Abstract
Background
Senna, one of the major stimulant laxatives, is widely used for treating constipation. Chronic senna use has been reported to be associated with colonic disorders such as melanosis coli and/or epithelial hyperplasia. However, there is no obvious information on the influence of chronic senna use on organs except for the intestine.
Objective
To clarify the influence of senna laxative use on skin barrier function by repeated senna administration.
Methods
Eight-week-old male hairless mice received senna (10 mg/kg/day) for 21 days. After administration, we evaluated transepidermal water loss (TEWL), and investigated the biomarkers in plasma and skin using protein analysis methods.
Results
Fecal water content on day seven was significantly increased; however, on day 21, it was significantly decreased after repeated senna administration. In the senna-administered group, TEWL was significantly higher compared to the control on days seven and 21. Plasma acetylcholine concentration and NO2 −/NO3 − were increased on days seven and 21, respectively. In skin, tryptase-positive mast cells and inducible nitric oxide synthase (iNOS)-positive cells were increased on days seven and 21, respectively. The increase of TEWL on days seven and 21 was suppressed by the administration of atropine and N(G)-nitro-L-arginine methyl ester, respectively.
Conclusion
It was suggested that diarrhea or constipation induced by repeated senna administration caused the impairment of skin barrier function. There is a possibility that this impaired skin barrier function occurred due to degranulation of mast cells via cholinergic signals or oxidative stress derived from iNOS.
In the clinical setting, constipation is often observed. Constipation, although not life-threatening, can cause much discomfort affecting the quality of life. Laxatives are widely used for treating constipation. When a constipation symptom continues, a laxative will be administrated chronically. In a recent multi-national survey of patients with chronic constipation, between 16% and 40% reported that they used laxatives, with almost two-thirds using them on at least a monthly basis1.
Plants of senna that contain anthranoid derivatives such as sennoside are frequently used as cathartics. Chronic senna use has been reported to be associated with colonic disorders such as melanosis coli and/or epithelial hyperplasia2. However, there is no obvious information on the influence of chronic laxative use on organs except for the intestine. Our previous study revealed that mast cells and 3,4 dihydroxyphenylalanine-positive cells in the colon were increased after repeated administration of senna. Further, in skin, the number of Langerhans cells decreased by repeated senna administration3. The skin barrier function plays an important role in protecting against the penetration of microbial pathogens and allergens, and against excessive transepidermal water loss (TEWL)4. In the epidermis, Langerhans cells also maintain physiological homeostasis via immune reaction against foreign antigens5. Regarding immunological defenses, both the intestine and skin are very important. One of the major intestinal tract diseases is inflammatory bowel disease (IBD), which occasionally complicates skin disorders as extraintestinal manifestations6. Additionally, the skin barrier function was impaired via mast cells in a small intestine-injured mouse model7. Further, in mice with colitis or colon cancer, the impaired skin barrier function occurred with destruction of type I collagen89. These findings implicated a close relationship between the skin and colon. The skin barrier function may be affected by various intestinal diseases; however, the detailed mechanism is not clear. In this study, we investigated the effect of repeated senna administration on skin barrier function in mice.
