INTRODUCTION
Differentiated thyroid cancer (DTC) is known to be one of the most curable cancers, and radioactive iodine (RAI) treatment has been contributed to its excellent prognosis. Thus, still a large number of DTC has been treated with RAI therapy after total thyroidectomy, depending on the risk stratification of current guidelines [
12].
During the initial RAI treatment in DTC, a star-shaped region of intense
131I uptake can be observed on whole body scans (WBSs) sometimes. This phenomenon is called a ‘star artifact’ (
Fig. 1) and has been reported to be caused by the penetration of the collimator's septa by the γ-rays from the high uptake of RAI in the thyroid [
34]. Although this phenomenon is often seen in clinics, there has been no investigation into its clinical implications or outcomes.
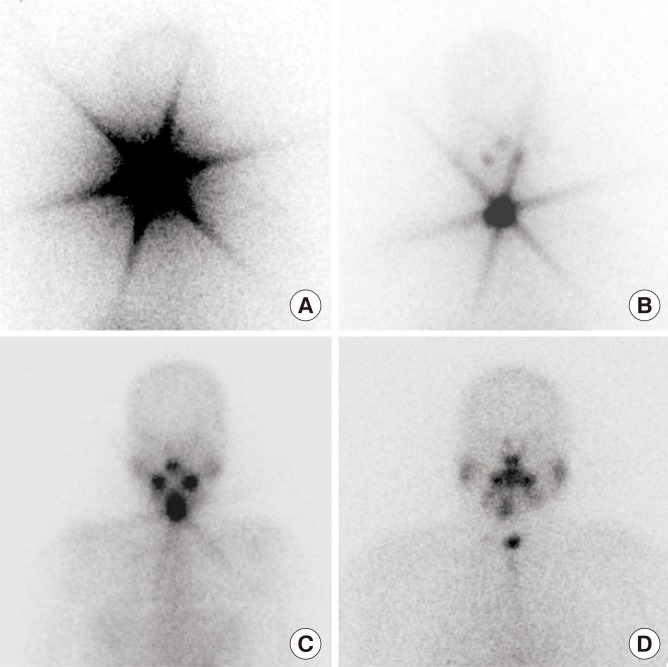 | Fig. 1Representative 131I whole body scan images (A–C) with and (D) without star artifact after 1st 1.1 GBq of radioactive iodine treatment.
|
There are a few theories regarding the high uptake of RAI that causes star artifacts. First of all, it could be caused by large amounts of remnant thyroid tissue after surgery. It was reported that patients with large amounts of remnant thyroid tissue had a higher uptake of RAI, compared with those who did not [
5]. However, thyroid tissues with high expression of the sodiumiodine symporter (NIS) also could cause an excessively high uptake of RAI; active transport by the NIS leads to iodide uptake across the membranes of thyroid cancer cells [
6]. Therefore, it is still questionable whether the presence of a star artifact is a poor prognostic marker representing a large amount of remnant thyroid or residual tumor that indicates the need for a more thorough evaluation, or a good prognostic marker reflecting better treatment efficacy. If it is a good prognostic marker, a relatively lower dose of second RAI ablation might be sufficient or even a diagnostic WBS might be not required in patients with a star artifact. Considering that the usefulness of the diagnostic WBS or second RAI treatment after initial low dose of RAI remnant ablation treatment is still unclear [
1], determining the prognostic value of the star artifacts would be helpful for the planning of further managements.
Therefore, in this study, we investigated the clinical implications of the star artifact observed after initial RAI therapy by comparing the success rate of remnant ablation and long-term recurrence rate between patients with and without a star artifact.
Go to :

METHODS
Study population
We reviewed 720 consecutive patients who underwent total thyroidectomy and RAI therapy for the treatment of DTC between January 2005 and December 2007 in the Seoul National University Hospital. Among them, 72 patients who initially received 131I dose over 1.1 GBq (30 mCi), and 12 patients showing distant metastasis at the initial WBS were excluded. The remaining 636 patients (125 men [19.7%] and 511 women [80.3%]; mean age, 59.0±11.0 years) who received 1.1 GBq of 131I were analyzed in this study. The median duration of the follow-up was 8.0 years (interquartile range [IQR], 1.0 to 10.0). The study was approved and a patient's informed consent was waived by the Institutional Review Board (IRB) of the Seoul National University Hospital (IRB number 1104-080-359).
