractions of tracheal rings isolated from porcine coronary and splenic arteries, with such effects linked to the inhibition of voltage-dependent calcium channels [922]. These data are consistent with the results obtained by this research, which show that α-bisabolol can inhibit spontaneous contractions in the pregnant human uterus, an effect which could also be related to the blockade of the calcium channels, as reported in the studies mentioned above. Further studies are required to evaluate, in detail, the α-bisabolol mechanism of action involved in the blockade of voltage-gated calcium channels in the uterine smooth muscle.
cAMP is the most common endogenous second messenger that plays a key role in the regulation of several biologic responses in humans, such as the inflammation of immune cells [323]. Many cell-surface receptors are coupled to a signal-transducing G protein. Usually, ligand binding to these receptors activates their associated G protein, which then activates an effector enzyme, which, in turn, generates an intracellular second messenger, such as cAMP [24]. cAMP acts directly on three main targets: protein kinase A (PKA); the exchange protein activated by cAMP (Epac); and, cyclic nucleotide-gated ion channels (CNGC) [2324]. PKA modulates, via phosphorylation, a number of cellular substrates, including transcription factors, ion channels, transporters, exchangers, and intracellular calcium-handling proteins, as well as the contractile machinery [232425]. In order to confirm whether the utero-relaxant effect induced by α-bisabolol was caused by intracellular cAMP levels, this research measured the production of cAMP in the pregnant human myometrium in the presence of α-bisabolol, using the specific AC activator forskolin as a positive control [26]. In this study, α-bisabolol alone decreased the basal and forskolin-induced cAMP production in a concentration-dependent manner (p<0.05) (Fig. 2). These data suggest that the utero-relaxant effect induced by α-bisabolol on spontaneous contractions in the pregnant human myometrium was not associated with the cAMP production. However, further studies are required to determine how α-bisabolol induces the inhibition of the uterine contraction.
Inflammation is a bodily response to harmful stimuli such as infection and injury [27]. Some infectious agents from pathogenic bacteria can stimulate the release of proinflammatory mediators, such as prostaglandins, TNF-α and IL-1β, and finally promote inflammation and immunological responses [28]. LPS, a component of the outer membrane of gram-negative bacteria, can induce an overproduction of various inflammatory molecules, such as TNF-α, IL-1β and IL-6. LPS is a strong inducer of immune responses in premature human parturition [32829]. Some plant-derived immunomodulatory agents modify inflammatory responses by inducing an anti-inflammatory microenvironment through the release of immunosuppressive and anti-inflammatory cytokines, such as IL-10, IL-4 and others. Moreover, their potential inhibitory effects on the production of proinflammatory cytokines have been studied in in vitro models, such as LPS-stimulated rat kupffer cells, murine macrophages, human monocytes and the pregnant human myometrium [14303132333435]. Therefore, these cell and tissue culture systems are excellent models for studying and identifying new immunomodulatory and anti-inflammatory agents for the treatment of preterm birth associated with infection [1436]. The results of this study show that α-bisabolol promotes an interesting anti-inflammatory effect on the pregnant human myometrium, an effect which was associated with the inhibition of the production of TNF-α and IL-1β, and with the increase of the anti-inflammatory cytokine IL-10 (Figs. 3, 4, 5). Furthermore, the combination of forskolin and α-bisabolol seems to enhance the inhibition of the production of the proinflammatory cytokine. Interestingly, the combination of α-bisabolol with forskolin and LPS decreased the levels of IL-10 compared to forskolin alone, suggesting that α-bisabolol and forskolin are likely to have different mechanisms of action. cAMP acts mainly through the serine/threonine-specific protein kinase A (PKA) in order to modulate, via phosphorylation, different cellular substrates, including the nuclear transcription factor known as the cAMP response element binding protein (CREB) [23], which promotes the production of interleukin 10 (IL-10), a well-known anti-inflammatory mediator [25]. The proposed signaling pathways involved in proinflammatory cytokine production occur via PKA/CREB and NF-κB inhibition, as reported in melanocyte and mice [3738]. Researchers have suggested that the inactivation of the NF-κB transcription factor did not affect IL-10 production [33], instead increasing it, as the IL-10 transcription is regulated by seven different transcription factors in macrophages [34]. The proposed inactivation of the CREB transcription factor by α-bisabolol could lead to a decrease in intracellular cAMP levels, which is a key process for the activation of CREB [3839]. In summary, the inhibition of the cAMP/PKA/CREB signaling pathway contributes to the deregulation of IL-10 production, which has been shown to be a crucial process for the activation of the gene transcription of inflammatory cytokines, such as TNFα, IL-1β and IL-6 [4041]. On the other hand, α-bisabolol, as a calcium-channel blocker, could, consequently, affect the secretion of inflammatory cytokines, such as TNF-α and IL-1β, as has been reported in human neutrophils [42]. The results of this study show that the immunomodulatory and anti-inflammatory effects induced by α-bisabolol were consistent with other reports, which demonstrated the ability of α-bisabolol to inhibit the release of the proinflammatory cytokines induced with LPS in mice and human dendritic cells [4344]. As proposed in other studies, some molecules from herbal extracts, known as flavonoids and terpenes, could exert their anti-inflammatory effects by deregulating the expression of genes associated with inducible nitric oxide synthase (iNOS) and cyclooxygenase-2 (COX-2). However, it has also been suggested that the mechanism of action might be related to the inhibition of NF-kB signaling and the negative regulation of the transcription of the ERK and p38 transcription factors [104344]. The exact mechanism of action of α-bisabolol responsible for inhibiting the production of TNFα and IL-1β is yet to be completely clarified [44]. In light of the possible side effects of α-bisabolol, some reports have shown this compound to be non-toxic in both animal models and dermatological clinical trials [4546].
ACKNOWLEDGEMENTS
This work was supported by the Programa Anual de Investigación (PAI) 2015-UAEH-DI-ICSA-MED-SF-062 and Red Temática : Farmacología de la Reproducción grants from REDES-PRODEP-SEP, Mexico. The authors thank Shamayra Sauz Hernández and Karina Reséndiz Sánchez from the SSH General Hospital, Pachuca, Hidalgo, Mexico, for their support and collaboration in the gynecological field.
Notes
Author contributions: V.M.M.P. performed the functional and ELISA experiments and wrote the manuscript. M.I.O. coordinated the study, reviewed the manuscript and analyzed the data. H.A.P.M. supervised the functional experiments and reviewed the manuscript. V.M.P. and G.B.R. isolated and provided the human biological samples and reviewed the manuscript.
References
1. Kemp MW, Saito M, Newnham JP, Nitsos I, Okamura K, Kallapur SG. Preterm birth, infection, and inflammation advances from the study of animal models. Reprod Sci. 2010; 17:619–628. PMID: 20581349.