Seven-week-old hairless Hos:HR-1 mice were obtained from Japan SLC, Inc. (Shizuoka, Japan) and housed in stainless steel wire mesh-bottomed cages with a constant temperature of 23℃±1℃, relative humidity of 50%±10%, and 12-hour light and dark cycles, as described previously3. Mice were provided laboratory chow and water ad libitum. After an acclimation period of 1 week, the mice were randomly assigned to four groups (n=5/group), including the control, sennoside, sennoside+atropine, and sennoside+N(G)-nitro-L-arginine methyl ester (L-NAME) groups. Mice in the sennoside, sennoside+ atropine, and sennoside+L-NAME groups were given senna (10 mg/kg/day, postorally; Suzu Pharmaceutical Co., Ltd., Osaka, Japan) once a day for 21 days. Further, the sennoside+atropine and sennoside+L-NAME groups were intraperitoneally administered with atropine (1 mg/kg/day, once a day; Wako, Osaka, Japan) and L-NAME (20 mg/kg/day, once a day; Cayman Chemical, Ann Arbor, MI, USA), respectively. All mice were killed after 21 days of treatment with pentobarbital anesthesia. The animal protocol for this study was approved by the Animal Care Regulations Committee of the Suzuka University of Medical Science.
On days zero, seven, 14, and 21 after administration, the body weight, fecal weight, and fecal water content were measured. Feces were collected and weighed when seen, and then dried for >24 hours at 70℃ in a ventilated oven. The fecal water content was calculated from the difference between the fecal wet weight and dry weight, and the fecal moisture percentage was obtained as the ratio of fecal water content to fecal wet weight.
TEWL and the skin hydration levels of the dorsal skin were respectively measured using a Tewameter® TM300 and Corneometer® CM825 (Courage+Khazaka Electronic GmbH, Cologne, Germany) on days zero, seven, 14, and 21, according to previously described methods7.
All mice were euthanized using pentobarbital anesthesia on days zero, seven, 14, and 21. Immediately following euthanization, a 1 ml blood sample was collected from each mouse by cardiac puncture. We measured the plasma acetylcholine concentrations using an Acetylcholine Assay Kit (Cell Biolabs, San Diego, CA, USA). We measured plasma tumor necrosis factor alpha (TNF-α) and interferon gamma (IFN-γ) using the respective enzyme-linked immunosorbent assay kits (R&D Systems, Minneapolis, MN, USA and eBioscience Inc., San Diego, CA, USA, respectively) according to the manufacturer's instructions. Plasma NO2 −/NO3 − levels were also measured as a surrogate marker for inducible nitric oxide synthase (iNOS) expression using the NO2/NO3 Assay Kit-C II (Colorimetric) and Griess Reagent Kit (DOJINDO Molecular Technologies, Inc., Kumamoto, Japan) for each nitric oxide (NO) radical.
After euthanization and blood sampling, tissue samples were isolated from the dorsal skin, followed by fixation in phosphate buffered saline (PBS) containing 4% paraformaldehyde. Fixed tissue specimens were embedded in Tissue Tek Optimum Cutting Temperature Compound (Sakura Finetek, Tokyo, Japan), frozen, and cut into 5-µm thick sections. Sections were then stained with hematoxylin and eosin (H&E) according to established procedures. Other skin specimens were washed with PBS and incubated overnight at 4℃ with one of the following primary antibodies: goat polyclonal anti-mast cell tryptase (1:50; Santa Cruz Biotec, Inc., Santa Cruz, CA, USA) and rabbit polyclonal anti-iNOS (1:100; Santa Cruz Biotec, Inc.). After staining, specimens were washed in PBS and incubated at room temperature for 2 hours with fluorescein isothiocyannate-conjugated anti-goat or anti-rabbit immunoglobulin. Protein expression was evaluated immunohistochemically with a BX51 fluorescent microscope (Olympus, Tokyo, Japan). The number of tryptase-positive mast cells or iNOS-positive cells was determined using the software program ImageJ (National Institutes of Health, Bethesda, MD, USA).
Fig. 1 shows the transition of body weight (Fig. 1A), fecal weight (Fig. 1B), and fecal water content (Fig. 1C). Body weight in the sennoside group was gradually decreased compared with that in the control over 21 days. The feces weight and fecal water content in the sennoside group increased to approximately 1.5 times that in the control on day seven, and diarrhea was observed. However, on day 21, the feces weight and fecal water content in the sennoside group were almost half that in the control. Fig. 1D shows H&E stain after treatment. There was no apparent skin disruption in both control and sennoside group.
The transition of plasma concentrations of acetylcholine, TNF-α, IFN-γ, and NO2 −/NO3 − over 21 days are shown in Fig. 2. Senna administration resulted in a drastic increase in plasma acetylcholine on day seven. However, the plasma acetylcholine concentrations in the sennoside group was decreased, and were the same level as that of the control on days 14 and 21. Conversely, the plasma levels of TNF-α, IFN-γ, and NO2 −/NO3 − in the sennoside group were similar compared to those in the control on days seven and 14; however, they were significantly increased on day 21.
