Abstract
Hypotension is one of the potential causes of dizziness. In this review, we summarize the studies published in recent years about the electrophysiological and pharmacological mechanisms of hypotension-induced dizziness and the role of the vestibular system in the control of blood pressure in response to hypotension. It is postulated that ischemic excitation of the peripheral vestibular hair cells as a result of a reduction in blood flow to the inner ear following hypotension leads to excitation of the central vestibular nuclei, which in turn may produce dizziness after hypotension. In addition, excitation of the vestibular nuclei following hypotension elicits the vestibulosympathetic reflex, and the reflex then regulates blood pressure by a dual-control (neurogenic and humoral control) mechanism. In fact, recent studies have shown that peripheral vestibular receptors play a role in the control of blood pressure through neural reflex pathways. This review illustrates the dual-control mechanism of peripheral vestibular receptors in the regulation of blood pressure following hypotension.
The vestibular system is the principal sensory system and has been demonstrated to be responsible for normal spatial orientation, motor coordination, and autonomic regulation. Peripheral vestibular receptors in the inner ear send information of head position and velocity to the central vestibular nuclear complex and the cerebellum through afferent vestibular nerves, and the vestibular nuclear complex then estimates orientation of the head and body. The output of the central vestibular system is generally described in terms of three simple reflexes, the vestibulo-ocular, vestibulospinal, and vestibuloautonomic reflex (VSR) [1]. In fact, the vestibular system influences the function of the autonomic nervous system through the VSR and controls body posture and movement through the vestibulo-ocular and vestibulospinal reflex [2].
Autonomic symptoms, such as nausea, vomiting, tachycardia, vertigo, and tachypnea, can be induced by abnormal stimulation of the vestibular system. Activities of sympathetic nerves are increased by electrical stimulation of the vestibular nerves, and bilateral vestibular dysfunction reduces motion sickness susceptibility. However, the patients with bilateral vestibular loss may be more susceptible to orthostatic hypotension [34567]. The effect of the vestibular system on autonomic functions has been widely studied. However, few studies have investigated the effect of blood pressure on the vestibular function. Stimulation of the vestibular receptors by head movement can affect heart rate, arterial blood pressure, and the baroreceptor reflex [8910111213]. The patients who experience hypotension or have heart diseases often complain of dizziness [141516]. Therefore, decreased blood flow in the peripheral vestibular system may implicate in the pathophysiology of dizziness. In fact, previous studies have suggested that the vestibular activity affects blood pressure and blood pressure also affects the activity of the vestibular system [17181920]. In this review, we delineate the electrophysiological and pharmacological mechanisms of hypotension-induced dizziness and the neural pathways behind the neurogenic and humoral control of the VSR in response to hypotension.
Using the electrophysiological recording in rats with intact labyrinths, Park et al. [17] have demonstrated that hypotension with a 10–30% decrease in blood pressure induced by sodium nitroprusside administration or hemorrhage elicited excitation of type I neurons and inhibition of type II neurons in the bilateral medial vestibular nucleus (MVN). Even though mean arterial pressure is decreased to 50% of control, cerebral blood flow is well controlled by autoregulation [2122]. However, the decrease in cochlea blood flow in the inner ear is in proportion to the reduction in arterial blood pressure [2324]. In addition, a 10% to 30% reduction in arterial blood pressure decreases blood flow in the peripheral vestibular system but does not affect brainstem blood flow [25]. In fact, excitation of type I neurons and inhibition of type II neurons in the bilateral MVN are elicited by hypotension in rats with intact labyrinths. However, in unilateral labyrinthectomized rats, hypotension elicits inhibition of type I neurons and excitation of type II neurons ipsilateral to the lesion and induces excitation of type I neurons and inhibition of type II neurons contralateral to the lesion [17]. The responses of neurons in the MVN contralateral to the lesion are produced by commissural connections to the MVN ipsilateral to the lesion. These results suggest that hypotension alters the central vestibular neuronal activity by activating the peripheral vestibular receptors rather than the central vestibular nuclear complex itself.