RAI ablation and definition of a ‘star artifact’ on WBS images
For
131I RAI therapy, 608 patients discontinued thyroxine replacement at least 4 weeks prior to
131I administration and were switched to triiodothyronine, which was discontinued 2 weeks before
131I administration. Iodine restriction diet was started 2 weeks before
131I administration. Serum samples for thyroglobulin (Tg) and anti-thyroglobulin antibody (TgAb) measurement were collected 1 hour before
131I administration. The other 28 patients underwent recombinant human thyroid stimulating hormone (rhTSH; Thyrogen, Genzyme Corp., Cambridge, MA, USA) therapy; after adhering to iodine restriction diet for 2 weeks, rhTSH was administered (0.9 mg intramuscular) for 2 consecutive days. Tg and TgAb levels at the time of ablation were measured on the 5th day after the first rhTSH injection, which corresponded to the 3rd day after
131I administration [
7]. Thyroxine was stopped the day before the 1st administration of rhTSH, and started again the day after WBS. For both stimulation methods, serum levels of thyroid stimulating hormone (TSH) over 30 IU/mL were considered sufficient.
A post-therapy WBS was acquired 2 days after 131I administration using a large field-of-view gamma camera (ON 410, Ohio Nuclear, Solon, OH, USA) with a high-energy general purpose collimator. A 20% symmetric window was centered at 364 keV. A total of 100,000 counts were accumulated in each case, and anterior images of the neck, chest, and abdomen were obtained.
The presence of star artifacts was assessed according to the pattern of
131I uptake in the remnant thyroid on WBS after the initial RAI administration. As shown in
Fig. 1, the star artifact in our study was defined as a star-shaped region of intense uptake of
131I, reviewed by two independent physicians (S.H.K. and J.A.L.). The final consensus for the discrepant cases was made after discussion with another physician (Y.J.P.).
Follow-up protocols and the definition of recurrence
In some patients (
n=50) who showed no or faint RAI uptake only in the thyroid bed with stimulated thyroglobulin (sTg) <0.2 ng/mL at the time of initial ablation, neck ultrasonography was repeated and the Tg level on TSH suppression was measured 6 to 12 months after initial ablation. Otherwise, 2nd WBS using 0.15 to 1.1 GBq (4 to 30 mCi) of
131I was performed (
n=586). Regular follow-up included a physical examination, measurement of TSH, free thyroxine, total triiodothyronine, serum Tg and TgAb levels every 6 to 12 months, and neck ultrasonography every 1 to 3 years. Levothyroxine was administered to achieve serum TSH levels during the first 5 to 10 years after initial treatment [
8].
Clinical recurrence was defined as a reappearance of pathologically proven malignant tissue and/or an appearance of definitive metastatic lesions in other organs by WBS, positron emission tomography computed tomography (PET-CT), or CT, assessed by radiologic specialists [
8].
Serum Tg and TgAb measurement
Serum Tg was measured with an immunoradiometric assay (RIA Tgplus, BRAHMS GmbH, Hennigsdorf, Germany). The analytical sensitivity read at the optimal curve was 0.08 ng/mL, and the functional assay sensitivity (20% interassay coefficient of variation) was 0.2 ng/mL. TSH level was measured with an immunoradiometric assay (TSH-CTK-3, DiaSorin, Saluggia, Italy). The analytical sensitivity was 0.04 mU/L, and the functional assay sensitivity was 0.07 mU/L. TgAb was measured with a radioimmunometric assay (anti-Tg, BRAHMS GmbH). The analytical sensitivity was 5.5 U/mL, and the functional assay sensitivity was <20 U/mL. TgAb was defined as positive when the levels were >60 U/mL.
Statistical analysis
Categorical variables are presented as numbers and percentages, and continuous variables are expressed as mean±standard deviation (SD) for normal distribution, or medians with IQR for nonnormal distribution. Pearson chi-square test was used to compare categorical variables. Continuous variables were compared using Student t test for normally distributed variables and the Mann-Whitney U test for nonnormal distributions. Recurrence-free survival (RFS) curves were constructed using the Kaplan-Meier method and compared using the log-rank test. All P values were two-sided with P<0.05 considered statistically significant. All statistical analyses were performed with SPSS software version 22.0 (IBM Co., Armonk, NY, USA).
Go to :

DISCUSSION
In this study, we found that star artifacts resulting from the strong uptake of
131I could be a good prognostic factor reflecting higher ablation success rates after the initial RAI therapy or long-term prognosis in patients with sTg level ≤2 ng/dL. The sTg level has been thought a well-known predictive marker for recurrence in DTC because it could reflect the amount of residual mass of thyroid, including cancer, and patients with higher sTg levels have shown a higher probability of recurrence when they are negative for TgAb [
9]. In cases with high sTg levels, star artifacts might indicate a large amount of residual thyroid mass; if that were the case, the presence of a star artifact would be a poor prognostic factor. On the other hand, in cases with low sTg levels, star artifacts might instead reflect an increased RAI uptake by the residual thyroid tissue. If the expression of NIS in the residual tissue is upregulated, the RAI uptake would be greater even if the residual mass was lower. In such cases, the star artifact could be a good prognostic factor to predict a successful ablation after RAI therapy.
