Pancreatic cancer was present in 3 patients, common bile duct neoplasm in 4 patients, small hepatic hemangiomas in 19 patients, small liver cysts in 5 patients, and liver metastases in 4 patients. The optimal monochromatic energies for imaging of the common hepatic artery, portal vein and hepatic vein in the 35 patients were located between 49 keV and 53 keV with an average of 51 keV. A significant (
p < 0.05) difference existed in the hepatic vascular CT value, IN, CNR, SNR, and subjective score among the optimal monochromatic, 70 keV and QC images. The CT value, IN, CNR, and SNR of the common hepatic artery, portal vein and hepatic vein were significantly (
p < 0.001) higher in the optimal monochromatic images (546 ± 97, 38.0 ± 18.2, 24.6 ± 10.9, and 28.3 ± 12.3,
p < 0.001, respectively, for the common hepatic artery; 293 ± 45, 27.4 ± 11.0, 6.9 ± 2.7, and 17.6 ± 5.7,
p < 0.001, respectively, for the portal vein; and 281 ± 49, 24.7 ± 9.2, 5.7 ± 2.3, and 14.9 ± 4.9,
p < 0.001, respectively for the hepatic vein) than in the 70 keV (287 ± 49.7, 21.0 ± 9.8, 18.1 ± 8.3, and 23.2 ± 10.3,
p < 0.001, respectively, for the common hepatic artery; 161 ± 23, 16.8 ± 6.6, 4.3 ± 1.9, and 10.9 ± 3.9,
p < 0.001, respectively, for the portal vein; 165 ± 25, 14.4 ± 5.2, 4.2 ± 1.9, and 13.9 ± 4.8,
p < 0.001, respectively, for the hepatic vein) and the QC (243 ± 45, 21.5 ± 10.1, 11.6 ± 4.6, and 15.4 ± 5.7,
p < 0.001, respectively, for the common hepatic artery; 140 ± 19, 19.9 ± 5.6, 3.0 ± 2.1, and 7.7 ± 4.8,
p < 0.001, respectively, for the portal vein; 142 ± 20, 19.1 ± 6.2, 2.7 ± 1.4, and 9.5 ± 3.9,
p < 0.001, respectively, for the hepatic vein) images. The subjective scores of the image quality of common hepatic artery, portal vein and hepatic vein in the optimal monochromatic images (4.7 ± 0.2, 4.5 ± 0.3, and 4.3 ± 0.4, respectively) were significantly (
p < 0.001) higher than in the 70 keV (4.0 ± 0.3, 3.9 ± 0.4, and 3.8 ± 0.4, respectively) and QC (3.6 ± 0.4, 3.3 ± 0.3, and 3.2 ± 0.3, respectively) images (
Tables 1,
2,
3,
Fig. 1).
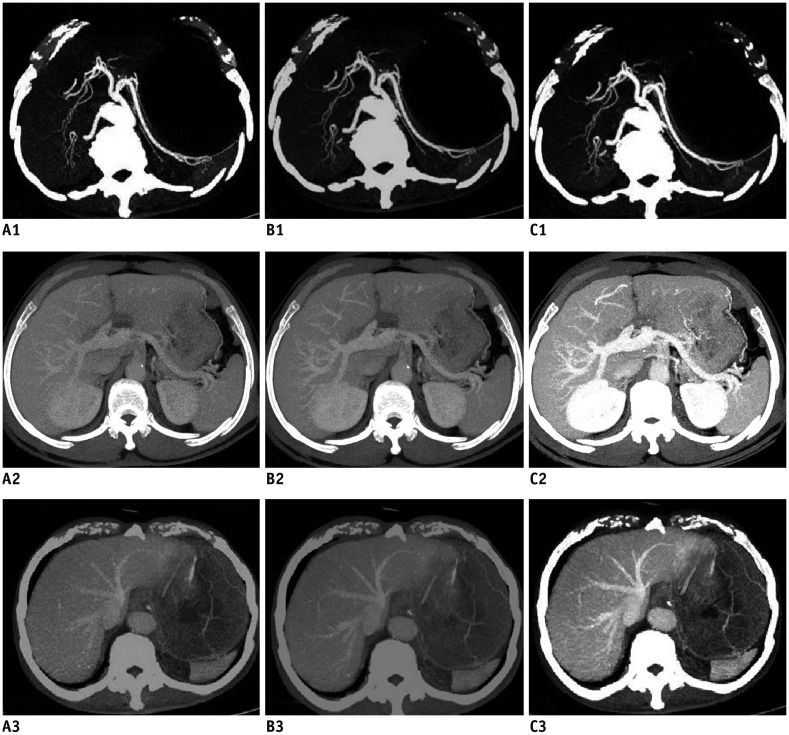 | Fig. 1
Comparison of images.
In phase of hepatic artery in QC (A1), in 50 keV (B1), and in 70 keV (C1). In phase of portal vein for QC (A2), in 50 keV (B2), and in 70 keV (C2). In phase of hepatic vein in QC (A3), in 50 keV (B3), and in 70 keV (C3). QC = quality check

