Abstract
Background
Keloids are characterized by excessive collagen deposition in the dermis, in which transforming growth factor β (TGF-β)/Smad signaling plays an important role. Low-level light therapy (LLLT) is reported as effective in preventing keloids in clinical reports, recently. To date, studies investigating the effect of LLLT on keloid fibroblasts are extremely rare.
Objective
We investigated the effect of LLLT with blue (410 nm), red (630 nm), and infrared (830 nm) light on the collagen synthesis in keloid fibroblasts.
Methods
Keloid fibroblasts were isolated from keloid-revision surgery samples and irradiated using 410-, 630-, 830-nm light emitting diode twice, with a 24-hour interval at 10 J/cm2. After irradiation, cells were incubated for 24 and 48 hours and real-time quantitative reverse transcription polymerase chain reaction was performed. Western blot analysis was also performed in 48 hours after last irradiation. The genes and proteins of collagen type I, TGF-β1, Smad3, and Smad7 were analyzed.
Results
We observed no statistically significant change in the viability of keloid fibroblasts after irradiation. Collagen type I was the only gene whose expression significantly decreased after irradiation at 410 nm when compared to the non-irradiated control. Western blot analysis showed that LLLT at 410 nm lowered the protein levels of collagen type I compared to the control.
Keloids are characterized by hyperproliferative growth of dermal fibroblasts and excessive collagen deposition in the dermis12. Previous studies suggested that the initial step in the development of the fibrotic reaction in keloids involves the expression of transforming growth factor β1 (TGF-β1) in neovascular endothelial cells, thus inducing the adjacent fibroblasts to express markedly high levels of TGF-β1/β2 and their receptors, as well as type I and VI collagen34. Recent studies emphasize a potential role for TGF-β1 intracellular signaling pathways, especially for the Smad pathway, in the pathogenesis of keloids4. Smad2 and Smad3 are overexpressed and the inhibitory Smad6 and Smad7 are reduced in keloid fibroblasts135.
Treatments for keloids include surgical excision, intralesional corticosteroids, 5-fluorouracil, bleomycin and interferon, topical imiquimod, compression, cryotherapy, radiation, silicon sheeting and laser or light-based therapies, showing variable success. Recurrence is common, even with combination therapy6. Therefore, preventing keloid is an important issue.
Low-level light therapy (LLLT) uses low levels of visible to near infrared light. It has been widely used to reduce pain and inflammation, to promote wound healing, and to prevent tissue necrosis. Many reports demonstrate that LLLT modulates normal dermal fibroblasts and the TGF-β family1. Recently, two clinical reports showed that LLLT was effective in preventing hypertrophic scars and keloids78. However, studies investigating the effect of LLLT on keloid fibroblasts are still rare, especially the ones investigating the effects of LLLT at molecular level. Herein, we aimed to evaluate the effect of LLLT using blue (410 nm), red (630 nm), and infrared (830 nm) light on the collagen synthesis in keloid fibroblasts, by observing the signaling molecules involved.
Keloid fibroblasts were isolated from keloid-revision surgery samples after obtaining informed consents from the patients. Keloid fibroblasts were cultured in Dulbecco's modified Eagle's medium (DMEM), supplemented with 10% fetal bovine serum, 100 U/ml penicillin and 100 µg/ml streptomycin at 37℃ in a humidified atmosphere containing 5% carbon dioxide (CO2).
Keloid fibroblasts were seeded in a 6-well plate at 3.0×105 cells/well. APL® (Medro Medical Division, Seoul, Korea) was used for this study. This device produces blue (410 nm), red (630 nm), and infrared (830 nm) light emitting diode (LED) light. The power of each wavelength was 205 mW/cm2 for blue, 172 mW/cm2 for red, 50 mW/cm2 for infrared. Keloid fibroblasts were irradiated twice, with the second irradiation 24 hours after the first one. Preliminary experiment was performed to determine the lowest fluence to inhibit the proliferation of keloid fibroblasts. Cells were maintained in 400 µl Dulbecco's phosphate-buffered saline during irradiation and transferred in DMEM after irradiation for 24 or 48 hours.