In the study, the recurrence rate was significantly higher in patients with sTg levels >2 ng/mL than in those with sTg levels ≤2 ng/mL, as previously reported [
10]. Within the subgroup of patients with sTg levels >2 ng/mL, star artifacts was not associated with the rate of recurrence, and it was same when the analysis was performed in patients with a sTg value of 2 to 10 ng/mL. In other words, in patients with higher Tg levels, which may represent a larger residual mass, it is difficult to conclude whether the star artifact indicates a high RAI uptake capability or large amount of residual thyroid. It is suspected that the prognostic power of high sTg levels might be strong enough to mask the effects of the star artifact. On the other hand, in patients with sTg levels ≤2 ng/mL, RFS and the proportion of negative subsequent WBS was significantly higher in patients with star artifacts than in those without. This implicates that the presence of a star artifact in patients with low sTg levels, which likely represent a small residual thyroid mass, reflects the high RAI uptake of the residual thyroid, resulting in good therapeutic effects.
A putative explanation of the good prognostic value of star artifacts could be a different degree of differentiation of remnant thyroid tissue, mainly because of NIS expression. NIS is a well-known main carrier of iodide transport in thyrocytes [
6]. In a previous study, patients with positive NIS immunostaining responded to RAI treatment better than those with negative immunostaining, although the authors did not provide recurrence or mortality data [
11]. Therefore, we could hypothesize that when the remnant tumor size is similar, a higher uptake of RAI due to a higher expression of NIS could increase the efficacy of RAI treatment, and that might lead to a better prognosis. Additionally, it has been proposed that measuring NIS expression in postoperative tissue might predict the effectiveness of RAI treatment, and could be helpful to determine individualized doses of RAI [
11]. Unfortunately, we did not measure the expression of NIS in this study, and further study is required to prove the hypothesis.
On the other hand, there still exists a possibility that the star artifact might reflect the high burden of remnant thyroid tissue and lead to a poor prognosis. Schneider et al. [
5] reported that recurred patients had a 10-fold higher uptake-to-dose ratio of RAI in remnant thyroid compared with those who did not. They suggested that the high uptake of RAI in remnant thyroid could be a risk factor for a recurrent disease. However, the previous study did not measure sTg levels, which might provide information about the residual amount of thyroid tissue, unlike the present study, in which the analysis was stratified according to sTg levels. The subgroup of patients with higher sTg levels had a higher recurrence rate and no significant difference in recurrence rates between patients with and without star artifacts. Therefore, sTg levels could be a better marker for reflecting the burden of remnant thyroid cancer or poor prognosis than star artifacts in those with high sTg levels.
The current study is the first study regarding the clinical outcomes of star artifacts, which found in almost 40% of patients after the initial RAI treatment. There has only been a case report [
4] and a review that mentioned star artifacts [
3]. Because most hospitals perform measurements of dose-to-response ratio during WBS only in research settings [
5], it is more practical to base the assessment on the presence of star artifacts, rather than measuring dose-to-response ratio. In addition, we performed a stratified analysis according to the patients' sTg levels, allowing us to distinguish patients with a high burden of remnant thyroid cancer. Lastly, the duration of follow-up in this study was quite long (median, 8 years), which enables a comparison of long-term recurrence, but not mortality data.
The study also has a few limitations. As previously described, we could not evaluate NIS expression in postoperative thyroid tissue to confirm our hypothesis. Also, because the incidence of star artifacts could be variable by doses of RAI therapy and the acquisition methods such as camera and collimators, the incidence of star artifact in this study cannot be generalized in other settings. In addition, the presence of star artifacts was determined subjectively, and we were unable to assess the correlation between dose-to-response ratio and star artifacts in this retrospective study. Dose-to-response ratio can be measured during the WBS, but this is not a routine process in the hospital. However, the star artifact itself could be useful in clinical settings, because the measurement of dose-to-response ratio is rarely done in most hospitals.
In conclusion, in patients with low sTg, a star-shaped intense uptake on WBS, a star artifact, may reflect the positive therapeutic efficacy of initial RAI therapy in patients with DTC. It might be applicable as a prognostic factor in patients with sTg levels ≤2 ng/mL.
Go to :