2. Burdet J, Rubio AP, Salazar AI, Ribeiro ML, Ibarra C, Franchi AM. Inflammation, infection and preterm birth. Curr Pharm Des. 2014; 20:4741–4748. PMID: 24588830.


3. Muñoz-Pérez VM, Fernández-Martínez E, Ponce-Monter H, Ortiz MI. Relaxant and anti-inflammatory effect of two thalidomide analogs as PDE-4 inhibitors in pregnant rat uterus. Korean J Physiol Pharmacol. 2017; 21:429–437. PMID: 28706457.


4. Hubinont C, Debieve F. Prevention of preterm labour: 2011 update on tocolysis. J Pregnancy. 2011; 2011:941057. PMID: 22175022.


5. de Heus R, Mol BW, Erwich JJ, van Geijn HP, Gyselaers WJ, Hanssens M, Härmark L, van Holsbeke CD, Duvekot JJ, Schobben FF, Wolf H, Visser GH. Adverse drug reactions to tocolytic treatment for preterm labour: prospective cohort study. BMJ. 2009; 338:b744. PMID: 19264820.


6. Abitayeh G, Tsatsaris V, Goffinet F, Cabrol D. New tocolytic agents. Eur Clinics Obstet Gynecol. 2005; 1:29–35.


7. Agrawal V, Hirsch E. Intrauterine infection and preterm labor. Semin Fetal Neonatal Med. 2012; 17:12–19. PMID: 21944863.