According to previous studies, it remains unclear how reduction of blood flow activates the peripheral vestibular receptors, but one assumption is that ischemic damage to the hair cells in the peripheral vestibular system is caused by decreased blood flow in the inner ear. In fact, the level of neurotransmitter glutamate is increased during cochlear ischemia, and excess glutamate causes acute excitotoxicity in the inner hair cells [26]. In addition, a reduction of inner ear blood flow due to hypotension causes ischemic excitation of the peripheral vestibular hair cells, resulting in activation of the central vestibular nuclei, which may in turn produce dizziness. Although the central vestibular nuclei are vulnerable to ischemia [27], the central vestibular nuclei may not be involved in dizziness after hypotension because loss of bilateral peripheral vestibular receptors cannot induce excitation of the central vestibular nuclei after hypotension.
Hypotension induces expression of the c-Fos protein in the preoptic area, hypothalamus, and medulla, which are involved in regulation of body fluid [2829]. Sodium nitroprusside-induced hypotension increases expression of the c-Fos protein in the vestibular nuclear complex, particularly in the MVN, in control animals. However, in unilateral labyrinthectomized animals, reduction in the c-Fos protein expression is more pronounced in the vestibular nuclei ipsilateral than contralateral to the lesion after hypotension. Furthermore, bilateral labyrinthectomy completely suppresses the expression of the c-Fos protein in the bilateral vestibular nuclei after hypotension [18]. The pattern of phosphorylated extracellular signal-regulated kinase (pERK) expression in the vestibular nuclei after hypotension is similar to that of c-Fos protein expression [30]. These results are consistent with those obtained in electrophysiological studies.
Excitation of peripheral vestibular receptors due to hypotension is transmitted to the vestibular nuclei through neurotransmitters. Glutamate, aspartate, glycine, alanine, taurine, and gamma-aminobutyric acid (GABA) are the main neurotransmitters in the central nervous system [31]. In particular, glutamate is an excitatory neurotransmitter of afferent fibers in the peripheral vestibular system [32]. GABA is an inhibitory neurotransmitter of commissural connections between bilateral vestibular nuclei and is involved in the inhibitory cerebellar inputs [33]. Taurine is a possible modulator of GABA function [34]. To understand the signaling pathway in the vestibular nuclei after hypotension, Li et al. [35] used microdialysis and high performance liquid chromatography to measure the levels of specific amino acids in the MVN involved in regulation of vestibular nuclear activity after hypotension, and the results revealed increased glutamate release but decreased GABA and taurine release in the MVN following hypotension. In unilateral labyrinthectomized rats, the levels of glutamate, GABA, and taurine were unchanged in the MVN ipsilateral to the lesion; however, in the MVN contralateral to the lesion, glutamate release was increased, and GABA and taurine release was decreased following hypotension.
Glutamate, a neurotransmitter in brain, has different types of receptors, such as ionotropic receptors (alpha-amino-3-hydroxy-5-methylisoxazole-4-propionic acid [AMPA], N-methyl-D-aspartate [NMDA], and kainic acid) and metabotropic receptors (metabotropic glutamate receptor 1 [GluR1] to metabotropic glutamate receptor 11). Ischemic damage caused by hypotension to the hair cells in the peripheral vestibular system increases glutamate release in the vestibular afferent nerves, and the released glutamate in turn activates its receptors to excite the vestibular nuclei. To determine whether the glutamate receptors are involved in this process, Li et al. [36] investigated the signaling pathway of glutamate and its receptors in the central vestibular nuclei following hypotension in conscious rats. It was found that microinjection of NMDA or AMPA into the lateral ventricle increased c-Fos protein expression in the bilateral MVN. However, after microinjection of 6-cyano-7-nitroquinoxaline-2, 3-dione (CNQX) or dizocilpine (MK801) into the lateral ventricle, little c-Fos protein was expressed in the bilateral MVN following hypotension. These results suggest that excitatory afferent signals from the peripheral vestibular receptors release glutamate into postsynaptic neurons in the central vestibular nuclear complex in response to decreased blood pressure. Choi et al. [37] investigated by western blotting the expression of subunits of glutamate receptors, AMPA and NMDA, in the MVN following hypotension. They found that the excitatory signals from the peripheral vestibular receptors to the vestibular nuclei were transmitted through NR2B subunit of NMDA receptors and the GluR1 subunit of AMPA receptors in the vestibular system [3637].
