Abstract
Purpose
Group A rotavirus (RV) is most common etiologic agent of acute gastroenteritis (AGE) in children worldwide. Recently, vaccination has been introduced in several countries to reduce the disease burden caused by RV infections, but continuous surveillance of RV strains is necessary to detect the emergence of potential variants induced by vaccine-immune pressure. This study aimed to investigate the changing pattern of RV genotypes in children with AGE, following the introduction of vaccination in Korea.
Methods
Genotyping of RVs by RT-PCR on the basis of VP7 and VP4 gene segment sequence was carried out on 201 rotavirus-positive stool samples, from children hospitalized with AGE between August 2009 and June 2013. We have directly sequenced PCR products and analyzed the phylogenetic tree.
Results
The most prevalent G genotype was G9 (33.3%), followed by G1 (22.4%), G3 (15.9%), G2 (6.0%), G4 (3.0%), G10 (1.5%), and mixed G-type (15.4%), with some nontypeable cases (2.5%). The detected P genotypes were P[4] (45.3%), P[8] (43.8%), mixed P-type (10.4%), and P[2] (0.5%). The G9P[4] genotype was predominantly observed in hospitalized cases in Seoul in 2010/2011, however G1P[8] has been re-emerged as the predominant genotype in the following season (P=0.004).
Acute gastroenteritis (AGE) is the one of important causes of hospitalization in children in both developing as well as developed countries and Group A rotaviruses (RVA) are the most common cause of AGE among children. Recently, RV vaccines have been introduced in several countries including Korea to reduce the disease burden caused by RV infections. However, continuous surveillance of RV strains is necessary to detect the possible emergence of potential variants induced by vaccine-immune pressure because RV shows genetic diversity due to frequent point mutation and re-assortment. RVA belongs to the Reoviridae family, which is characterized by a segmented doublestranded RNA genome; RVA contains 11 gene segments encoding structural proteins (VP1-4, VP6, and VP7) and nonstructural proteins (NSP1-5/6)1). RVs are classified on the basis of their VP7 and VP4 gene sequences, and 27 G and 37 P genotypes have been reported2); the major genotypes reported worldwide are the G1P[8], G2P[4], G3P[8], G4P[8], G9P[8] combination genotypes, and G123). In Korea, the G1 genotype was predominant in the 2000's, followed by G9, G3 and G4 genotypes4). Recently, re-emergence of G9 and subsequent increase of G1 and G3 have been reported5). A classification system that incorporates all 11 gene segments has been proposed1), which would be useful for delineating the reassortment of genomes due to zoonotic transmission or immune selection pressure following the introduction of vaccination6). Although frequent detection of some genotypes such as G2 or G3 was has been reported after introduction of vaccines in some countries78), it is uncertain whether these findings were due to natural seasonal variation or immune pressure induced by mass campaign. RV vaccines have been introduced in Korea in June 2007 (RotaTeq® vaccine) and March 2008 (Rotarix® vaccine). The overall vaccination rate was 30% in 2009, and 34.1 % (Rotarix® vaccine) and 26.1% (RotaTeq® vaccine) in 2012910). However, the data for the change of RV genotypes after introduction of vaccines is still lacking in Korea. The purpose of this study was to investigate the epidemiology and genotypic change of RV following the introduction of vaccination in Korea during 2009–2013.
Stool samples were obtained from children with AGE who were treated at Sanggyepaik Hospital from August 2009 to June 2013. The initial identification of RV was carried out using an enzyme immunoassay (EIA) kit, Rotaclone® (Meridian Bioscience, Cincinnati, OH, USA) and RV-positive stool samples were included in this study. The study was approved by the Institutional Review Board of Sanggyepaik Hospital, Inje University, Seoul, Korea. Samples were obtained after receiving informed written consent from a patient's parents. The stool samples were diluted 1:10 (weight/volume) with phosphate-buffered saline, homogenized using a vortex mixer, and subjected to centrifugation at 5,000× g for 30 min at 4℃. Supernatants were recovered and stored at −70℃ until use.
Total RNA was extracted from stool samples using the QIAamp Viral RNA kit (Qiagen GmbH, Hilden, Germany), and RV sequences were then amplified via reverse transcription-polymerase chain reaction (RTPCR), using nested primers. RT-PCR was carried out on the 201 RV-positive samples in order to genotype the RV on the basis of VP7 and VP4 gene segment sequence. G genotyping was performed using a pool of different primers specific for G1-G4, G8, G9, G10, and G121112). The VP4 gene was characterized by using a pool of different P genotype-specific primers for P[4], P[6], and P[8]-P[10]13). An 881-bp fragment of the VP7 gene amplified in the first round of PCR, using VP7 consensus primers, was sequenced14). For PCR amplification of the VP4 gene, an 876-bp fragment