8. Rocha NF, Oliveira GV, Araújo FY, Rios ER, Carvalho AM, Vasconcelos LF, Macêdo DS, Soares PM, Sousa DP, Sousa FC. (−)-α-Bisabolol-induced gastroprotection is associated with reduction in lipid peroxidation, superoxide dismutase activity and neutrophil migration. Eur J Pharm Sci. 2011; 44:455–461. PMID: 21924353.


9. de Siqueira RJ, Freire WB, Vasconcelos-Silva AA, Fonseca-Magalhães PA, Lima FJ, Brito TS, Mourão LT, Ribeiro RA, Lahlou S, Magalhães PJ. In-vitro characterization of the pharmacological effects induced by (-)-α-bisabolol in rat smooth muscle preparations. Can J Physiol Pharmacol. 2012; 90:23–35. PMID: 22171824.


10. Ko SG, Yin CS, Du B, Kim KH. Herbal medicines for inflammatory diseases 2016. Mediators Inflamm. 2016; 2016:8270323. PMID: 27847408.


11. Jeon JS, Kim HT, Kim MG, Oh MS, Hong SR, Yoon MH, Shin HC, Shim JH, Afifi NA, Hacımüftüoğlu A, El-Aty AMA. Simultaneous detection of glabridin, (−)-α-bisabolol, and ascorbyl tetraisopalmitate in whitening cosmetic creams using HPLC-PAD. Chromatographia. 2016; 79:851–860.


12. de Siqueira RJ, Ribeiro-Filho HV, Freire RS, Cosker F, Freire WB, Vasconcelos-Silva AA, Soares MA, Lahlou S, Magalhães PJ. (−)-α-Bisabolol inhibits preferentially electromechanical coupling on rat isolated arteries. Vascul Pharmacol. 2014; 63:37–45. PMID: 25128618.


13. Fernández-Martínez E, Ponce-Monter H, Soria-Jasso LE, Ortiz MI, Arias-Montaño JA, Barragán-Ramírez G, Mayén-García C. Inhibition of uterine contractility by thalidomide analogs via phosphodiesterase-4 inhibition and calcium entry blockade. Molecules. 2016; 21:E1332. PMID: 27739411.


14. Oger S, Méhats C, Barnette MS, Ferré F, Cabrol D, Leroy MJ. Anti-inflammatory and utero-relaxant effects in human myometrium of new generation phosphodiesterase 4 inhibitors. Biol Reprod. 2004; 70:458–464. PMID: 14561639.


15. Kinney MV, Lawn JE, Howson CP, Belizan J. 15 Million preterm births annually: what has changed this year? Reprod Health. 2012; 9:28. PMID: 23148557.


16. Purisch SE, Gyamfi-Bannerman C. Epidemiology of preterm birth. Semin Perinatol. 2017; 41:387–391. PMID: 28865982.


17. Rezaeizadeh G, Hantoushzadeh S, Ghiasi S, Nikfar S, Abdollahi M. A systematic review of the uterine relaxant effect of herbal sources. Curr Pharm Biotechnol. 2016; 17:934–948. PMID: 27396394.


18. Tribe RM. Regulation of human myometrial contractility during pregnancy and labour: are calcium homeostatic pathways important? Exp Physiol. 2001; 86:247–254. PMID: 11429641.


19. Garfield RE, Maner WL. Physiology and electrical activity of uterine contractions. Semin Cell Dev Biol. 2007; 18:289–295. PMID: 17659954.


20. Pehlivanoğlu B, Bayrak S, Doğan M. A close look at the contraction and relaxation of the myometrium; the role of calcium. J Turk Ger Gynecol Assoc. 2013; 14:230–234. PMID: 24592112.
21. Luckas MJ, Taggart MJ, Wray S. Intracellular calcium stores and agonist-induced contractions in isolated human myometrium. Am J Obstet Gynecol. 1999; 181:468–476. PMID: 10454702.