In the VSR, vestibular nuclear neurons, which are excited by neurotransmitters, transmit afferent signals to the STN [13]. The STN also receives afferent signals from several other visceral organs to control autonomic functions in the body. Blood pressure is maintained by a complex network of physiological systems, such as the baroreceptor reflex and VSR [103839]. The baroreceptor reflex maintains blood pressure by a feedback control system during hemorrhage and physical movement [40]. Baroreceptors are located in the carotid sinus and aortic arch. The carotid sinus transmits afferent signals to the STN through the glossopharyngeal nerve, and the aortic arch sends the signals to the STN through the vagus nerve. Neurons from the STN transmit the signals to the caudal ventrolateral medullary nucleus (CVLM) and nucleus ambiguus. Neurons from the CVLM transmit the inhibitory signals to the rostral ventrolateral medullary nucleus (RVLM). RVLM, an area containing presympathetic neurons, is the center of the sympathetic nervous system and innervates the intermediolateral cell column (IMC) in the spinal cord.
The VSR controls blood pressure through activation of the sympathetic nervous system that is elicited by vestibular stimulation [212]. The STN, CVLM, and RVLM receive afferent inputs from the inferior vestibular nucleus and the caudal portion in the MVN [4142]. The STN also receives primary inputs from the cardiovascular, digestive, and respiratory system [43]. In addition, direct projections from the inferior vestibular nucleus and MVN to both the STN and the dorsal motor nucleus in the vagus nerve were observed in rabbits and rats, and the results suggest that vestibular inputs converge with baroreceptor reflex inputs in the STN. The STN integrates afferent signals from baroreceptors and vestibular receptors to control blood pressure [204144]. Jiang et al. [45] measured pERK expression in the STN in conscious rats that had undergone bilateral labyrinthectomy and/or sinoaortic denervation (SAD) following hypotension. The results showed that acute hypotension significantly increased expression of pERK in the STN in the control group. Hypotension also increased pERK expression in the bilateral labyrinthectomy, SAD, and combination of labyrinthectomy and denervation groups; however, the increase of pERK expression in the three experimental groups was smaller than that in the control group. The SAD group showed a higher expression of pERK than did the bilateral labyrinthectomy group. In the bilateral labyrinthectomy and SAD groups, pERK-immunoreactive neurons were mainly localized in the caudal region of the STN.
A recent study [20] also found that expression of the c-Fos protein was significantly increased in the STN in the control group following hypotension. However, c-Fos protein expression in the bilateral labyrinthectomy, SAD, or combination of labyrinthectomy and denervation group was significant decreased compared with that in the control group. The combination of labyrinthectomy and denervation group showed the least expression of c-Fos among the three experimental groups, and c-Fos protein expression in the bilateral labyrinthectomy group was higher than that in the SAD group. These results suggest that the function of the STN can be modulated by the vestibular system.
The signaling pathway from the vestibular nuclei to the STN was also pharmacologically investigated by Li et al. [46]. It was found that pretreatment with glutamate receptor antagonists (MK801 or CNQX) in the MVN abolished the increase in expression of both c-Fos and pERK proteins in the STN following hypotension. Moreover, expression of both pERK and c-Fos proteins in the STN was increased after microinjection of glutamate receptor agonists (AMPA or NMDA) into the MVN. These results suggest that NMDA and AMPA receptors are involved in transmission of excitatory signals from the vestibular nuclei to the STN following hypotension.
The STN receives afferent signals from the vestibular nuclei as well as the baroreceptors and transmits the signals to the RVLM through the CVLM in the VSR and the baroreceptor reflex. Doba and Reis first reported that the vestibular system plays a role in cardiovascular responses to postural movement [3]. Activity of the sympathetic nerve is increased by electrical or head rotatory stimulation of the peripheral vestibular receptors in cats [474849]. Many reports suggest that the neural circuit in the VSR is independent of the neural circuit in the baroreceptor reflex in various species [50]. The RVLM, the most essential regulation center of the cardiac sympathetic nerve and sympathetic vasoconstrictor nerve, receives information from the STN and CVLM. The RVLM integrates various information and regulates tonic activity of the sympathetic nerve by its fibers bound to presympathetic neurons that innervate the IMC in the spinal cord [51].
