See the Original "Effects of DA-5513 on alcohol metabolism and alcoholic fatty liver in rats" on page 49.
See the retraction "Effects of DA-5513 on alcohol metabolism and alcoholic fatty liver in rats" in Volume 34 on page 49.
Abstract
Hangover is characterized by a number of unpleasant physical and mental symptoms that occur after heavy alcohol drinking. In addition, consistently excessive alcohol intake is considered as a major reason causes liver disease. The present study investigated the in vivo effects of DA-5513 (Morning care® Kang Hwang) on biological parameters relevant to hangover relief and alcoholic fatty liver. Blood alcohol and acetaldehyde concentrations were determined in rats administered a single dose of alcohol and treated with DA-5513 or commercially available hangover relief beverages (Yeomyung® and Ukon®). The effects of DA-5513 on alcoholic fatty liver were also determined in rats fed alcohol-containing Lieber-DeCarli diets for 4 weeks. Serum liver function markers (aspartate and alanine aminotransferase activities) and serum/liver lipid levels were assessed. Blood alcohol and acetaldehyde concentrations were lower in the groups treated with DA-5513 or Yeomyung®, as compared with control rats. However, Ukon® did not produce any significant effects on these parameters. Treatment with DA-5513 significantly reduced serum aspartate and alanine aminotransferase activities and markedly reduced serum cholesterol and triglyceride levels, as compared with control rats. Histological observations using Oil Red O staining found that DA-5513 delayed the development of alcoholic fatty liver by reversing hepatic fat accumulation. These findings suggest that DA-5513 could have a beneficial effect on alcohol-induced hangovers and has the potential to ameliorate alcoholic fatty liver.
Alcoholic liver disease has been demonstrated to be a major cause of morbidity and mortality worldwide in individuals with consistently excessive alcohol intake [1]. Hangover is characterized by a number of unpleasant physical and mental symptoms that occur after heavy alcohol drinking [23]. Alcohol is initially oxidized to acetaldehyde by the alcohol dehydrogenase (ADH) enzyme; this is subsequently converted to acetate by aldehyde dehydrogenase (ALDH) in the liver [23]. Acetaldehyde is much more toxic than ethanol and this metabolite may cause the physical symptoms of hangover such as fatigue, headache, increased sensitivity to light and sound, redness of the eyes, muscle aches, and thirst [3]. Furthermore, long-term consumption of alcohol in large quantities may cause chronic liver diseases and hepatic steatosis (alcoholic fatty liver), which is defined as excess lipid accumulation in the cytoplasm of hepatocytes; this is regarded as a significant risk factor for hepatic fibrosis and cirrhosis [4]. Thus, reduction of alcohol-induced hepatic fat accumulation may block or delay the progression of steatosis to advanced stages of alcoholic liver disease.
Multiple mechanisms contribute to the pathogenesis of alcoholic hepatic steatosis, including increased de novo hepatic lipogenesis, impaired mitochondrial fatty acid β-oxidation, and reduced export of very low-density lipoprotein [5]. However, the complex mechanisms involved in alcoholic fatty liver formation have been debated in the literature [6]. Accumulating evidence has suggested that adipose tissue dysfunction might impact on hepatic lipid metabolism [78]. A direct link between adipose triglyceride (TG) loss and hepatic TG gain was revealed using deuterium-labeled TG in alcohol-fed mice [9]. Dysregulation of lipid homeostasis was also evidenced in clinical studies, which identified a lower fat mass during the development of alcoholic fatty liver [10]. Adipose tissue plays a crucial role as a major metabolic buffering system in lipid homeostasis [1112], and modification of alcohol-induced dysfunction in this tissue might therefore provide an important target for the development of functional foods to prevent alcoholic fatty liver [13]. Many treatments have been reported to prevent and/or reduce the severity of hangover and alcoholic fatty liver symptoms, including innumerable folk remedies and recommendations.
Yeomyung® (YM, Glami, Gwangwon, Korea) is the most commonly sold hangover recovery drink in Korea, and Ukon® (UK, Enagic, Okinawa, Japan) is a turmeric extract-based beverage that is marketed as an anti-hangover drink and has enjoyed huge success in Japan. In addition, various products have been released on the basis that they decrease the accumulation of fat in the liver, prevent liver damage, and alleviate alcohol-related hangovers. However, the mechanism of action of these products has not been revealed, and scientific research into this area is therefore required. DA-5513 (Morning care® Kang Hwang) was developed by the Dong-A Pharm. Co. (Yongin, Korea) and has been approved by the Korea Food and Drug Administration as an over-the-counter treatment for hangover. The proprietary liquid DA-5513 formulation was developed using seven herbal extracts, including GMT-ALC-5L (fermented rice embryo and bean extract), Curcuma longa L., Trapa japonica Flerov., Silybum marianum L., Paullinia cupana Mart., Pueraria thunbergiana Benth., and honey. These natural products have been used in traditional oriental medicines to prevent alcohol-induced hangover and protect the liver against diverse hepatotoxins such as ethanol, carbon tetrachloride, antitubercular agents, and thioacetamide [14151617181920].
The present study was therefore designed to investigate the effect of DA-5513 on hangover relief, which would be associated with the rapid elimination of alcohol and acetaldehyde. Furthermore, a chronic ethanol-treated rat model was developed to evaluate the ability of this product to protect from alcoholic fatty liver.
DA-5513 was provided by the Dong-A Pharm. Co. (Yongin, Korea), while Yeomyung® (YM, Glami, Gwangwon, Korea) and Ukon® (UK, Enagic, Okinawa, Japan) were purchased from the market. All other chemicals used were of analytical grade.