22. Roberts RE, Allen S, Chang AP, Henderson H, Hobson GC, Karania B, Morgan KN, Pek AS, Raghvani K, Shee CY, Shikotra J, Street E, Abbas Z, Ellis K, Heer JK, Alexander SP. Distinct mechanisms of relaxation to bioactive components from chamomile species in porcine isolated blood vessels. Toxicol Appl Pharmacol. 2013; 272:797–805. PMID: 23845591.


23. Sassone-Corsi P. The cyclic AMP pathway. Cold Spring Harb Perspect Biol. 2012; 4:a011148. PMID: 23209152.


24. Taskén K, Aandahl EM. Localized effects of cAMP mediated by distinct routes of protein kinase A. Physiol Rev. 2004; 84:137–167. PMID: 14715913.
25. Brenner S, Prösch S, Schenke-Layland K, Riese U, Gausmann U, Platzer C. cAMP-induced Interleukin-10 promoter activation depends on CCAAT/enhancer-binding protein expression and monocytic differentiation. J Biol Chem. 2003; 278:5597–5604. PMID: 12493739.


26. Insel PA, Ostrom RS. Forskolin as a tool for examining adenylyl cyclase expression, regulation, and G protein signaling. Cell Mol Neurobiol. 2003; 23:305–314. PMID: 12825829.
27. Choi YH, Kim GY, Lee HH. Anti-inflammatory effects of cordycepin in lipopolysaccharide-stimulated RAW 264.7 macrophages through Toll-like receptor 4-mediated suppression of mitogen-activated protein kinases and NF-κB signaling pathways. Drug Des Devel Ther. 2014; 8:1941–1953.
28. Cha JY, Jung JY, Jung JY, Lee JR, Cho IJ, Ku SK, Byun SH, Ahn YT, Lee CW, Kim SC, An WG. Inhibitory effects of traditional herbal formula pyungwi-san on inflammatory response in vitro and in vivo. Evid Based Complement Alternat Med. 2013; 2013:630198. PMID: 23533508.
29. Gomez-Lopez N, StLouis D, Lehr MA, Sanchez-Rodriguez EN, Arenas-Hernandez M. Immune cells in term and preterm labor. Cell Mol Immunol. 2014; 11:571–581. PMID: 24954221.


30. Jantan I, Ahmad W, Bukhari SN. Plant-derived immunomodulators: an insight on their preclinical evaluation and clinical trials. Front Plant Sci. 2015; 6:655. PMID: 26379683.


31. Kiemer AK, Müller C, Vollmar AM. Inhibition of LPS-induced nitric oxide and TNF-alpha production by alpha-lipoic acid in rat Kupffer cells and in RAW 264.7 murine macrophages. Immunol Cell Biol. 2002; 80:550–557. PMID: 12406389.
32. Saad B, Abouatta BS, Basha W, Hmade A, Kmail A, Khasib S, Said O. Hypericum triquetrifolium-derived factors downregulate the production levels of LPS-induced nitric oxide and tumor necrosis factor-α in THP-1 cells. Evid Based Complement Alternat Med. 2011; 2011:586470. PMID: 18955363.
33. Iyer SS, Cheng G. Role of interleukin 10 transcriptional regulation in inflammation and autoimmune disease. Crit Rev Immunol. 2012; 32:23–63. PMID: 22428854.


34. Saraiva M, O'Garra A. The regulation of IL-10 production by immune cells. Nat Rev Immunol. 2010; 10:170–181. PMID: 20154735.