Lan et al. [52] observed c-Fos protein expression in the RVLM in conscious rats that had undergone bilateral labyrinthectomy and/or SAD. It was found that expression of the c-Fos protein was significantly increased in the RVLM in the control group following hypotension; however, c-Fos protein expression in the bilateral labyrinthectomy, SAD, or combination of labyrinthectomy and denervation groups was significantly decreased compared with that in the control group. They also found that the expression of pERK significantly increased in the RVLM in the control group following hypotension [38]. Hypotension also increased expression of pERK in the bilateral labyrinthectomy, SAD, or combination of labyrinthectomy and denervation groups, although the increase in the three experimental groups was smaller than that in the control group. The bilateral labyrinthectomy group showed a higher pERK expression than did the SAD group. Furthermore, glutamate release was significantly increased in the RVLM in the control, bilateral labyrinthectomy, and SAD groups following hypotension. The bilateral labyrinthectomy group showed a higher glutamate release than did the SAD group. These results indicate that the vestibular receptors have a less powerful role in regulating pERK expression and glutamate release in the RVLM following hypotension than do the baroreceptors; however, the vestibular receptors play an important role in the RVLM and are involved in blood pressure maintenance.
The RVLM transmits efferent signals to the IMC in order to produce sympathetic outputs. Sympathetic preganglionic neurons in the spinal cord are located in the IMC. Nerve fibers from the IMC in the spinal cord directly innervate the adrenal gland or the internal organs through postganglionic neurons. Cardiac sympathetic nerves and vasoconstrictor nerves participate in the regulation of cardiovascular activity.
It has been reported that hypotension excites RVLM neurons through vestibular receptors in SAD animals [38]. It is postulated that hypotension activates the VSR by exciting the sympathetic nerves through the IMC in the spinal cord. Interestingly, Lu et al. [53] and Park et al. [54] found that hypotension increased c-Fos and pERK protein expression in the IMC of T4-T7 spinal cord in conscious rats with SAD. These findings demonstrate that hypotension increases sympathetic nerve activity through the vestibular nuclei and RVLM.
Additionally, it has been demonstrated that glutamate plays an important role in the signaling pathway underlying the transmission of neural signals from the RVLM to the IMC in response to hypotension [5354]. In fact, pretreatment with microinjection of glutamate receptor antagonists (MK801 or CNQX) into the MVN or RVLM prevents the increase of pERK or c-Fos protein expression in the IMC after hypotension. These results indicate that the activated transmission of neural signals from the vestibular receptors to the IMC may compensate for decreased blood pressure following hypotension through the NMDA and AMPA receptors of glutamate in the IMC. These neural networks modulate blood pressure by direct stimulation of the sympathetic nerves to the cardiovascular system following hypotension. This may be considered as a neurogenic or fast control of blood pressure in the VSR.
The IMC transmits sympathetic outputs to the adrenal medulla and visceral organs through the peripheral sympathetic nerves. The adrenal medulla releases epinephrine into the bloodstream, and the released epinephrine plays an important role in the control of blood pressure. Recent studies have investigated the role of blood epinephrine in the humoral control of blood pressure [5354]. It was found that blood epinephrine levels were elevated after hypotension, and microinjection of glutamate agonists (NMDA or AMPA) into the MVN or RVLM also increased the blood epinephrine levels. However, pretreatment with microinjection of glutamate antagonists (MK801 or CNQX) into the MVN or RVLM abolished the increase in blood epinephrine level following hypotension. Increased blood epinephrine following hypotension may result in excitation of the sympathetic nerves in the adrenal medulla. Therefore, after hypotension, the VSR may regulate blood pressure through increased epinephrine release from the adrenal medulla, and the released epinephrine may be involved in the humoral or delayed control of blood pressure in the vestibulosympathetic reflex (Fig. 1) [54].
In conclusion, hypotension elicits the VSR, which in turn regulates blood pressure by neurogenic and humoral control mechanisms. In the neurogenic control, the peripheral vestibular receptors are excited by hypotension, and the excitatory signals from the vestibular receptors are transmitted to the T4-T7 IMC through the MVN and RVLM. Excitation of the peripheral sympathetic nerves then increases blood pressure. This neural circuit is involved in the rapid response in the VSR. In the humoral control, an increased epinephrine release from the adrenal medulla elevates blood pressure. This pathway is involved in the delayed response to hypotension in the VSR. The VSR, therefore, shows a dual control mechanism in the regulation of blood pressure in response to hypotension.
Notes
References
1. Wilson VJ, Jones GM. Mammalian vestibular physiology. New York: Plenum press;1979.
2. Yates BJ. Vestibular influences on the sympathetic nervous system. Brain Res Brain Res Rev. 1992; 17:51–59. PMID: 1638275.