Seven-week-old male Wistar rats were purchased from Chung-Ang Lab Animal Inc. (Seoul, Korea) and acclimatized to the laboratory setting (22.0±2.0℃, 12-h light/dark cycles, 55%±5% humidity) with free access to water and food (Samyang Co, Incheon, Korea) for a week before the experiment. The experimental protocol was approved by the Institutional Animal Care and Use Committee of Dong-A Pharm. Co. (Yongin, Korea), and all experimental procedures were conducted in compliance with this company's guidelines for the care and use of laboratory animals.
The rats (200–250 g) were randomly divided into four groups (n=8 per group). Each group had a single oral administration of the following test treatments: ethanol (control group), and ethanol with either DA-5513, YM, or UK. Then, after 0.5 h, 10 mL/kg of body weight was administered. At 0.5, 1, 2, 4, and 6 h post-ethanol administration, 0.2 mL blood was collected from each rat and left at room temperature for 30 min before centrifugation at 3,000 rpm for 15 min. The levels of alcohol and acetaldehyde in the supernatant were measured using kits for the detection of ethanol (Roche Co., Darmstadt, Germany) and acetaldehyde (R-Biopharm, Darmstadt, Germany). During the metabolism of ethanol to acetaldehyde and acetate, nicotinamide adenine dinucleotide (NAD+) is converted to NADH. Thus, the concentration of NADH was determined by measuring absorbance at 340 nm.
Rats (220–230 g) were randomly divided into four groups based on their body weight (n=10 per group): untreated control (CON), ethanol-treated control (ED), and ethanol-treated with UK or DA-5513 groups. The rats were fed a standard Lieber-DeCarli ethanol diet (36% ethanol-derived calories) for 4 weeks [2122]; pair-fed control rats were administered dextran-maltose to match the alcohol-derived calories in the ethanol diet. DA-5513 or UK was introduced into the alcohol diet by gradually mixing it with distilled water and feeding it at the same time each day throughout the experiment. The rats were sacrificed using ether anesthesia; blood samples were centrifuged (1,500 g, 4℃, 10 min) to separate the serum and stored at −80℃ until analysis. The livers were quickly removed and preserved in phosphate-buffered formalin for histological examination. The rest of the liver was frozen at −80℃ prior to analysis of hepatic TG levels.
Serum levels of alanine aminotransferase (ALT), aspartate aminotransferase (AST), total cholesterol (T-CHO), and TG were monitored by standard clinical chemistry assays on an Automated Chemistry Analyzer (Prestige 24I; Tokyo Boeki Medical System, Tokyo, Japan). Total liver lipids were extracted from homogenates prepared from 100mg rat liver using chloroform:methanol (2:1, v/v) [23]. The TG levels within total lipid samples were determined enzymatically using a commercially available enzymatic kit (Sigma Chem. Co.) according to the manufacturer's protocol.
As proposed by Levene et al., Oil Red O can be used to identify lipids and quantify hepatic steatosis. Several protocols have been developed, including paraffin-embedded sections and cryosections (or frozen sections) [2425]. In this study, frozen liver tissues were cut into 4-mm sections and affixed to microscope slides. Sections were stained with Oil Red O solution buffer. Histopathologic examinations of the liver sections were conducted by a pathologist and were peer-reviewed. The slides were dehydrated, dealcoholized, mounted utilizing Canada balsam, and assessed for inflammation and tissue damage utilizing an Olympus microscope (Olympus, Tokyo, Japan) [2627].
In order to determine the effects of DA-5513 on hangovers, blood alcohol levels were investigated and presented in Table 1. DA-5513, YM, or UK was administered orally 0.5 h before 25% ethanol consumption, and blood was collected 0.5, 1, 2, 4, and 6 h after alcohol was administered. Administration of UK resulted in a lower blood alcohol concentration than that observed in the control group; however, this difference was not statistically significant (P>0.05). On the other hand, DA-5513 and YM produced a similar significant reduction of the blood alcohol concentration at each of the timepoints examined, as compared with the control group (Table 1).
The blood acetaldehyde concentration, which was highest in the CON group, peaked 0.5 h after ethanol consumption (Table 2). Rats treated with UK showed lower blood acetaldehyde levels than control rats, but these differences were not statistically significant. Both DA-5513 and YM significantly reduced the acetaldehyde levels in the blood at every time-point examined, as compared with the control group. However, this effect was more marked for DA-5513 than for YM throughout the study. Taken together, these findings indicated that DA-5512 provided more effective reduction of alcohol and acetaldehyde levels than other products, suggesting that it has the potential to act as a hangover relief beverage.
All rats were fed the standard Lieber-DeCarli ethanol diet for 4 weeks and hepatotoxicity was evaluated by clinical chemistry. As seen in Figure 1A and 1B, the ED group showed a marked increase in the levels of serum AST and ALT (by approximately 1.3- and 1.4-fold, respectively), as compared to the untreated control group (P<0.01). The administration of UK product after ethanol diet could reduce the ALT level (P<0.05) but not AST level in comparison with ED group. In contrast, the blood samples of the animals treated with DA-5513 revealed significant hepatoprotective activity, as evidenced by an amelioration of this increase in serum AST and ALT levels (P<0.01 and P<0.05, respectively).
The serum T-CHO and TG levels (Figure 2A and 2B) increased significantly in the ED group (by approximately 1.2- and 1.8-fold, respectively) (P<0.01). Animals that received DA-5513 showed a significantly lower level of both serum TG and T-CHO than that of ED group (P<0.01 and P<0.05, respectively) while the difference between UK and ED group was not significant.
