Abstract
Figures and Tables
Figure 1
Specific activity of antioxidant enzymes (A: SOD, B: GPX, C: CAT, and D: GST) in the jejunum of piglets fed the diets supplemented with 100 ppm of Zn with ZnO (ZnO-1), 2,500 ppm of Zn with ZnO (ZnO-2), 100 ppm of Zn with lipid-coated with ZnO (LCZ-1), 200 ppm of Zn with lipid-coated with ZnO (LCZ-2), and 400 ppm of Zn with lipid-coated with ZnO (LCZ-3). Means (Mean±SE, n=8) with different superscript differ among groups (P<0.05). Asterisk (*) indicates the significance difference in the linear and quadratic effects (P<0.05) in response to the dietary LCZ level.
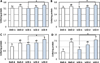
Figure 2
Specific activity of antioxidant enzymes (A: SOD, B: GPX, C: CAT, and D: GST) in the liver of piglets fed the diets supplemented with 100 ppm of Zn with ZnO (ZnO-1), 2,500 ppm of Zn with ZnO (ZnO-2), 100 ppm of Zn with lipid-coated with ZnO (LCZ-1), 200 ppm of Zn with lipid-coated with ZnO (LCZ-2), and 400 ppm of Zn with lipid-coated with ZnO (LCZ-3). Means (Mean±SE, n=8) with different superscript differ among groups (P<0.05). Asterisk (*) indicates the significance difference in the linear and quadratic effects (P<0.05) in response to the dietary LCZ level.
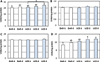
Figure 3
The levels of total antioxidant power in the plasma of piglets fed the diets supplemented with 100 ppm of Zn with ZnO (ZnO-1), 2,500 ppm of Zn with ZnO (ZnO-2), 100 ppm of Zn with lipid-coated with ZnO (LCZ-1), 200 ppm of Zn with lipid-coated with ZnO (LCZ-2), and 400 ppm of Zn with lipid-coated with ZnO (LCZ-3). Data represents Mean±SE for 8 piglets. Asterisk (*) indicates the significance difference in the linear and quadratic effects (P<0.05) in response to the dietary LCZ level.
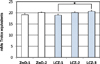
Figure 4
The levels of MDA (A) in microsomal fraction of the jejunum (A) and liver (B) in the liver of piglets fed the diets supplemented with 100 ppm of Zn with ZnO (ZnO-1), 2,500 ppm of Zn with ZnO (ZnO-2), 100 ppm of Zn with lipid-coated with ZnO (LCZ-1), 200 ppm of Zn with lipid-coated with ZnO (LCZ-2), and 400 ppm of Zn with lipid-coated with ZnO (LCZ-3). Data represents Mean±SE for 8 piglets.
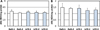
Table 1
The formulation and nutrient levels of the basal diets (as-fed basis)
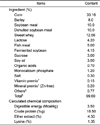
†Provided per kg of diet: 1,500 ppm IU vitamin A, 2,000 IU vitamin D3, 65 IU vitamin E, 1.5 mg vitamin K, 1.0 mg thiamin, 6 mg riboflavin, 20 mg pantothenic acid, 25 mg niacin, 1.5 mg vitamin B6, 1 mg folic acid, 25 µg vitamin B12, 25 µg biotin, and 150 mg choline. ‡Provided per kg: 160 mg Cu, 200 mg Fe, 40 mg Mn, 1 mg I, 0.15 mg Co, and 0.4 mg Se. §Provided per total weight: 0.1% choline-HCl, 0.347% L-lysine-HCl (78%), 0.150% DL-methionine (99%), 0.114% L-threonine (99%), 0.009% L-tryptophan, and 0.05% ethoxyquin. ¶Five experimental diets were supplemented to the basal diet with 125 mg ZnO, 3,125 mg ZnO, 139 mg of 10% lipid (w/w)-coated ZnO [CTCBIO, Seoul], 278 mg CZ, and 556 mg CZ per kg of diet, respectively, in addition to 0 to 0.3% corn, to provide 100, 2,500, 100, 200, and 400 mg Zn/kg, respectively.
Table 2
Primers used for the quantification of mRNA using Real Time-PCR
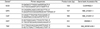
Table 3
Effect of Zinc source and level on the mRNA expression of antioxidant enzymes (SOD, GPX, CAT and GST) in the jejunal mucosal tissues of piglets
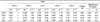
*ZnO-1 (ZnO, 100 ppm), ZnO-2 (ZnO 2,500 ppm), LCZ-1 (lipid-coated ZnO, 100 ppm), LCZ-2 (lipid-coated ZnO, 200 ppm), and LCZ-3 (lipid-coated ZnO, 400 ppm).
The values are ΔCT, which is represented as the CT of each target gene corrected by CT of the control gene (TBP).
The fold difference in the relative expression of the target gene was calculated as the 2−ΔΔCT.
Means (n=8) with different superscript differ among groups (P<0.05).
Table 4
Effect of Zinc source and level on the mRNA expression of antioxidant enzymes (SOD, GPX, CAT, and GST) in the liver of piglets
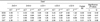
*ZnO-1 (ZnO, 100 ppm), ZnO-2 (ZnO 2,500 ppm), LCZ-1 (lipid-coated ZnO, 100 ppm), LCZ-2 (lipid-coated ZnO, 200 ppm), and LCZ-3 (lipid-coated ZnO, 400 ppm).
The values are ΔCT, which is represented as the CT of each target gene corrected by CT of the control gene (TBP).
The fold difference in the relative expression of the target gene was calculated as the 2−ΔΔCT.
Means (n=8) with different superscript differ among groups (P<0.05).
Acknowledgments
References

