3. Doba N, Reis DJ. Role of the cerebellum and the vestibular apparatus in regulation of orthostatic reflexes in the cat. Circ Res. 1974; 34:9–18. PMID: 4543723.


4. Yates BJ, Siniaia MS, Miller AD. Descending pathways necessary for vestibular influences on sympathetic and inspiratory outflow. Am J Physiol. 1995; 268:R1381–R1385. PMID: 7611512.


5. Park BR, Kim MS, Lee MY, Kim YK, Choi SC, Nah YH. Effects of galvanic stimulation of the mastoid process on the gastric motility induced by caloric stimulation. Auris Nasus Larynx. 1999; 26:263–268. PMID: 10419033.


6. Graybiel A, Miller EF 2nd, Newsom BD, Kennedy RS. The effect of water immersion on perception of the oculogravic illusion in normal and labyrinthine-defective subjects. Acta Otolaryngol. 1968; 65:599–610. PMID: 5706030.


7. Kennedy RS, Graybiel A, McDonough RC, Beckwith FD. Symptomatology under storm conditions in the North Atlantic in control subjects and in persons with bilateral labyrinthine defects. Acta Otolaryngol. 1968; 66:533–540. PMID: 5732654.


8. Kolev OI, Tibbling L. Vestibular and cardiac reactions to open-sea exposure. J Vestib Res. 1992; 2:153–157. PMID: 1342389.
9. Normand H, Etard O, Denise P. Otolithic and tonic neck receptors control of limb blood flow in humans. J Appl Physiol (1985). 1997; 82:1734–1738. PMID: 9173934.
10. Convertino VA. Interaction of semicircular canal stimulation with carotid baroreceptor reflex control of heart rate. J Vestib Res. 1998; 8:43–49. PMID: 9416588.


11. Yates BJ, Aoki M, Burchill P, Bronstein AM, Gresty MA. Cardiovascular responses elicited by linear acceleration in humans. Exp Brain Res. 1999; 125:476–484. PMID: 10323294.


12. Yates BJ, Miller AD. Properties of sympathetic reflexes elicited by natural vestibular stimulation: implications for cardiovascular control. J Neurophysiol. 1994; 71:2087–2092. PMID: 7931504.