To determine the direct effect of LLLT on the proliferation of viable cells, we counted the number of viable cells using the EZ-CyTox cell viability assay kit (Daeillab, Seoul, Korea). Twenty-four hours after the last irradiation, the cells were treated with 40 µl of Ez-CyTox solution and incubated for additional 3 hours at 37℃. Absorbance was measured at 450 nm using an ELISA reader (Molecular Device, Menlo Park, CA, USA). Viability was expressed as percentage of viable cells compared to the non-irradiated control. The experiment was repeated twice with two set of fibroblasts.
The mRNA of collagen type I, TGF-β1, Smad3, and Smad7 were measured in 24 and 48 hours after last irradiation. Total RNA was isolated using RNAasy® mini kit (Quiagen, Hilden, Germany). cDNA transcription was performed using the Superscript® III First-Strand (Invitrogen, Carlsbad, CA, USA) according to the manufacturer's instructions. cDNA was analyzed by real-time quantitative reverse transcription polymerase chain reaction using ABI Prism 7000 Sequence Detection System (Applied Biosystems, Foster, CA, USA). Fluorescent signals were collected during extension phase, Ct values of the sample were calculated, and the transcript levels were analyzed by 2−ΔΔCt method. Primers and internal probes for collagen type I, TGF-β1, Smad3, and Smad7 were purchased as assays on demand primer-probe sets (Applied Biosystems). The experiment was repeated five times.
The protein of collagen type I, TGF-β1, Smad3, and Smad7 were measured in 48 hours after last irradiation. Cells were lysed in lysis buffer (50 mM Tris [pH 7.4], 150 mM NaCl, 0.1% NP 40, 1% sodium dodecyl sulfate [SDS], 0.5% deoxycholic acid, 1 mM EDTA, protease and phosphatase inhibitors). Samples were separated on 10% SDS-polyacrylamide gels and transferred onto polyvinyldenefluoride membranes. The protein blots were incubated with antibodies against collagen type I (Abcam, Cambridge, MA, USA), TGF-β1 (GeneTex, Irvine, CA, USA), Smad3 (Epitomics, Burlingame, CA, USA), Smad7 (Santa Cruz Biotechnology, Santa Cruz, CA, USA) and actin (Santa Cruz Biotechnology). Detection was performed via enhanced chemiluminescence (Amersham Biosciences, Buckinghamshire, UK). The intensity of each band was quantified using Image J and the data were reported as relative intensity according to actin.
IBM SPSS Statistics Desktop ver. 20.0.0 (IBM Co., Armonk, NY, USA) was used for statistical analysis. Results are expressed as mean and standard deviation. Mann-Whitney U test was used for statistical evaluation between control and experimental groups in the study. A p-value lower than 0.05 was considered statistically significant.
Keloid fibroblasts were irradiated once with 3, 10, 30 J/cm2 fluences of the three wavelengths of 410-, 630-, and 830-nm as preliminary experiment. Tryptan blue assay exhibited proliferations rates of keloid fibroblasts tend to decrease only at 10 and 30 J/cm2, showing rather increased proliferation rate at 3 J/cm2, in all wavelengths, although it was not significant (Appendix). In the condition of similar proliferation rate, using lower dose is more suitable and convenient for conducting experiment. Therefore, we determined to irradiate 10 J/cm2 for keloid fibroblasts.
Viable keloid fibroblasts decreased with irradiation with all wavelengths compared to non-irradiated control. However, it was not statistically significant (Fig. 1).
Collagen type I expression was significantly decreased with irradiation at 410 nm in 48 hours (p<0.005). We also observed lower Smad3 expression after irradiation at 410 nm in 48 hours, but it was not statistically significant (p=0.065). There were no differences between irradiated samples in 24 hours and non-irradiated controls (Fig. 2, Table 1).
Irradiation at 410 nm reduced the protein levels of collagen type I in 48 hours. However, we observed no differences between irradiated samples and the control for TGF-β1, Smad3, and Smad7 (Fig. 3).