was generated using the Con3 forward primer and Con2 reverse primer13). For G9-positive samples (G9P[4]: 10 cases, and G9P[8]: 3 cases), which were chosen by implying the status and amounts of remnant samples, characterization of other gene segments that encode VP1-3, VP6, and NSP1-5 was carried out via RT-PCR, using specific primers for each gene segment1). Amplified products of PCR reactions, which were randomly selected, were purified using the QIAquick® PCR Purification kit (Qiagen), and were then directly sequenced using the BigDye® Terminator Cycle Sequencing kit (version 3.1; Life Technologies, Carlsbad, CA, USA). Sequencing reaction products were resolved using an Applied Biosystems® ABI 3730 XL DNA analyzer (Life Technologies) . The nucleotide sequences of the VP7 and VP4 genes were aligned using the Clustal W, and phylogenetic trees were constructed using the MEGA software, version 4.014). We compiled the nucleotide sequences that were determined in this study with those available in the GenBank database by using the BLAST program (http://blast.ncbi.nlm.nih.gov/). The VP7 and VP4 sequences identified in this study have been submitted to the GenBank database under the accession numbers; VP7 from KF812568 to KF812615, and VP4 from KF812 616 to KF812663, respectively.
Categorical variables among study periods were compared using Chi-square test for trend. A P-value <0.05 was considered as statistically significant. All statistical analysis was performed by MedCalc version 15.6.1 (MedCalc software, Mariakerke, Belgium).
Stool samples were obtained from a total of 1,147 children with AGE who were treated at Sanggyepaik Hospital from August 2009 to June 2013. A total of 201 RV-positive stool samples were included in this study after screening of RV using an EIA kit. The G and P genotypes were determined by RT-PCR for 201 RV-positive samples that were obtained from children hospitalized with AGE between August 2009 and June 2013. During the period covered by this study, the most common G genotype was G9 (33.3%, 67 cases), followed by G1 (22.4%, 45 cases), G3 (15.9%, 32 cases), G2 (6.0%, 12 cases), G4 (3.0%, 6 cases), G10 (1.5%, 3 cases), and mixed G-type (15.4 %, 31 cases), with some nontypeable cases (2.5%, 5 cases) (Fig. 1A). The G3 genotypes were most frequently detected in cases hospitalized between August 2009 and July 2010, but the G9 genotype had rapidly emerged as the predominant genotype between August 2010 and July 2012; after August 2010, there was an increase in cases of the G1 genotype, which re-emerged as a major genotype between August 2012 and June 2013 (P=0.004) (Table 1). These data show periodic fluctuation of the G1 and G9 genotypes between September 2007 and June 2013 (Fig. 1A). During the period covered by the present study, the detected P genotypes were P[4] (45.3%, 91 cases), P[8] (43.8%, 88 cases), mixed P-type (10.4%, 21 cases), and P[2] (0.5%, 1 case). The most frequent combinations of G/ P genotypes were G9P[4] (26.4%, 53 cases) and G1P [8] (19.4%, 39 cases), followed by G3P[8] (13.9%, 28 cases), and G9P[8] (7.0%, 14 cases) (Fig. 1B). Detection of a high rate of unusual combination genotypes such as G9P[4] is notable, as these were not observed in our previous study that covered the period September 2007–July 2009.
On the basis of VP7 gene sequence, the 14 G1 strain s clustered in lineage I, and shared close sequence similarity with the previously reported RV strain from Korea (KOR/Seoul-669/2009 HM130947), as well as with strains from Japan (OH3592/2012 AB796447) and China (CHN/2678 EU708572) (Fig. 2). The six G2 isolates clustered in lineage IV and V, which showed that they were genetically close to other G2 isolates from Korea (Kor/Seoul-397/2009 HM130951 and Kor/Seoul-558/2009 HM130953) (Fig. 2). The 12 G3 isolates clustered in lineage III, and shared close sequence similarity with G3 isolates from Finland (FIN/ HAL1166 L20882) and Korea (KOR/Seoul-502/2009 HM130957) (Fig. 2). The four G4 strains clustered in sublineage Ic and showed close sequence similarity to a G4 strain from the USA (GBR/ST3/1975/G4P6 EF672616) (Fig. 2). The nine G9 strains clustered in lineage III, and had high homology with strains from China (CHN/L463/2006 EU708596) and Korea (KOR/ Seoul-378/2008 HM130979) (Fig. 3). The three G10 isolates clustered in lineage IX, and shared close sequence similarity with the G10 strains from Australia (for example, AUS/V585/2011/G10P14 JX567749) (Fig. 3). The genetic relationships of RV isolates from this study were also determined on the basis of VP4 gene sequence. Of the P[8] strains, 27 clustered in sublineage IIIA, and showed sequence similarity with previously reported P[8] strains from Korea (KOR/Seoul-291/2008/ G1P HM130991 and KOR/KMR004/2000/G1P8 EF0773 30) (Fig. 3). Of the P[4] isolates, 21 clustered in lineage V, and showed high sequence similarity with strains isolated from Korea (KOR/Seoul-710/2009/ G2P4 HM131053 and KOR/Seoul-620/2009/G3P4 HM131054) (Fig. 3). In thirteen G9 isolates, all gene segments belonged to the Wa-like genotype 1 constellation, but one isolate (SE-11-04-169) showed I2 and R2 genotypes for the VP6 and VP1 genes, respectively (Fig. 5).