In addition, qualitative hepatic TG measurement, which further confirmed the histological results, demonstrated that alcohol feeding with Lieber-DeCarly diet greatly increased the hepatic TG level in mice by 1.8-fold, in comparison with control group (Figure 3). This elevation was significant decreased by concomitant administration of UK product (P<0.05) or DA-5513 (P<0.01).
The Oil Red O staining technique was employed to examine lipid droplet accumulation and histological changes in the liver. As shown in Figure 4, a massive accumulation of lipid droplets was found in the ED group. Normal cells exhibited lobular architectures, but cells in the ED group exhibited panlobular mixed micro/macro vesicular steatosis and focal clusters of inflammatory cells, with associated necrosis. In contrast, these pathologic changes were markedly attenuated in the DA-5513 group, which was consistent with results of serum and hepatic TG measurement.
Heavy alcohol drinking can result in several alcohol-induced hangover symptoms, which are attributed to the physiological effects of alcohol and its metabolites. It is well established that accumulation of acetaldehyde, an intermediate alcohol metabolite, plays a pivotal role in the development of hangover [23]. In order to determine the effects of DA-5513 on hangovers, we measured rat blood alcohol and acetaldehyde levels at different timepoints after the administration of alcohol. Two commercially available products, YM and UK, were used as positive control treatments. The administration of DA-5513 and YM was associated with lower blood alcohol and acetaldehyde levels over the time-course of the experiment. However, the reduction in the acetaldehyde level observed in rats treated with DA-5513 was 20% and 27% greater than that observed in rats treated with YM and UK, respectively. These findings indicated that DA-5512 produced more effective reduction of the acetaldehyde level than YM, demonstrating that it had the potential to act as a hangover relief beverage.
The liver is the largest internal organ in the human body and it has many different roles. One of its most important functions is to filter harmful substances from the blood. The liver commonly repairs itself by rebuilding new liver cells when the old ones are damaged. However, chronic alcohol ingestion can lead to the development of liver diseases such as fatty liver, alcoholic hepatitis, and cirrhosis [4]. This liver damage occurs through several interrelated pathways. The oxidative reactions involved in alcohol metabolism generate hydrogen, which converts NAD to NADH, increasing the redox potential (NADH/NAD) of the liver [28]. This increase in redox potential inhibits fatty acid oxidation and gluconeogenesis, promoting fat accumulation in the liver. In addition, chronic alcoholism induces the microsomal ethanol-oxidizing system to break down alcohol, mainly in the endoplasmic reticulum [29]. This pathway, where cytochrome P450 2E1 is the main enzyme, can account for 20% of alcohol metabolism. This enzyme is upregulated by chronic alcohol use, and generates free radicals and harmful reactive oxygen species via the oxidation of nicotinamide adenine dinucleotide phosphate (NADPH) to NADP [28]. This oxidative stress promotes hepatocyte necrosis and apoptosis, and lipid peroxidation, which causes inflammation and fibrosis. Inflammation is also exacerbated by acetaldehyde, which can bind covalently to cellular proteins, forming antigenic adducts [3031].
Apart from the inconvenient symptoms of hangover, long-term consumption of alcohol in large quantities is the leading cause of liver disease and hepatic steatosis. Alcoholic fatty liver disease results from the deposition of fat and the accumulation of TG in liver cells. The potential pathophysiologic mechanisms involved in fatty liver include a reduction in mitochondrial fatty acid β-oxidation, increased endogenous fatty acid synthesis or enhanced delivery of fatty acids to the liver, and deficient incorporation or export of TG as very low-density lipoproteins [3233]. The Lieber-DeCarli liquid diet model is used to induce alcoholic fatty liver disease in animals, where it causes liver injury, steatosis, and oxidative stress. This model has been used to investigate the relationship between alcohol and therapeutic agents [2234]. Previous data have indicated that feeding mice with a standard Lieber-DeCarli formula for 2 weeks was sufficient to induce significant steatosis [22]. The degree of steatosis, determined by the hepatic TG concentration, revealed that the liver samples met the criteria for a clinical diagnosis of steatosis [35]. In our study, mice treated with the Lieber-DeCarli diet for 4 weeks showed steatosis, as confirmed by a significant increase in serum ALT and AST activities, T-CHO, and TG levels. Our data showed that dietary DA-5513 markedly attenuated the hepatic steatosis observed in this model, as indicated by Oil Red O staining, hepatic TG quantification, and serum measures of AST, ALT, T-CHO, and TG.