35. Kelly A, Lynch A, Vereker E, Nolan Y, Queenan P, Whittaker E, O'Neill LA, Lynch MA. The anti-inflammatory cytokine, interleukin (IL)-10, blocks the inhibitory effect of IL-1 beta on long term potentiation. A role for JNK. J Biol Chem. 2001; 276:45564–45572. PMID: 11581275.
36. Oger S, Méhats C, Dallot E, Ferré F, Leroy MJ. Interleukin-1beta induces phosphodiesterase 4B2 expression in human myometrial cells through a prostaglandin E2- and cyclic adenosine 3′,5′-monophosphate-dependent pathway. J Clin Endocrinol Metab. 2002; 87:5524–5531. PMID: 12466348.
37. D'Almeida APL, Pacheco de Oliveira MT, de Souza ÉT, de Sá Coutinho D, Ciambarella BT, Gomes CR, Terroso T, Guterres SS, Pohlmann AR, Silva PM, Martins MA, Bernardi A. α-Bisabolol-loaded lipid-core nanocapsules reduce lipopolysaccharide-induced pulmonary inflammation in mice. Int J Nanomedicine. 2017; 12:4479–4491. PMID: 28684908.
38. Roh E, Yun CY, Young Yun J, Park D, Doo Kim N, Yeon Hwang B, Jung SH, Park SK, Kim YB, Han SB, Kim Y. cAMP-binding site of PKA as a molecular target of bisabolangelone against melanocyte-specific hyperpigmented disorder. J Invest Dermatol. 2013; 133:1072–1079. PMID: 23254773.


39. Kim S, Lee J, Jung E, Huh S, Park JO, Lee JW, Byun SY, Park D. Mechanisms of depigmentation by alpha-bisabolol. J Dermatol Sci. 2008; 52:219–222. PMID: 18692366.
40. Avni D, Ernst O, Philosoph A, Zor T. Role of CREB in modulation of TNFalpha and IL-10 expression in LPS-stimulated RAW264.7 macrophages. Mol Immunol. 2010; 47:1396–1403. PMID: 20303596.
41. Wen AY, Sakamoto KM, Miller LS. The role of the transcription factor CREB in immune function. J Immunol. 2010; 185:6413–6419. PMID: 21084670.


42. Shima E, Katsube M, Kato T, Kitagawa M, Hato F, Hino M, Takahashi T, Fujita H, Kitagawa S. Calcium channel blockers suppress cytokine-induced activation of human neutrophils. Am J Hypertens. 2008; 21:78–84. PMID: 18091748.


43. Barreto RSS, Quintans JSS, Amarante RKL, Nascimento TS, Amarante RS, Barreto AS, Pereira EWM, Duarte MC, Coutinho HDM, Menezes IRA, Zengin G, Aktumsek A, Quintans-Júnior LJ. Evidence for the involvement of TNF-α and IL-1β in the antinociceptive and anti-inflammatory activity of Stachys lavandulifolia Vahl. (Lamiaceae) essential oil and (−)-α-bisabolol, its main compound, in mice. J Ethnopharmacol. 2016; 191:9–18. PMID: 27292196.


44. Marongiu L, Donini M, Bovi M, Perduca M, Vivian F, Romeo A, Mariotto S, Monaco HL, Dusi S. The inclusion into PLGA nanoparticles enables α-bisabolol to efficiently inhibit the human dendritic cell pro-inflammatory activity. J Nanopart Res. 2014; 16:2554.


45. Corpas-López V, Morillas-Márquez F, Navarro-Moll MC, Merino-Espinosa G, Díaz-Sáez V, Martín-Sánchez J. (−)-α-Bisabolol, a promising oral compound for the treatment of visceral leishmaniasis. J Nat Prod. 2015; 78:1202–1207. PMID: 26076227.