Comparison of VP7 antigenic regions of Korean G1 isolates, identified in this study, with both vaccine strains (Rotarix® G1P[8] and RotaTeq® G1P[5]) revealed conservation of the six amino acid residues in the 7-1b antigenic site, but showed different amino acid residues at positions 91, 100, and 123 in the 7-1a region, and at position 217 in the 7-2 region (Fig. 4A). Among Korean G9 isolates identified in this study, there were 12 amino acid changes when compared to the Rotarix® G1 strain and the RotaTeq ®G1 strain across the three antigenic sites. Korean G4 isolates showed different amino acid residues in five positions when compared to the RotaTeq® G4 strain across the three antigenic sites. Within the VP4 antigenic epitopes of the Korean P[8] isolates, amino acid differences were noted at four or five positions in comparison with the RotaTeq® strain, and at ten positions in comparison with the Rotarix® strain. Korean P[4] isolates showed divergent amino acid sequences when compared to the P[8] epitopes of the vaccine strains (17 amino acid differences with the Rotarix® strain, and 16 with the RotaTeq® strain) (Fig. 4B)
Between August 2009 and June 2013, the predominan t G genotype of human RV in Seoul, Korea successively changed. When compared to the results of our previous study6), it appears that the predominant G genotypes (G1, G3, and G9) naturally fluctuated, despite RV vaccination being introduced to Korea in 2007. Following the introduction of vaccination, a statistically significant decrease of the disease burden due to RV was reported in Korea16).
Interestingly, G1P[8] strains increased towards the end of this study. G1 lineage I was predominant throughout the study period and differences of amino acid residues at three positions (7-1a region) and one position (7-2 region) was revealed by comparison of VP7 antigenic regions of Korean G1 strains with both vaccine strains. Sublineage P[8]IIIA was most prevalent during the study period, consistent with previous findings in Brazil in which the definition of 1.5% cutoff value was proposed17). Continuous surveillance of the G1P[8] lineages are required to investigate any long term effect of vaccination on G1P[8] strain evolution.
combination genotype was observed and initially attributed to immune pressure following the introduction of the Rotarix® vaccine7), but it was later considered as a consequence of natural seasonal variation, in a study that showed frequent detection of the G2P[4] genotype in neighboring countries without a RV vaccination campaign1819). In Korea, the G2P[4] genotype was detected in 4.0–18.3% of cases between 2000 and 200920212223). In the present study, the G2P[4] genotype was detected in 4.9% of cases, which is similar to the incidence (4.0–4.3%) in other Korean studies that were carried out after the introduction of RV vaccination in 2007524). Frequent detection of the G3 genotype after the introduction of the RotaTeq® vaccine has been reported in Australia and the USA825). In the present study, the G3 genotype was mostly found in patients hospitalized in 2009/2010, but the detection rate of this genotype has fluctuated each year since the preintroduction of RV vaccine in Korea521). These results suggest that the prevalence of the G3 genotype might be unaffected by immune pressure following the introduction of RV vaccine.
In this study, the G4 genotype clustered in sublineage Ic, which was of interest because worldwide outbreaks due to this sublineage have been reported in several countries2627). The G12 genotype, a novel genotype recently detected in several countries, including Korea2528) was not found in the present study, however, another unusual genotype, G10, was found in three cases that occurred in 2011. This finding showed that re-assortment between human and bovine RV strains have occurred in Korea as in Africa29). The surveillance of animal RV strains is important because unusual strains could affect the diversity of human RV population. Collectively, our results for cases from the years 2009–2013 suggest that the emergence of unusual G genotypes due to vaccine pressure has not yet occurred in Korea, but it is necessary to remind that this study could not be the representatives of RV strain in the whole Korea during the same period.
In this study, circulating rotavirus isolates were highly diverse and mixed infections was most frequently found (26.7%) between August 2010 and July 2011. These results suggest the possible association between high rate of mixed infections of RV and the extent of strain diversity of circulating RV, which was consistent with those of a recent study30). In this study, 2.5% (5 cases) was non-typeable, which might be attributed to low concentration of virus particles or PCR inhibitors. The dominance of nontypeable isolates in adults with acute gastroenteritis was observed in a recent Indian study31).