Mono-and poly-herbal preparations have been used in traditional medical systems for the treatment of liver disease since long before recorded history; some of these products appear to have positive effects on this potentially reversible disease. Both basic and clinical studies have suggested that herbal medicines and their constituents such as Gynostemma pentaphyllum (Thunb.) Makino, Panax notoginseng (Burkill) F.H.Chen (saponins), Crataegus pinnatifida Bunge (penta-oligogalacturonide), Dioscorea opposita Thunb. (dioscin), Punica granatum L. (gallic acid), glycyrrhizin, silymarin, Prunus armeniaca L (kernels), and baicalin may have modest benefits in the treatment of fatty liver disease [36]. Therefore, DA-5513, which is composed of several herbal extracts, was investigated as a hangover remedy that may reduce the blood alcohol concentration, as well as preventing alcoholic fatty liver. GMT-ALC-5L (fermented rice embryo and bean extract) promotes the health of both habitual alcohol drinkers and non-habitual alcohol drinkers. This preparation has been demonstrated to reduce blood alcohol levels and promote liver function recovery by modulating alcohol-metabolizing enzymes [3738]. Turmeric (Curcuma longa) has been used in traditional medicines as a household remedy for various diseases, including biliary disorders, anorexia, cough, diabetic wounds, rheumatism, sinusitis, and hepatic disorders. In vitro and in vivo animal studies have provided evidence for the hepatoprotective effects of turmeric against a variety of hepatotoxic substances, including carbon tetrachloride, galactosamine, pentobarbitol, 1-chloro-2,4-dinitrobenzene, 4-hydroxynonenal, and acetaminophen (paracetamol) [39]. These hepatoprotective effects may stem from the potent antioxidant effects of turmeric. Dietary supplementation of turmeric in rats (1% turmeric by weight for 10 weeks) was found to significantly protect against iron-induced lipid peroxide formation [40]. Curcumin is also helpful in the relief of hangover. It exhibited an inhibitory effect on alcohol intoxication in humans, as evidenced by a reduced blood acetaldehyde concentration and reduced discomfort [4142]. Curcumin significantly reduces plasma low-density and very low-density lipoprotein levels, reduces T-CHO levels in the liver, and increases the α-tocopherol level in rat plasma, suggesting an in vivo interaction between curcumin and α-tocopherol that may increase the bioavailability of vitamin E and decrease T-CHO levels [43]. Silybum marianum (milk thistle) has been used for centuries as an herbal medicine for the treatment of liver disease. Silymarin's hepatoprotective effects involve several mechanisms including an antioxidant effect, inhibition of lipid peroxidation, enhancement of liver detoxification via inhibition of phase I detoxification, and an increased hepatocyte protein synthesis, thereby promoting hepatic tissue regeneration [16]. Animal studies have also demonstrated that silybin could reduce the conversion of hepatic stellate cells into myofibroblasts, thus slowing or even reversing fibrosis [16]. Clinical studies of patients with chronic alcoholic liver disease in Austria and Hungary demonstrated that silymarin administration resulted in a normalization of serum liver enzyme and total bilirubin levels in patients with alcoholic liver disease, in addition to improved liver tissue histology [44]. In patients with cirrhosis, long-term (41 months) administration of silymarin at 420 mg per day resulted in a significant increase in survival, as compared to the placebo group [45]. Although most studies of water chestnuts (Trapa japonica Flerov.) have focused on their nutritional and ecological value, they have also been reported to have antioxidant, anti-cancer, and anti-diabetic effects; these were associated with a reduction in blood glucose level, and inhibition of α-amylase and α-glucosidase [15]. Trapa japonica is also used as an ethno-medicine for the treatment of gastric ulcer, diarrhea, alcohol hangover, and dysentery [46]. Trapa japonica Flerov. was reported to significantly inhibit the production of reactive oxygen species, thus protecting the liver from tert-butyl hydroperoxide (t-BHP)-induced damage by stabilizing antioxidant systems and regulating the mitochondrial membranes within liver cells [47]. In vivo models have indicated that Trapa japonica Flerov. significantly attenuated t-BHP-induced increases in serum glutamate oxaloacetate transaminase and glutamate pyruvate transaminase levels, and in hepatic malondialdehyde levels [48]. Guarana has also been used as a tonic for hangovers, neuralgia and menstrual headaches, leucorrhea, diarrhea, and fevers; this compound has been shown to prevent DNA damage in carbon tetrachloride-treated rats [1820]. Kudzu (Pueraria thunbergiana) is employed in traditional Chinese medicine and its major isoflavone constituent, puerarin, has antioxidant activity and a variety of biological actions in cardiovascular disease, gynecological disease, osteoporosis, cognition, and diabetic nephropathy [49]. Studies of Pueraria flos showed that it increased the acetaldehyde removal rate in both rats and humans after alcohol consumption, and reduced hangover symptoms [50]. The kudzu vine is potentially highly beneficial in the treatment of liver damage, as it scavenges reactive free radicals and boosts the endogenous antioxidant system. Kudzu vine extract significantly reduced the cytotoxicity and production of reactive oxygen species induced by t-BHP in vitro and lowered the plasma levels of ALT and AST in a rat model of carbon tetrachloride-induced hepatotoxicity [15]. Another ingredient that may help to counteract the effects of heavy alcohol drinking is honey. Honey contains fructose, a sugar that promotes alcohol metabolism [51]. Furthermore, honey has considerable anti-inflammatory, antioxidant, and antitumor activities, and plays a key role in normalizing kidney function and protecting the liver from a range of toxic agents [52]. Consistent with these findings, the combination of these herbal ingredients in DA-5513 significantly ameliorated hepatic steatosis, as evidenced by its effects on hepatic TG, serum ALT and AST activities, and serum T-CHO and TG levels.
In summary, these findings indicated that DA-5513 produced beneficial effects on alcohol metabolite levels and alcoholic fatty liver in rats. Further studies are required to investigate the antioxidant activity of this preparation, and its effects on lipid mechanism, in rats administered alcohol.
References
1. Rehm J, Mathers C, Popova S, Thavorncharoensap M, Teerawattananon Y, Patra J. Global burden of disease and injury and economic cost attributable to alcohol use and alcohol-use disorders. Lancet. 2009; 373(9682):2223–2233. PMID: 19560604.