Immunohistochemical analysis of mast cell tryptase and inducible NO in the skin of mice is shown in Fig. 3. The number of tryptase-positive mast cells in the skin of mice was significantly higher in the sennoside group than in the control on day seven. On days 14 and 21, there were no significant differences in the number of tryptase-positive mast cells between the control and sennoside groups. The induction of iNOS-positive cells in the sennoside group was increased over time. The number of iNOS-positive cells was significantly higher in the sennoside group than in the control on days 14 and 21.
After coadministration of atropine or L-NAME, transition of body weight, fecal weight, plasma acetylcholine, TNF-α, and NO2 −/NO3 − in sennoside+atropine group and in sennoside+L-NAME group were similar to that in sennoside group (Fig. 4A~E). In sennoside+atropine group, significant increase in iNOS positive cells on day 21 was observed. On the other hand, significant increase in mast cell tryptase on day 7 was observed in sennoside+ L-NAME group (Fig. 4F, G).
Fig. 5 shows the transition of TEWL and the skin hydration level over 21 days. There were significant differences in TEWL between the sennoside and control groups on days seven and 21. Likewise, there were significant differences in the skin hydration levels between the sennoside and control groups on days seven and 21 (Fig. 5A). However, significant differences in TEWL and the skin hydration levels on day seven were not observed between the sennoside+ atropine and control groups (Fig. 5B). Further, significant differences in TEWL and the skin hydration levels on day 21 were not recognized between the sennoside+ L-NAME and control groups (Fig. 5C).
In the present study, the impairment of skin barrier function was caused by repeated senna administration in hairless mice. Diarrhea and constipation occurred sequentially on days seven and 21 by daily senna administration, respectively. According to the transition of fecal symptoms, mast cell tryptase and iNOS-derived NO in the skin of hairless mice were increased on days seven and day 21, respectively. Therefore, mast cell tryptase and oxidative damage by NO seemed to be key factors in the pathogenesis of impaired skin barrier.
Rhein, the active metabolite of sennoside laxatives, activates chloride secretion by excitation of submucosal neurons and the release of acetylcholine10. In our study, administration of senna for seven days caused diarrhea, and plasma acetylcholine concentration was increased (Fig. 2A). Further, increased TEWL and a decreased skin hydration level were observed on day seven, which indicated destruction of the skin barrier (Fig. 5A). Concomitant administration of atropine, which is an antagonist of acetylcholine receptor (AChR), recovered the same levels of TEWL and skin hydration at baseline (Fig. 5B). These data indicated that the impairment of skin barrier function on day seven was associated with acetylcholine. On day seven, there was a significant increase of mast cells in the skin (Fig. 3A). Mast cells have AChRs, and degranulation of mast cells is regulated by these receptors111213. Destruction of the skin barrier on day seven may have involved the degranulation of mast cells via cholinergic signals. Further, there are several neuronal and/or non-neuronal cholinergic systems in the skin1415. Nicotinic and muscarinic AChRs colocalize on the mast cell surface. These ligand-activated receptors are positively and negatively regulated1617. In addition to mast cells, various cells such as keratinocyte and fibroblast maintain skin homeostasis through these cholinergic systems1819. They may exhibit diverse actions through the cholinergic system. It is thought that a complicated outbreak mechanism of impaired skin barrier function via acetylcholine exists.