|
Table 1
Comparison of Hepatic Artery under Monochromatic and Hybrid Spectrum

Image |
CT Value |
IN |
CNR |
SNR |
Score |
Optimal keV |
546 ± 97 |
38.0 ± 18.2 |
24.6 ± 10.9 |
28.3 ± 12.3 |
4.7 ± 0.2 |
QC |
243 ± 45*
|
21.5 ± 10.1*
|
11.6 ± 4.6*
|
15.4 ± 5.7*
|
3.6 ± 0.4*
|
70 keV |
287 ± 49.7*†
|
21.0 ± 9.8*
|
18.1 ± 8.3*†
|
23.2 ± 10.3*†
|
4.0 ± 0.3*†
|
p
|
< 0.001 |
< 0.001 |
< 0.001 |
< 0.001 |
< 0.001 |

Table 2
Comparison of Portal Vein under Monochromatic and Hybrid Spectrum

Image |
CT Value |
IN |
CNR |
SNR |
Score |
Optimal keV |
293 ± 45 |
27.4 ± 11.0 |
6.9 ± 2.7 |
17.6 ± 5.7 |
4.5 ± 0.3 |
QC |
140 ± 19*
|
19.9 ± 5.6*
|
3.0 ± 2.1*
|
7.7 ± 4.8*
|
3.3 ± 0.3*
|
70 keV |
161 ± 23*†
|
16.8 ± 6.6*
|
4.3 ± 1.9*†
|
10.9 ± 3.9*†
|
3.9 ± 0.4*†
|
p
|
< 0.001 |
< 0.001 |
< 0.001 |
< 0.001 |
< 0.001 |

Table 3
Comparison of Hepatic Vein under Monochromatic and Hybrid Spectrum

Images |
CT Value |
IN |
CNR |
SNR |
Score |
Optimal keV |
281 ± 49 |
24.7 ± 9.2 |
5.7 ± 2.3 |
14.9 ± 4.9 |
4.3 ± 0.4 |
QC |
142 ± 20*
|
19.1 ± 6.2*
|
2.7 ± 1.4*
|
9.5 ± 3.9*
|
3.2 ± 0.3*
|
70 keV |
165 ± 25*†
|
14.4 ± 5.2*
|
4.2 ± 1.9*†
|
13.9 ± 4.8†
|
3.8 ± 0.4*†
|
p
|
< 0.001 |
< 0.001 |
< 0.001 |
< 0.001 |
< 0.001 |

The CT value, CNR, SNR, and a subjective score of the common hepatic artery (celiac artery), portal vein and hepatic vein were significantly (p < 0.05) higher in the 70 keV images than in the QC images. There was no statistically (p > 0.05) significant difference in the IN between the two groups.