Interestingly, the G9P[4] combination genotype was frequently detected in this study. Outbreaks of the unusual G9P[4] combination genotype have been reported in Mexico and Bangladesh3233), and were attributed to a possible reassortment in response to immune pressure. In Korea, the G9P[4] genotype was first identified in Gyeonggi province during 2003–2005 (detection rate: 1.7%)20), but this genotype was rarely found (range of detection rate: 0.04–0.7%) in later studies62124) prior to this study; these findings are similar to those of a recent study in Latin America18). In the present study, phylogenetic analysis of the G9 and P[4] genotypes showed that they clustered in lineages III and V, respectively. Further characterization of remnant gene segments in 10 G9P[4] isolates and three G9P[8] isolates revealed that all of them, except one isolate, belonged to the Wa-like genotype 1 constellation. These findings differ from those of a previous study in Mexico, which showed the predominance of the E6 genotype in the NSP4 gene33); the human-porcine reassortment of the NSP2 and NSP3 genes was not observed here, either. In the present study, we compared the amino acid residues in antigenic epitopes of VP7 and VP4 between the circulating isolates and the vaccine strains in Korea, which revealed less antigenic variation than in a comparable recent study in Bangladesh34). In a recent Finnish study35), there were no amino acid changes affecting the G1 genotype of VP7 and VP8*, despite RotaTeq ® being exclusively used for vaccination after 2009, which suggests that there is no evidence of selection pressure. This study has some limitations: 1) lack of RV vaccination history and 2) using a primer amplifying about 40% of the VP4 segment. It had been suggested recently at least 50% of sequence were required from a segment for proper classification1).
In conclusion, periodic fluctuation of RV genotypes such as G1, G3, and G9 has been observed in Korea during the period 2009–2013, following the introduction of vaccination. Predominance of the unusual G9P[4] combination genotype in 2011 was initially considered to be evidence of immune pressure due to vaccination, but the subsequent re-emergence of G1P[8] in 2013 suggests the possibility of natural fluctuation of genotypes. In addition, we could not detect any significant differences in sublineages of either G9 or P[4], or in the entire constellation of RV gene segments. However , it is still necessary to continuously monitor and characterize RV strains, in order to detect novel strains that emerge due to the immune pressure associated with mass vaccination.
Figures and Tables
Fig. 1
(A) Distribution of VP7 genotypes of rotavirus from children hospitalized at Sanggyepaik Hospital between August 2007 and June 2013. (B) G/P combinations of rotavirus at Sanggyepaik Hospital between August 2009-June 2013. *The results of our previous study [5] between September 2007 and July 2009 was included to show the evolution of G type according to year.
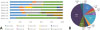
Fig. 2
Phylogenetic analysis of VP7 nucleotide sequences of genotype G1, G2, G3, and G4 strains. The tree was constructed using the Kimura 2-parameter and neighbor-joining methods in the MEGA software, version 4.0. Bootstrap values are shown at the branch nodes. Strains from other lineages and sublineages isolated worldwide are used for comparison. The lineages and sublineages are indicated at the right-hand side. The strains from this study are indicated in bold. The relevant GenBank accession numbers are indicated after the strain designation. The abbreviations are as follows: AUS Australia, BAN Bangladesh, BRA Brazil, CHN China, GHA Ghana, JPN Japan, KOR Korea, SA South Africa, THA Thailand, TPE Taipei, USA United States of America, VIE Vietnams, BEL Belgium, CHN China, FIN Finland, GBR Great Britain, HUN Hungary, ITA Italy, and RUS Russia.
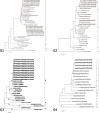
Fig. 3
Phylogenetic analysis of genotype G9, G10, P[8], and P[4] strains. The tree was constructed using the Kimura 2-parameter and neighbor-joining methods in the MEGA software, version 4.0. Bootstrap values are shown at the branch nodes. Strains from other lineages and sublineages isolated worldwide are used for comparison. The lineages and sublineages are indicated at the right-hand side. The strains from this study are indicated in bold. The relevant GenBank accession numbers are indicated after the strain designation. The abbreviations are as follows: AUS Australia, BAN Bangladesh, BRA Brazil, CHN China, CMR Cameroon, GBR Great Britain, IND India, JPN Japan, KOR Korea, THA Thailand, USA United States of America, VIE Vietnam, ITA Italy, MAW Malawi, PHI Philippines, RUS Russia, SLO Slovenia, and TPE Taipei.
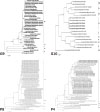
References
1. Matthijnssens J, Ciarlet M, McDonald SM, Attoui H, Banyai K, Brister K, et al. Uniformity of rotavirus strain nomenclature proposed by the Rotavirus Classification Working Group (RCWG). Arch Virol. 2011; 156:1397–1413.