Conversely, on day 21, the moisture content of feces and the fecal weight were significantly decreased (Fig. 1B, C), which indicated that constipation occurred in mice. Repeated senna administration caused functional intestinal disturbance20 and epithelial hyperplasia21. Therefore, it was suggested that structural change of the intestine was also caused in mice receiving senna. In IBD, one of the representative diseases of the intestine, the colonic epithelial hyperplasia is dependent on iNOS22. TNF-α and IFN-γ produced by IBD lead to the production of iNOS23, which introduce NO2425. Repeated senna administration in hairless mice also induced the upregulation of TNF-α and IFN-γ; further, NO2 −/NO3 −, which is a surrogate marker of NO, was also increased (Fig. 2B~D). Accordingly, oxidative stress derived from NO may participate in the onset of epithelial hyperplasia due to chronic senna administration. Furthermore, in skin, iNOS-positive cells were increased on day 21 (Fig. 3B), and skin barrier impairment occurred (Fig. 5A). Damage to the skin barrier on day 21 was ameliorated by the administration of L-NAME, which is an iNOS inhibitor. It is suggested that the impairment of skin barrier function on day 21 was caused by oxidative stress derived from iNOS induced by TNF-α and IFN-γ. To clarify the detailed mechanisms of skin disruption, we will perform another experiment and administer an anti-tryptase antibody or antioxidant to directly block tryptase or oxidative stress in further research. There have been several reports about the toxicity of chronic senna administration on the intestine2627, however, there is no report about the toxicity on skin. Significant body weight loss was observed after senna treatment, however, weight loss was less likely to have an influence on the skin barrier function because similar tendency was also observed in other groups. This current study suggests that repeated senna administration may aggravate underlying diseases of the skin such as atopic dermatitis and senile xerosis. The number of tryptase-positive mast cells and iNOS-positive cells in skin varied according to the duration of senna administration. Therefore, the onset mechanisms of impaired skin barrier function would be different on days seven and 21. Further study to clarify the role of molecular markers involved in neuronal and/or non-neuronal signal transduction from the intestine to the skin is needed.
This study suggested the possibility that repeated senna administration affects the skin barrier function. Clinicians and pharmacists should pay attention to the skin as well as the intestine in patients receiving long-term senna treatment, especially in those who are suffering from dermatoses.
Figures and Tables
Fig. 1
Effects of repeated senna administration on mice (A) body weight, (B) fecal weight, and (C) fecal water content. Blue circle is the control group. Red circle is the sennoside group. (D) H&E stain in control and sennoside group. *p<0.05 compared with the control. Scale bar=100 µm.
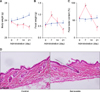
Fig. 2
Plasma concentration of (A) acetylcholine, (B) tumor necrosis factor alpha (TNF-α), (C) interferon gamma (IFN-γ), and (D) NO2 −/NO3 −. Blue circle is the control. Red circle is the sennoside group. *p<0.05 compared with the control.
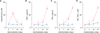
Fig. 3
(A) Immunohistochemistry of mast cell tryptase (arrows) and the number of mast cell tryptase in the skin. (B) Immunohistochemistry of inducible nitric oxide synthase (iNOS) (arrows) and the number of iNOS-positive cells in the skin. Blue circle is the control. Red circle is the sennoside group. *p<0.05, **p<0.01 compared with the control. Scale bar=100 µm.
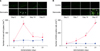
Fig. 4
Transition of (A) body weight, (B) fecal weight, (C) plasma acetylcholine, (D) tumor necrosis factor alpha (TNF-α), (E) NO2 −/NO3 −, (F) number of mast cell tryptase, and (G) inducible nitric oxide synthase (iNOS) positive cells. Blue circle is the control. Green square is the sennoside+atropine group. Red triangle is the sennoside+L-NAME group. *p<0.05 compared with the control. L-NAME: N(G)-nitro-L-arginine methyl ester.
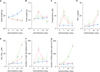
Fig. 5
Transition of TEWL and the skin hydration level over 21 days. (A~C) Blue circle is the control. (A) Red circle is the sennoside group. (B) Green square is the sennoside+atropine group. (C) Open triangle is the sennoside+L-NAME group. *p<0.05 compared with the control. TEWL: transepidermal water loss, L-NAME: N(G)-nitro-L-arginine methyl ester.
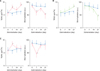
References
1. Wald A, Scarpignato C, Mueller-Lissner S, Kamm MA, Hinkel U, Helfrich I, et al. A multinational survey of prevalence and patterns of laxative use among adults with self-defined constipation. Aliment Pharmacol Ther. 2008; 28:917–930.