2. Wiese J, McPherson S, Odden MC, Shlipak MG. Effect of Opuntia ficus indica on symptoms of the alcohol hangover. Arch Intern Med. 2004; 164(12):1334–1340. PMID: 15226168.


3. Swift R, Davidson D. Alcohol hangover: mechanisms and mediators. Alcohol Health Res World. 1998; 22(1):54–60. PMID: 15706734.
4. Lieber CS. Alcoholic fatty liver: its pathogenesis and mechanism of progression to inflammation and fibrosis. Alcohol. 2004; 34(1):9–19. PMID: 15670660.


5. Altamirano J, Bataller R. Alcoholic liver disease: pathogenesis and new targets for therapy. Nat Rev Gastroenterol Hepatol. 2011; 8(9):491–501. PMID: 21826088.


6. Purohit V, Gao B, Song BJ. Molecular mechanisms of alcoholic fatty liver. Alcohol Clin Exp Res. 2009; 33(2):191–205. PMID: 19032584.


7. Lafontan M, Girard J. Impact of visceral adipose tissue on liver metabolism: Part I: Heterogeneity of adipose tissue and functional properties of visceral adipose tissue. Diabetes Metab. 2008; 34(4 Pt 1):317–327. PMID: 18550411.
8. Wree A, Kahraman A, Gerken G, Canbay A. Obesity affects the liver-the link between adipocytes and hepatocytes. Digestion. 2011; 83(1-2):124–133. PMID: 21042023.
9. Zhong W, Zhao Y, Tang Y, Wei X, Shi X, Sun W, Sun X, Yin X, Sun X, Kim S, McClain CJ, Zhang X, Zhou Z. Chronic alcohol exposure stimulates adipose tissue lipolysis in mice: role of reverse triglyceride transport in the pathogenesis of alcoholic steatosis. Am J Pathol. 2012; 180(3):998–1007. PMID: 22234172.
10. Addolorato G, Capristo E, Greco AV, Stefanini GF, Gasbarrini G. Influence of chronic alcohol abuse on body weight and energy metabolism: is excess ethanol consumption a risk factor for obesity or malnutrition? J Intern Med. 1998; 244(5):387–395. PMID: 9845854.


11. Li Y, Wong K, Giles A, Jiang J, Lee JW, Adams AC, Kharitonenkov A, Yang Q, Gao B, Guarente L, Zang M. Hepatic SIRT1 attenuates hepatic steatosis and controls energy balance in mice by inducing fibroblast growth factor 21. Gastroenterology. 2014; 146(2):539–549.e7. PMID: 24184811.


12. Suter PM, Schutz Y, Jequier E. The effect of ethanol on fat storage in healthy subjects. N Engl J Med. 1992; 326(15):983–987. PMID: 1545851.


13. Neuschwander-Tetri BA. Hepatic lipotoxicity and the pathogenesis of nonalcoholic steatohepatitis: the central role of nontriglyceride fatty acid metabolites. Hepatology. 2010; 52(2):774–788. PMID: 20683968.


14. Pulido-Moran M, Moreno-Fernandez J, Ramirez-Tortosa C, Ramirez-Tortosa M. Curcumin and health. Molecules. 2016; 21(3):264. PMID: 26927041.


15. You Y, Duan X, Wei X, Su X, Zhao M, Sun J, Ruenroengklin N, Jiang Y. Identification of major phenolic compounds of Chinese water chestnut and their antioxidant activity. Molecules. 2007; 12(4):842–852. PMID: 17851436.
16. Vargas-Mendoza N, Madrigal-Santillán E, Morales-González Á, Esquivel-Soto J, Esquivel-Chirino C, García-Luna y González-Rubio M, Gayosso-de-Lucio JA, Morales-González JA. Hepatoprotective effect of silymarin. World J Hepatol. 2014; 6(3):144–149. PMID: 24672644.
17. Chang BY, Lee DS, Lee JK, Kim YC, Cho HK, Kim SY. Protective activity of kudzu (Pueraria thunbergiana) vine on chemically-induced hepatotoxicity: in vitro and in vivo studies. BMC Complement Altern Med. 2016; 16:39. PMID: 26825303.