2. Matthijnssens J, Van Ranst M. Genotype constellation and evolution of group A rotaviruses infecting humans. Curr Opin Virol. 2012; 2:426–433.


3. Banyai K, Laszlo B, Duque J, Steele AD, Nelson EA, Gentsch JR, et al. Systemic review of regional and temporal trends in global rotavirus strain diversity in the pre rotavirus vaccine era: insights for understanding the impact of rotavirus vaccine programs. Vaccine. 2012; 30:A122–A130.
4. Than VT, Jeong S, Kim W. A systemic review of genetic diversity of human rotavirus circulating in South Korea. Infect Genet Evol. 2014; 28:462–491.
5. Han TH, Kim CH, Chung JY, Park SH, Hwang ES. Genetic characterization of rotavirus in children in South Korea from 2007 to 2009. Arch Virol. 2010; 155:1663–1673.


6. Carvalho-Costa FA, Araujo IT, de Assis RMS, Fialho AM, Martins CM, Boja MN, et al. Rotavirus genotype distribution after vaccine introduction, Rio de Janeiro, Brazil. Emerg Infect Dis. 2009; 15:95–97.


7. Gurgel RQ, Cuevas LE, Vieira SC, Barros VC, Fontes PB, Salustino EF, et al. Predominance of rotavirus P[4]G2 in a vaccinated population, Brazil. Emerg Infect Dis. 2007; 13:1571–1573.