2. Morales MA, Hernandez D, Bustamante S, Bachiller I, Rojas A. Is senna laxative use associated to cathartic colon, genotoxicity, or carcinogenicity? J Toxicol. 2009; 2009:287247.


3. Yamate Y, Hiramoto K, Yokoyama S, Ooi K. Immunological changes in the intestines and skin after senna administration. Pharm Biol. 2015; 53:913–920.


4. Matsui T, Amagai M. Dissecting the formation, structure and barrier function of the stratum corneum. Int Immunol. 2015; 27:269–280.


5. Kubo A, Nagao K, Yokouchi M, Sasaki H, Amagai M. External antigen uptake by Langerhans cells with reorganization of epidermal tight junction barriers. J Exp Med. 2009; 206:2937–2946.


6. Huang BL, Chandra S, Shih DQ. Skin manifestations of inflammatory bowel disease. Front Physiol. 2012; 3:13.


7. Yokoyama S, Hiramoto K, Koyama M, Ooi K. Skin disruption is associated with indomethacin-induced small intestinal injury in mice. Exp Dermatol. 2014; 23:659–663.


8. Yokoyama S, Hiramoto K, Koyama M, Ooi K. Impaired skin barrier function in mice with colon carcinoma induced by azoxymethane and dextran sodium sulfate. Biol Pharm Bull. 2015; 38:947–950.


9. Yokoyama S, Hiramoto K, Koyama M, Ooi K. Impairment of skin barrier function via cholinergic signal transduction in a DSS-induced colitis mouse model. Exp Dermatol. 2015; 24:779–784.


10. Frieling T, Rupprecht C, Schemann M. Rhein stimulates electrogenic chloride secretion by activation of submucosal neurons in guinea pig colon. Pharmacology. 1993; 47:Suppl 1. 70–76.


11. Blandina P, Fantozzi R, Mannaioni PF, Masini E. Characteristics of histamine release evoked by acetylcholine in isolated rat mast cells. J Physiol. 1980; 301:281–293.


12. Radosa J, Dyck W, Goerdt S, Kurzen H. The cholinergic system in guttate psoriasis with special reference to mast cells. Exp Dermatol. 2011; 20:677–679.


13. Masini E, Fantozzi R, Conti A, Blandina P, Brunelleschi S, Mannaioni PF. Mast cell heterogeneity in response to cholinergic stimulation. Int Arch Allergy Appl Immunol. 1985; 77:184–185.


14. Roosterman D, Goerge T, Schneider SW, Bunnett NW, Steinhoff M. Neuronal control of skin function: the skin as a neuroimmunoendocrine organ. Physiol Rev. 2006; 86:1309–1379.


15. Kurzen H, Wessler I, Kirkpatrick CJ, Kawashima K, Grando SA. The non-neuronal cholinergic system of human skin. Horm Metab Res. 2007; 39:125–135.


16. Kageyama-Yahara N, Suehiro Y, Yamamoto T, Kadowaki M. IgE-induced degranulation of mucosal mast cells is negatively regulated via nicotinic acetylcholine receptors. Biochem Biophys Res Commun. 2008; 377:321–325.


17. Sudheer PS, Hall JE, Donev R, Read G, Rowbottom A, Williams PE. Nicotinic acetylcholine receptors on basophils and mast cells. Anaesthesia. 2006; 61:1170–1174.


18. Buchli R, Ndoye A, Rodriguez JG, Zia S, Webber RJ, Grando SA. Human skin fibroblasts express m2, m4, and m5 subtypes of muscarinic acetylcholine receptors. J Cell Biochem. 1999; 74:264–277.


19. Kurzen H, Henrich C, Booken D, Poenitz N, Gratchev A, Klemke CD, et al. Functional characterization of the epidermal cholinergic system in vitro. J Invest Dermatol. 2006; 126:2458–2472.


20. Nadal SR, Calore EE, Manzione CR, Puga FR, Perez NM. Effects of long-term administration of Senna occidentalis seeds in the large bowel of rats. Pathol Res Pract. 2003; 199:733–737.


21. Mengs U, Mitchell J, McPherson S, Gregson R, Tigner J. A 13-week oral toxicity study of senna in the rat with an 8-week recovery period. Arch Toxicol. 2004; 78:269–275.


22. Schreiber O, Petersson J, Walden T, Ahl D, Sandler S, Phillipson M, et al. iNOS-dependent increase in colonic mucus thickness in DSS-colitic rats. PLoS One. 2013; 8:e71843.


23. Fakhoury M, Negrulj R, Mooranian A, Al-Salami H. Inflammatory bowel disease: clinical aspects and treatments. J Inflamm Res. 2014; 7:113–120.


24. Xie QW, Whisnant R, Nathan C. Promoter of the mouse gene encoding calcium-independent nitric oxide synthase confers inducibility by interferon gamma and bacterial lipopolysaccharide. J Exp Med. 1993; 177:1779–1784.


25. Colon AL, Menchen LA, Hurtado O, De Cristobal J, Lizasoain I, Leza JC, et al. Implication of TNF-alpha converttase (TACE/ADAM17) in inducible nitric oxide synthase expression and inflammation in an experimental model of colitis. Cytokine. 2001; 16:220–226.