18. Mattei R, Dias RF, Espínola EB, Carlini EA, Barros SB. Guarana (Paullinia cupana): toxic behavioral effects in laboratory animals and antioxidants activity in vitro. J Ethnopharmacol. 1998; 60(2):111–116. PMID: 9582000.
19. Cheng N, Du B, Wang Y, Gao H, Cao W, Zheng J, Feng F. Antioxidant properties of jujube honey and its protective effects against chronic alcohol-induced liver damage in mice. Food Funct. 2014; 5(5):900–908. PMID: 24603671.


20. Kober H, Tatsch E, Torbitz VD, Cargnin LP, Sangoi MB, Bochi GV, da Silva AR, Barbisan F, Ribeiro EE, da Cruz IB, Moresco RN. Genoprotective and hepatoprotective effects of Guarana (Paullinia cupana Mart. var. sorbilis) on CCl4-induced liver damage in rats. Drug Chem Toxicol. 2016; 39(1):48–52. PMID: 25791997.
21. Kim CI, Leo MA, Lowe N, Lieber CS. Differential effects of retinoids and chronic ethanol consumption on membranes in rats. J Nutr. 1988; 118(9):1097–1103. PMID: 3418417.


22. Yin HQ, Lee BH. Temporal changes in the hepatic fatty liver in mice receiving standard Lieber-DeCarli diet. Toxicol Res. 2008; 24(2):113–117.


23. Bligh EG, Dyer WJ. A rapid method of total lipid extraction and purification. Can J Biochem Physiol. 1959; 37(8):911–917. PMID: 13671378.


24. Levene AP, Kudo H, Thursz MR, Anstee QM, Goldin RD. Is oil red-O staining and digital image analysis the gold standard for quantifying steatosis in the liver? Hepatology. 2010; 51(5):1859. PMID: 20432267.


25. Kucherenko MM, Marrone AK, Rishko VM, Yatsenko AS, Klepzig A, Shcherbata HR. Paraffin-embedded and frozen sections of drosophila adult muscles. J Vis Exp. 2010; (46):2438. PMID: 21206479.
26. Fakhoury-Sayegh N, Trak-Smayra V, Khazzaka A, Esseily F, Obeid O, Lahoud-Zouein M, Younes H. Characteristics of nonalcoholic fatty liver disease induced in wistar rats following four different diets. Nutr Res Pract. 2015; 9(4):350–357. PMID: 26244072.


27. Yogalakshmi B, Sreeja S, Geetha R, Radika MK, Anuradha CV. Grape Seed Proanthocyanidin Rescues Rats from Steatosis: A Comparative and Combination Study with Metformin. J Lipids. 2013; 2013:153897. PMID: 24307947.


28. Lieber CS. Alcohol: its metabolism and interaction with nutrients. Annu Rev Nutr. 2000; 20(1):395–430. PMID: 10940340.


29. Lu Y, Cederbaum AI. CYP2E1 and oxidative liver injury by alcohol. Free Radic Biol Med. 2008; 44(5):723–738. PMID: 18078827.


30. Guengerich FP, Beaune PH, Umbenhauer DR, Churchill PF, Bork RW, Dannan GA, Knodell RG, Lloyd RS, Martin MV. Cytochrome P-450 enzymes involved in genetic polymorphism of drug oxidation in humans. Biochem Soc Trans. 1987; 15(4):576–578. PMID: 3678578.


31. Stewart S, Jones D, Day CP. Alcoholic liver disease: new insights into mechanisms and preventative strategies. Trends Mol Med. 2001; 7(9):408–413. PMID: 11530336.


32. Ceni E, Mello T, Galli A. Pathogenesis of alcoholic liver disease: role of oxidative metabolism. World J Gastroenterol. 2014; 20(47):17756–17772. PMID: 25548474.


33. Lakshman MR. Some novel insights into the pathogenesis of alcoholic steatosis. Alcohol. 2004; 34(1):45–48. PMID: 15670665.


34. Lieber CS, DeCarli LM, Sorrell MF. Experimental methods of ethanol administration. Hepatology. 1989; 10(4):501–510. PMID: 2673971.


35. Adams LA, Lymp JF, St Sauver J, Sanderson SO, Lindor KD, Feldstein A, Angulo P. The natural history of nonalcoholic fatty liver disease: a population-based cohort study. Gastroenterology. 2005; 129(1):113–121. PMID: 16012941.


36. Hong M, Li S, Tan HY, Wang N, Tsao SW, Feng Y. Current status of herbal medicines in chronic liver disease therapy: the biological effects, molecular targets and future prospects. Int J Mol Sci. 2015; 16(12):28705–28745. PMID: 26633388.


37. Lee HS, Song J, Kim TM, Joo SS, Park D, Jeon JH, Shin S, Park HK, Lee WK, Ly SY, Kim MR, Lee DI, Kim YB. Effects of a preparation of combined glutathione-enriched yeast and rice embryo/soybean extracts on ethanol hangover. J Med Food. 2009; 12(6):1359–1367. PMID: 20041794.