8. Kirkwood CD, Boniface K, Barnes GL, Bishop RF. Distribution of rotavirus genotypes after introduction of rotavirus vaccines, Rotarix® and RotaTeq®, into the National Immunization Program of Australia. Pediatr Infect Dis J. 2011; 30:S48–S53.


9. Choe YJ, Yang JJ, Park SK, Choi EH, Lee HJ. Comparative estimation of coverage between national immunization program vaccines and non-NIP vaccines in Korea. J Korean Med Sci. 2013; 28:1283–1288.


10. Lee SG, Jeon SY, Kim KY. 2012 Korea National Immunization Survey [internet]. Korean CDC;cited 2014 Aug 4. Available from http://www.cdc.go.kr/CDC/info/CdcKrInfo0201.jsp?menuIds=HOME001-MNU1155-MNU1083-MNU1375-MNU0025&cid=20768.
11. Banerjee I, Ramani S, Primrose B, Iturriza-Gomara M, Gray JJ, Brown DW, et al. Modification of rotavirus multiplex RT-PCR for the detection of G12 strains based on characterization of emerging G12 rotavirus strains from South India. J Med Virol. 2007; 79:1413–1421.


12. Gouvea V, Glass RI, Woods P, Taniguchi K, Clark HF, Forrester B, et al. Polymerase chain reaction amplification and typing of rotavirus nucleic acid from stool specimens. J Clin Microbiol. 1990; 28:276–282.


13. Gentsch JR, Glass RI, Woods P, Gouvea V, Flores J, Das BK, et al. Identification of group A rotavirus gene 4 types by polymerase chain reaction. J Clin Microbiol. 1992; 30:1365–1373.


14. Iturriza-Gomara M, Kang G, Gray J. Rotavirus genotyping: keeping up with an evolving population of human rotaviruses. J Clin Virol. 2004; 31:259–265.


15. Tamura K, Dudley J, Nei M, Kumar S. MEGA 4: Molecular evolutionary genetics analysis (MEGA) software version 4.0. Mol Biol Evol. 2007; 24:1596–1599.


16. Choi UY, Lee SY, Ma SH, Jang YT, Kim JY, Kim HM, et al. Epidemiological changes in rotavirus gastroenteritis in children under 5 years of age after the introduction of RV vaccines in Korea. Eur J Pediatr. 2013; 172:947–952.


17. da Silva MF, Gómez MM, Rose TL, Volotão Ede M, Carvalho-Costa FA, Bello G, et al. VP8*P[8] lineages of group A rotaviruses circulating over 20 years in Brazil: proposal of six different sub-lineages for P[8]-3 clade. Infect Genet Evol. 2013; 16:200–205.


18. Linhares AC, Stupka JA, Ciapponi A, Bardach AE, Glujovsky D, Aruj PK, et al. Burden and typing of rotavirus group A in Latin America and the Caribbean: systematic review and meta-analysis. Rev Med Virol. 2011; 21:89–109.


19. Martinez M, Amarilla AA, Galeano ME, Aquino VH, Farina N, Russomando G, et al. Predominance of rotavirus G2P[4] and emergence of G12P[9] strains in Asuncion, Paraguay, 2006–2007. Arch Virol. 2010; 155:525–533.