13. Gotoh TM, Fujiki N, Matsuda T, Gao S, Morita H. Roles of baroreflex and vestibulosympathetic reflex in controlling arterial blood pressure during gravitational stress in conscious rats. Am J Physiol Regul Integr Comp Physiol. 2004; 286:R25–R30. PMID: 14500268.


15. Ohashi N, Imamura J, Nakagawa H, Mizukoshi K. Blood pressure abnormalities as background roles for vertigo, dizziness and disequilibrium. ORL J Otorhinolaryngol Relat Spec. 1990; 52:355–359. PMID: 2274319.


16. Yang CS, Young YH. Clinical investigation on hypotensive patients with vertigo. Eur Arch Otorhinolaryngol. 2006; 263:804–808. PMID: 16816934.


17. Park BR, Kim MS, Kim JH, Jin YZ. Effects of acute hypotension on neuronal activity in the medial vestibular nuclei of rats. Neuroreport. 2001; 12:3821–3824. PMID: 11726802.


18. Kim MS, Hyo Kim J, Kry D, Ae Choi M, Ok Choi D, Gon Cho B, Jin YZ, Ho Lee S, Park BR. Effects of acute hypotension on expression of cFos-like protein in the vestibular nuclei of rats. Brain Res. 2003; 962:111–121. PMID: 12543461.


19. Lee JO, Park SH, Kim HJ, Kim MS, Park BR, Kim JS. Vulnerability of the vestibular organs to transient ischemia: implications for isolated vascular vertigo. Neurosci Lett. 2014; 558:180–185. PMID: 24269984.


20. Jiang X, Li LW, Lan Y, Yang YZ, Jin GS, Kim MS, Park BR, Jin YZ. Comparative analysis of vestibular receptor and baroreceptor inputs to the nucleus tra rats. Neurosci Lett. 2014; 563:70–74. PMID: 24486893.
21. Bünemann L, Jensen KA, Riisager S, Thomsen LJ. Cerebral blood flow and metabolism during hypotension induced with sodium nitroprusside and metoprolol. Eur J Anaesthesiol. 1991; 8:197–201. PMID: 1874217.
22. Hamaguchi M, Ishibashi T, Katsumata N, Mitomi A, Imai S. Effects of sodium nitroprusside (MR7S1) and nitroglycerin on the systemic, renal, cerebral, and coronary circulation of dogs anesthetized with enflurane. Cardiovasc Drugs Ther. 1992; 6:611–622. PMID: 1292581.


23. Hasegawa M, Yokoyama K, Kobayashi N, Okamoto A, Tamura T, Watanabe I. Blood pressure and cochlear blood flow in the guinea pig. Acta Otolaryngol. 1989; 107:413–416. PMID: 2756833.


24. Preckel MP, Dégoute CS, Dubreuil C, Boulud B, Tassard AM, Banssillon V. Effects of buflomedil and naftidrofuryl on the human cochlear microcirculation measured by laser-Doppler. Rev Laryngol Otol Rhinol (Bord). 1995; 116:69–72. PMID: 7644852.
25. Angelborg C, Larsen HC. Blood flow in the peripheral vestibular system. J Otolaryngol. 1985; 14:41–43. PMID: 3877816.
26. Pujol R, Puel JL, Gervais d'Aldin C, Eybalin M. Pathophysiology of the glutamatergic synapses in the cochlea. Acta Otolaryngol. 1993; 113:330–334. PMID: 8100108.


27. Yamamoto K, Kubo T, Matsunaga T. Effects of asymmetric vertebral blood flow upon the vestibulo-ocular reflex of the rabbit. Arch Otorhinolaryngol. 1985; 241:195–202. PMID: 3872117.


28. Badoer E, McKinley MJ, Oldfield BJ, McAllen RM. Distribution of hypothalamic, medullary and lamina terminalis neurons expressing Fos after hemorrhage in conscious rats. Brain Res. 1992; 582:323–328. PMID: 1393554.


29. Krukoff TL, MacTavish D, Harris KH, Jhamandas JH. Changes in blood volume and pressure induce c-fos expression in brainstem neurons that project to the paraventricular nucleus of the hypothalamus. Brain Res Mol Brain Res. 1995; 34:99–108. PMID: 8750865.