38. Takahashi H, Greenway H, Matsumura H, Tsutsumi N, Nakazono M. Rice alcohol dehydrogenase 1 promotes survival and has a major impact on carbohydrate metabolism in the embryo and endosperm when seeds are germinated in partially oxygenated water. Ann Bot. 2014; 113(5):851–859. PMID: 24431339.


39. Kiso Y, Suzuki Y, Watanabe N, Oshima Y, Hikino H. Antihepatotoxic principles of Curcuma longa rhizomes. Planta Med. 1983; 49(3):185–187. PMID: 6657788.


40. Reddy AC, Lokesh BR. Effect of dietary turmeric (Curcuma longa) on iron-induced lipid peroxidation in the rat liver. Food Chem Toxicol. 1994; 32(3):279–283. PMID: 8157223.
41. Sasaki H, Sunagawa Y, Takahashi K, Imaizumi A, Fukuda H, Hashimoto T, Wada H, Katanasaka Y, Kakeya H, Fujita M, Hasegawa K, Morimoto T. Innovative preparation of curcumin for improved oral bioavailability. Biol Pharm Bull. 2011; 34(5):660–665. PMID: 21532153.


42. Hamano T, Nishi M, Itoh T, Ebihara S, Watanabe Y. The effect of beverage containing curcuma longa L. extract on the alcohol metabolism of healthy volunteers. Oyo Yakuri (Pharmacometrics). 2007; 72(1-2):31–38.
43. Kamal-Eldin A, Frank J, Razdan A, Tengblad S, Basu S, Vessby B. Effects of dietary phenolic compounds on tocopherol, cholesterol, and fatty acids in rats. Lipids. 2000; 35(4):427–435. PMID: 10858028.


44. Fehér J, Deák G, Müzes G, Láng I, Niederland V, Nékám K, Kárteszi M. Liver-protective action of silymarin therapy in chronic alcoholic liver diseases. Orv Hetil. 1989; 130(51):2723–2727. PMID: 2574842.
45. Ferenci P, Dragosics B, Dittrich H, Frank H, Benda L, Lochs H, Meryn S, Base W, Schneider B. Randomized controlled trial of silymarin treatment in patients with cirrhosis of the liver. J Hepatol. 1989; 9(1):105–113. PMID: 2671116.


46. Kim YS, Hwang JW, Jang JH, Son S, Seo IB, Jeong JH, Kim EH, Moon SH, Jeon BT, Park PJ. Trapa japonica pericarp extract reduces LPS-induced inflammation in macrophages and acute lung injury in mice. Molecules. 2016; 21(3):392. PMID: 27007369.


47. Kim YS, Hwang JW, Han YK, Kwon HJ, Hong H, Kim EH, Moon SH, Jeon BT, Park PJ. Antioxidant activity and protective effects of Trapa japonica pericarp extracts against tert-butylhydroperoxide-induced oxidative damage in Chang cells. Food Chem Toxicol. 2014; 64:49–56. PMID: 24269339.


48. Kim YS, Kim EK, Hwang JW, Seo IB, Jang JH, Son S, Jeong JH, Moon SH, Jeon BT, Park PJ. Characterization of the antioxidant fraction of Trapa japonica pericarp and its hepatic protective effects in vitro and in vivo. Food Funct. 2016; 7(3):1689–1699. PMID: 26956465.


49. Chen G, Li L. Nutrient consumption and production of isoflavones in bioreactor cultures of Pueraria Iobata (Willd). J Environ Biol. 2007; 28(2):321–326. PMID: 17915773.
50. Yamazaki T, Hosono T, Matsushita Y, Kawashima K, Someya M, Nakajima Y, Narui K, Hibi Y, Ishizaki M, Kinjo J, Nohara T. Pharmacological studies on Puerariae Flos. IV: Effects of Pueraria thomsonii dried flower extracts on blood ethanol and acetaldehyde levels in humans. Int J Clin Pharmacol Res. 2002; 22(1):23–28. PMID: 12395916.
51. Shi P, Chen B, Chen C, Xu J, Shen Z, Miao X, Yao H. Honey reduces blood alcohol concentration but not affects the level of serum MDA and GSH-Px activity in intoxicated male mice models. BMC Complement Altern Med. 2015; 15:225. PMID: 26169497.


52. Lowenstein LM, Simone R, Boulter P, Nathan P. Effect of fructose on alcohol concentrations in the blood in man. JAMA. 1970; 213(11):1899–1901. PMID: 4318655.