20. Huh JW, Kim WH, Yoon MH, Lim YH. Genotypic distribution of rotavirus strains causing severe gastroenteritis in Gyeonggi province, South Korea, from 2003 to 2005. Arch Virol. 2009; 154:167–170.


21. Jeong HS, Lee KB, Jeong AY, Jo MY, Jung SY, Ahn JH, et al. Genotypes of the circulating rotavirus strains in the seven prevaccine seasons from September 2000 to August 2007 in South Korea. Clin Microbiol Infect. 2011; 17:232–235.


22. Kang JO, Kilgore P, Kim JS, Nyambat B, Kim J, Suh HS, et al. Molecular epidemiological profile of rotavirus in South Korea, July 2002 through June 2003; emergence of G4P[6] and G9P [8] strains. J Infect Dis. 2005; 192:S57–S63.
23. Kim JS, Kang JO, Cho SC, Jang YT, Min SA, Park TH, et al. Epidemiological profile of rotavirus infection in the Republic of Korea results from prospective surveillance in the Jeongeub District, 1 July 2002 through 30 June 2004. J Infect Dis. 2005; 195:S49–S56.


24. Shim JO, Thai Than V, Ryoo E, Lim I, Yoon Y, Kim K, et al. Distribution of rotavirus G and P genotypes approximately two years following the introduction of rotavirus vaccines in South Korea. J Med Virol. 2013; 85:1307–1312.


25. Hull JJ, Teel EN, Kerin TK, Freeman MM, Esona MD, Gentsch JR, National Rotavirus, et al. United States rotavirus strain surveillance from 2005 to 2008: genotype prevalence before and after vaccine introduction. Pediatr Infect Dis J. 2011; 30:S42–S47.
26. Bok K, Matson DO, Gomez JA. Genetic variation of capsid protein VP7 in genotype g4 human rotavirus strains: simultaneous emergence and spread of different lineages in Argentina. J Clin Microbiol. 2002; 40:2016–2022.


27. Bucardo F, Karlsson B, Nordgren J, Paniaqua M, Gonzalez A, Amador JJ, et al. Mutated G4P[8] rotavirus associated with a nationwide outbreak of gastroenteritis in Nicargua in 2005. J Clin Microbiol. 2007; 45:990–997.


28. Matthijnssens J, Helen E, Zeller M, Rahman M, Lemey P, Van Ranst M. Phylodynamic analyses of rotavirus genotypes G9 and G12 underscore their potential for swift global spread. Mol Biol Evol. 2010; 27:2431–2436.


29. Esona MD, Banyai K, Foytich K, Freeman M, Mijatovic-Rustempasic S, Hull J, et al. Genomic characterization of human rotavirus G10 strains from the African Rotavirus Network: relationship to animal rotaviruses. Infect Genet Evol. 2011; 11:237–241.


30. Chitambar SD, Ranshing SS, Pradhan GN, Kalrao VR, Dhongde RK, Bavdekar AR. Changing trends in circulating rotavirus strains in Pune, western India in 2009-2012: Emergence of a rare G9P[4] rotavirus strain. Vaccine. 2014; 32:A29–A32.


31. Tatte VS, Chothe NS, Chitambar SD. Characterisation of rotavirus strains identified in adolescents and adults with acute gastroenteritis highlights circulation of non-typeable strains: 2008-2012. Vaccine. 2014; 32:Suppl 1. A68–A74.


32. Afrad MH, Rahman MZ, Matthijnssens , Das SK, Faruque AS, Azim T, et al. High incidence of reassortant G9P[4] rotavirus strain in Bangladesh: Fully heterotypic from vaccine strains. J Clin Virol. 2013; 58:755–756.


33. Yen C, Figueroa JR, Uribe ES, Carmen-Hernandez LD, Tate JE, Parashar UD, et al. Monovalent rotavirus vaccine provides protection against an emerging fully heterotypic G9P[4] rotavirus strain in Mexico. J Infect Dis. 2011; 204:783–786.