30. Kim MS, Choi DO, Choi MA, Kim JH, Kim KY, Lee MY, Rhee JK, Chun SW, Park BR. Immunohistochemical detection of phosphorylated form of extracellular signal-regulated kinase 1/2 in rat vestibular nuclei following hemorrhagic hypotension. Neurosci Lett. 2004; 360:49–52. PMID: 15082176.


31. Li H, Godfrey DA, Rubin AM. Quantitative distribution of amino acids in the rat vestibular nuclei. J Vestib Res. 1994; 4:437–452. PMID: 7850040.


32. Smith PF, de Waele C, Vidal PP, Darlington CL. Excitatory amino acid receptors in normal and abnormal vestibular function. Mol Neurobiol. 1991; 5:369–387. PMID: 1668393.


33. Smith PF, Darlington CL, Hubbard JI. Evidence for inhibitory amino acid receptors on guinea pig medial vestibular nucleus neurons in vitro. Neurosci Lett. 1991; 121:244–246. PMID: 1850504.


34. Schousboe A, Pasantes-Morales H. Potassium-stimulated release of [3H]taurine from cultured GABAergic and glutamatergic neurons. J Neurochem. 1989; 53:1309–1315. PMID: 2769270.
35. Li XL, An Y, Jin QH, Kim MS, Park BR, Jin YZ. Changes of some amino acid concentrations in the medial vestibular nucleus of conscious rats following acute hypotension. Neurosci Lett. 2010; 477:11–14. PMID: 20399837.


36. Li XL, Nian B, Jin Y, Li LW, Jin GS, Kim MS, Park BR, Jin YZ. Mechanism of glutamate receptor for excitation of medial vestibular nucleus induced by acute hypotension. Brain Res. 2012; 1443:27–33. PMID: 22305141.


37. Choi MA, Lee JH, Hwang JH, Choi SJ, Kim MS, Park BR. Signaling pathway of glutamate in the vestibular nuclei following acute hypotension in rats. Brain Res. 2008; 1229:111–117. PMID: 18639534.


38. Lan Y, Lu HJ, Jiang X, Li LW, Yang YZ, Jin GS, Park JY, Kim MS, Park BR, Jin YZ. Analysis of the baroreceptor and vestibular receptor inputs in the rostral ventrolateral medulla following hypotension in conscious rats. Korean J Physiol Pharmacol. 2015; 19:159–165. PMID: 25729278.


39. Kumagai H, Oshima N, Matsuura T, Iigaya K, Imai M, Onimaru H, Sakata K, Osaka M, Onami T, Takimoto C, Kamayachi T, Itoh H, Saruta T. Importance of rostral ventrolateral medulla neurons in determining efferent sympathetic nerve activity and blood pressure. Hypertens Res. 2012; 35:132–141. PMID: 22170390.


40. Spyer KM. Neural organisation and control of the baroreceptor reflex. Rev Physiol Biochem Pharmacol. 1981; 88:24–124. PMID: 7010509.


41. Balaban CD, Beryozkin G. Vestibular nucleus projections to nucleus tractus solitarius and the dorsal motor nucleus of the vagus nerve: potential substrates for vestibulo-autonomic interactions. Exp Brain Res. 1994; 98:200–212. PMID: 8050507.


42. Holstein GR, Friedrich VL Jr, Kang T, Kukielka E, Martinelli GP. Direct projections from the caudal vestibular nuclei to the ventrolateral medulla in the rat. Neuroscience. 2011; 175:104–117. PMID: 21163335.


43. Kalia M, Mesulam MM. Brain stem projections of sensory and motor components of the vagus complex in the cat: II. Laryngeal, tracheobronchial, pulmonary, cardiac, and gastrointestinal branches. J Comp Neurol. 1980; 193:467–508. PMID: 7440778.