Fig. 1
Inhibitory effect of α-bisabolol on spontaneous contractions.
(A) Graphic representation of the effect of increasing concentrations of α-bisabolol on strips taken from the pregnant human myometrium. Each column represent the mean of the results of 5 experiments (n=5) conducted on α-bisabolol and the control (DMSO), while the vertical bars indicate the standard error of the mean (±SEM). (B) Typical recording of spontaneous uterine contractions inhibited by α-bisabolol in a concentration-dependent manner. Difference vs. basal; Difference vs. α-bisabolol 1,860 µM; *p<0.05.
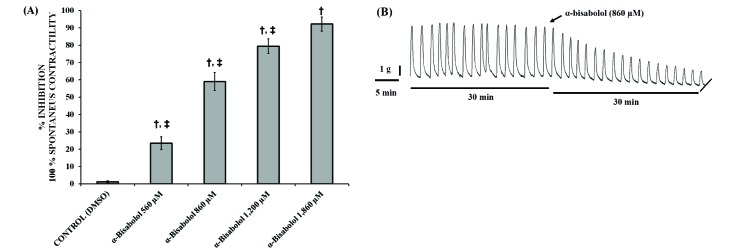
Fig. 2
Intracellular cAMP levels induced by α-bisabolol and forskolin in the pregnant human myometrium.
The different cAMP-levels induced both by α-bisabolol alone and combined with forskolin (10 µM) were obtained at concentrations of 560, 860 and 1,200 µM. Each column represents the mean of the results of 4 experiments (n=4) conducted on α-bisabolol and forskolin, both alone and combined, respectively. The vertical bars indicate the standard error of the mean (±SEM). †Difference vs. Basal; ‡Difference vs. Forskolin (FSK); §Difference vs. α-bisabolol 560+FSK, p<0.05.
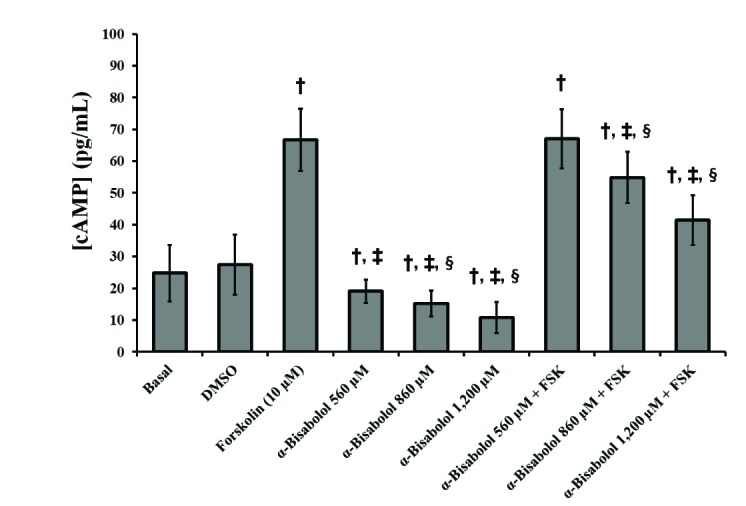
Fig. 3
Effects of α-bisabolol and forskolin on the secretion of TNFα induced by LPS in the pregnant human myometrium.
Explants were cultured both with and without LPS (10 µg/ml), in the presence of solely α-bisabolol at 560, 860 and 1,200 µM, and combined with FSK (10 µM) for 24 h. Control experiments, both basal and DMSO, were run without the addition of LPS or any other compound. Each column represents the mean of the results of 4 experiments (n=4) conducted on both compounds tested, while the vertical bars indicate the standard error of the mean (±SEM). †Difference vs. Basal; ‡Difference vs. LPS (10 µg/ml); §Difference vs. LPS+FSK, p<0.05.