Figure 1
Effects of DA-5513 on serum (A) AST activity and (B) ALT activity in chronic ethanol-treated rats. CON, control diet; ED, alcohol diet; ED+UK, alcohol diet with UK; ED+DA-5513, alcohol diet with DA-5513. The data represent mean±SD (n=10); **P<0.01 and *P<0.05.
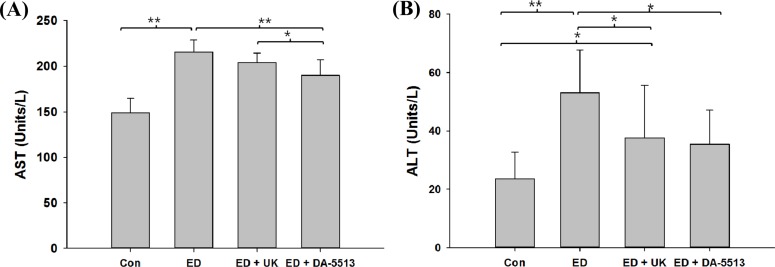
Figure 2
Effects of DA-5513 on serum (A) TG and (B) T-CHO levels in chronic ethanol-treated rats. CON, control diet; ED, alcohol diet; ED+UK, alcohol diet with UK; ED+DA-5513, alcohol diet with DA-5513. The data represent mean±SD (n=10); **P<0.01 and *P<0.05.
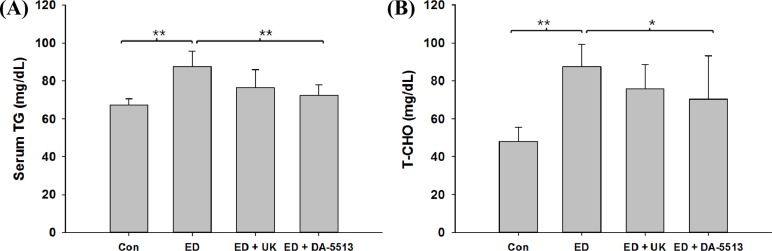
Figure 3
Effects of DA-5513 on hepatic TG in chronic ethanoltreated rats. CON, control diet; ED, alcohol diet; ED+UK; alcohol diet with UK; ED+DA-5513; alcohol diet with DA-5513. The data represent mean±SD (n=10); **P<0.01 and *P<0.05.
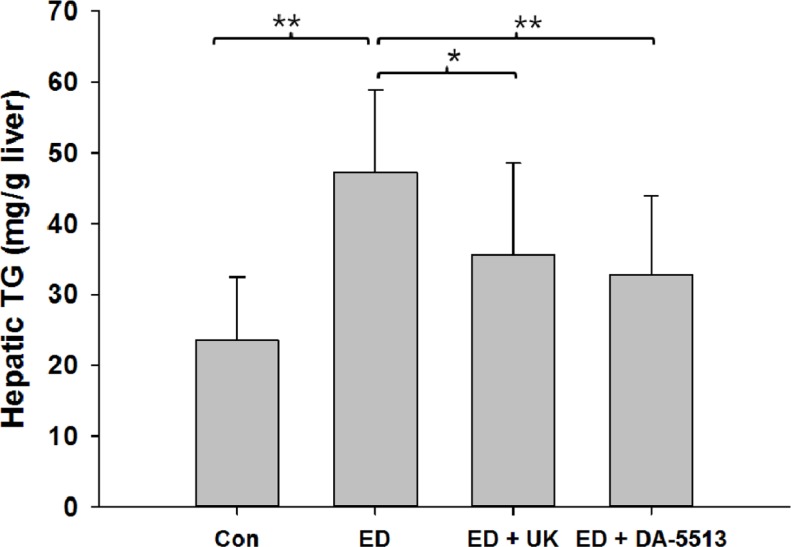
Figure 4
Effects of DA-5513 on hepatic lipid levels in chronic ethanol-treated rats. Liver sections were stained with Oil Red O for histopathological examination. CON, control diet; ED, alcohol diet; ED+UK, alcohol diet with UK; ED+DA-5513, alcohol diet with DA-5513.
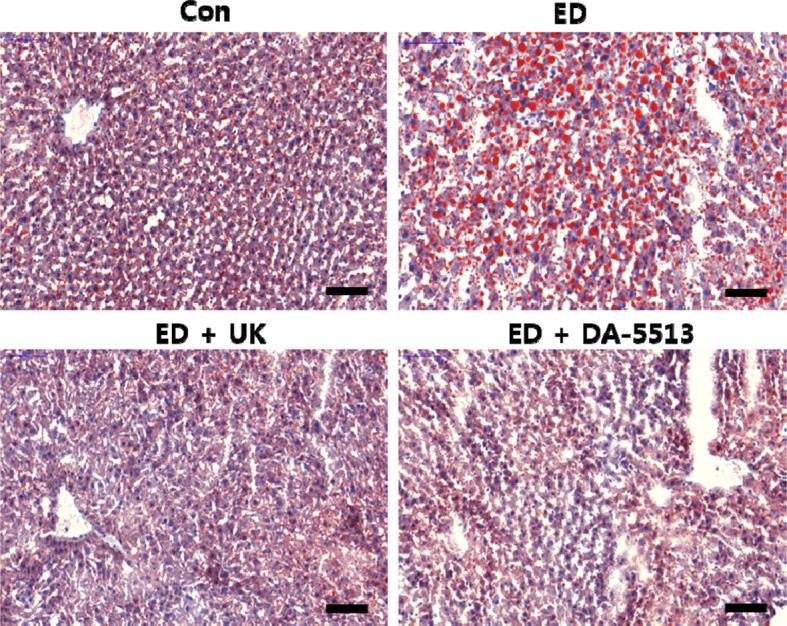
Table 1
Blood alcohol concentrations (mg/mL) in rats

Table 2
Blood acetaldehyde concentrations (µg/mL) in rats