44. Yates BJ, Grélot L, Kerman IA, Balaban CD, Jakus J, Miller AD. Organization of vestibular inputs to nucleus tractus solitarius and adjacent structures in cat brain stem. Am J Physiol. 1994; 267:R974–R983. PMID: 7524372.


45. Jiang X, Lan Y, Jin YZ, Park JY, Park BG, Ameer AN, Park BR. Effect of vestibulosympathetic reflex and baroreflex on expression of pERK in the aucleus tractus solitarius following acute hypotension in conscious rats. Korean J Physiol Pharmacol. 2014; 18:353–358. PMID: 25177169.
46. Li LW, Jin GS, Yang YZ, Ameer AN, Kim MS, Park BR, Jin YZ. Effect of glutamate on the vestibulo-solitary projection after sodium nitroprusside-induced hypotension in conscious rats. Korean J Physiol Pharmacol. 2015; 19:275–281. PMID: 25954134.


47. Kerman IA, McAllen RM, Yates BJ. Patterning of sympathetic nerve activity in response to vestibular stimulation. Brain Res Bull. 2000; 53:11–16. PMID: 11033203.


48. Kerman IA, Yates BJ, McAllen RM. Anatomic patterning in the expression of vestibulosympathetic reflexes. Am J Physiol Regul Integr Comp Physiol. 2000; 279:R109–R117. PMID: 10896871.


49. Ray CA, Carter JR. Vestibular activation of sympathetic nerve activity. Acta Physiol Scand. 2003; 177:313–319. PMID: 12609001.


50. Radtke A, Popov K, Bronstein AM, Gresty MA. Evidence for a vestibulo-cardiac reflex in man. Lancet. 2000; 356:736–737. PMID: 11085696.


51. Guyenet PG, Haselton JR, Sun MK. Sympathoexcitatory neurons of the rostroventrolateral medulla and the origin of the sympathetic vasomotor tone. Prog Brain Res. 1989; 81:105–116. PMID: 2616776.
52. Lan Y, Yang YZ, Jiang X, Li LW, Jin GS, Kim MS, Park BR, Jin YZ. Additive role of the vestibular end organ and baroreceptors on the regulation of blood pressure in rats. Korean J Physiol Pharmacol. 2013; 17:367–373. PMID: 23946697.


53. Lu HJ, Li MH, Li MZ, Park SE, Kim MS, Jin YZ, Park BR. Functional connections of the vestibulo-spino-adrenal axis in the control of blood pressure via the vestibulosympathetic reflex in conscious rats. Korean J Physiol Pharmacol. 2015; 19:427–434. PMID: 26330755.


54. Park SE, Jin YZ, Park BR. Dual control of the vestibulosympathetic reflex following hypotension in rats. Korean J Physiol Pharmacol. 2017; 21:675–686. PMID: 29200911.


Fig. 1
A block diagram shows the pathway of the neurogenic and humoral control in the vestibulosympathetic reflex.
The solid line is based on the results obtained in our studies. VNC, Vestibular nuclear complex; RVLM, Rostral ventrolateral medullary nuclei; IMC, Intermediolateral cell column of the spinal cord; AM, Adrenal medulla; NTS, Nucleus tractus solitarius; CVLM, Caudal ventrolateral medullary nucleus [54 with permission].
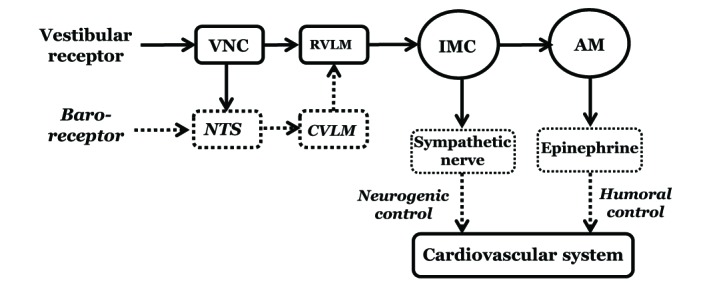