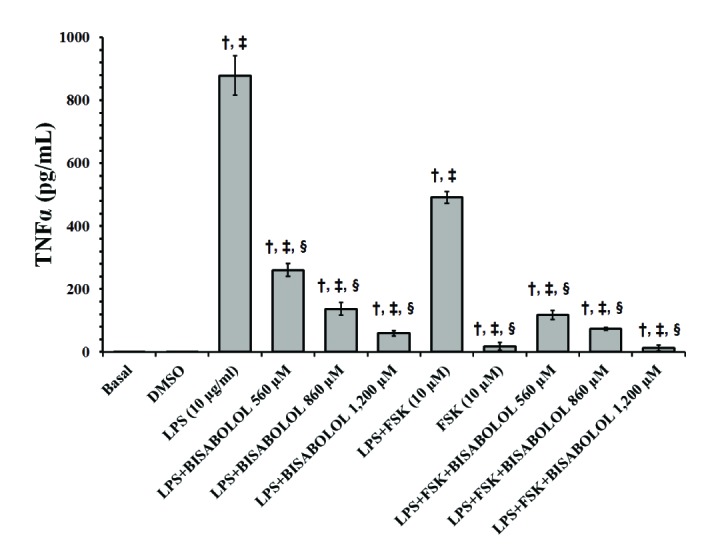
Fig. 4
Effects of α-bisabolol and forskolin on the secretion of IL-1β induced by LPS in the pregnant human myometrium.
Explants were cultured both without and with LPS (10 µg/ml) and in the presence of solely α-bisabolol at 560, 860 and 1,200 µM and combined with FSK (10 µM) for 24 h. Control experiments, both basal and DMSO, were run without the addition of LPS, or any other compound. Each column represents the mean of the results of 4 experiments (n=4) conducted on both compounds tested, while the vertical bars indicate the standard error of the mean (±SEM). †Difference vs. Basal; ‡Difference vs. LPS (10 µg/ml); §Difference vs. LPS+FSK, p<0.05.
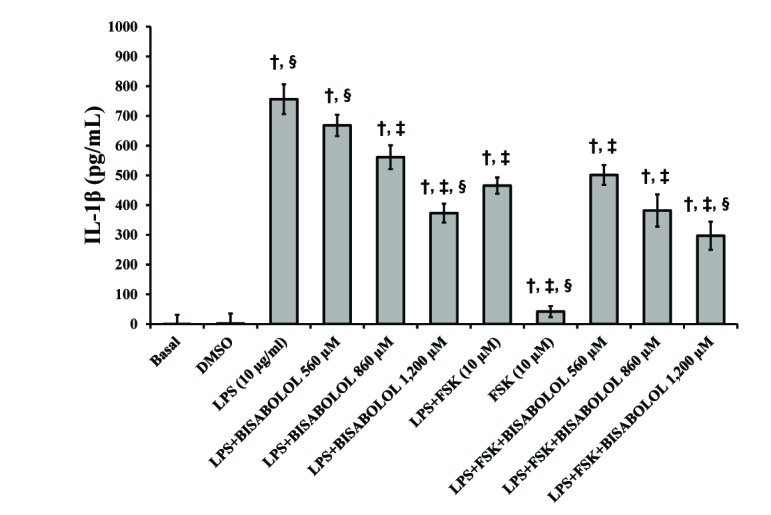
Fig. 5
Effects of α-bisabolol and forskolin on the secretion of IL-10 induced by LPS in the pregnant human myometrium.
Explants were cultured both with and without LPS (10 µg/ml) and in presence of solely α-bisabolol using the concentration of 860 µM both on its own and combined with FSK (10 µM) for 24 h. Control experiments, both basal and DMSO, were run without the addition of LPS or any other compound. Each column represents the mean of the results of 4 experiments (n=4) conducted on both compounds tested, while the vertical bars indicate the standard error of the mean (±SEM). †Difference vs. Basal; ‡Difference vs. LPS (10 µg/ml); §Difference vs. LPS+FSK, p<0.05.
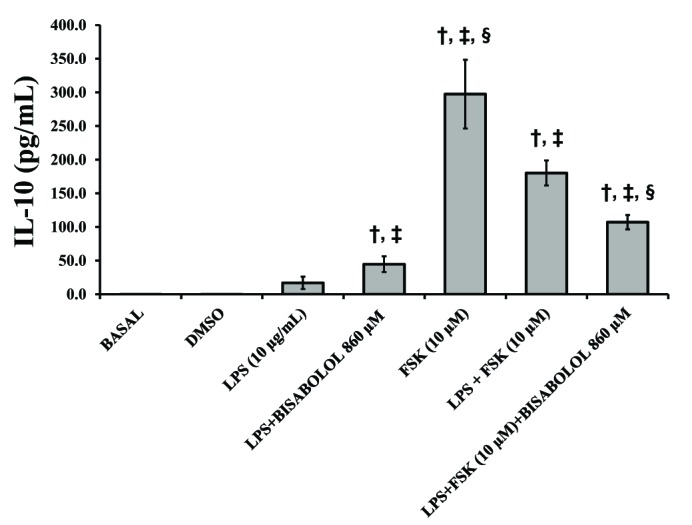