In the present study, we investigated whether LLLT could affect the collagen synthesis in keloid fibroblasts. Irradiation with 410 nm decreased collagen type I expression in keloid fibroblasts.
In normal fibroblasts, the effect of LLLT can be explained by photobiomodulation. Wavelengths above 500 nm are widely used to stimulate wound healing9. Their energy is absorbed by the cytochrome C oxidase in the mitochondrial membrane and activates various cellular signaling pathways through inducing the production of nitrogen oxide, adenosine triphosphate and reactive oxygen species (ROS)10. It can induce the transcription of various growth factors including platelet-derived growth factor, TGF-β, and fibroblast growth factor, and cytokines such as interleukins and tumor necrosis factor-alpha. On the other hand, blue light induces a higher production of intracellular ROS in a dose-dependent manner, which might act as a mediator of cellular effects of blue light11. Because of their relative close wavelengths, it is possible that blue and ultraviolet A light has similar biological effect such as ROS generation and immunomodulation11. Flavins are photo-acceptors and involved in the generation of ROS. Flavins are small, water-soluble molecules that absorb wavelengths below 500 nm and initiate free radical reactions when excited by light. Flavin-mediated photosensitization generates cytotoxic free radicals and is responsible for significant biological effects such as mutagenesis1213.
Previous reports indicate that 410 nm LED irradiation is cytotoxic for normal fibroblasts. Opländer et al.14 demonstrated that single irradiation with 410 or 420 nm LED at 5~10 J/cm2 inhibits the proliferation of dermal fibroblasts. Bonatti et al.15 also reported a significant reduction in the number of normal fibroblasts irradiated with 470 nm light at 18 J/cm2. According to Seo et al.16, red (630 nm, 9.5 J/cm2) and green (530 nm, 9.8 J/cm2) LED-irradiated cells were significantly more proliferated than cells irradiated with blue light (460 nm, 27 J/cm2). Also, blue light did not increase the expression of collagen type I and TGF-β1 in the dermis compared to the control, whereas red and green light enhance the expression of both genes.
In contrast to studies on normal fibroblasts, reports on the effect of LLLT on keloid fibroblasts are extremely rare and disappointing. Bonatti et al.15 showed that a single irradiation with 470 nm LED at 6, 12, and 18 J/cm2 does not induce significant differences in the number of keloid fibroblasts. To our knowledge, no study reports that LLLT affects the molecules investigated here in keloid fibroblasts.
Our results showed that collagen mRNA levels decreased after irradiation in keloid fibroblasts, which means that LLLT inhibited the transcription of this gene. Unfortunately, it is uncertain whether the TGF-β/Smad signaling pathway is inhibited in keloid fibroblasts, because other downstream signaling components, except collagen type I, did not show any significant changes in their expression after LLLT. It should be considered that LLLT also affects other signaling pathways in keloid, such as the mitogen-activated protein kinase (MAPK), which is suggested to mediate TGF-β/Smad signal transduction1718. The influence of MAPK on keloid fibroblasts was evaluated after pulsed dye laser (PDL) treatment in a few reports. Activation of extracellular signal-regulated kinase and the p38 signal transduction pathway is involved in the suppression of keloid fibroblast proliferation and the induction of apoptosis after PDL treatment1920. Recently, possible role of wingless type (Wnt)/β-catenin signaling pathway in keloid pathogenesis has been reported, which was known to play a key role in cellular proliferation. Wnt5a and β-catenin, its effector, were highly expressed in keloid fibroblasts and mediate the effect of TGF-β in wound healing process21. In addition, unlike normal fibroblasts, abnormal TGF-β signaling and autocrine loop are important in keloid fibroblasts14. For example, keloid fibroblasts show marked sensitivity to TGF-β, causing an accelerated increase in collagen and fibronectin synthesis compared to normal fibroblast2223. Therefore, the peak time of the TGF-β/Smad signaling components in keloid fibroblasts might be different from that of normal fibroblasts and it might be difficult to predict their expression pattern14.
This study has significance, in that it demonstrated that LLLT with 410 nm light inhibited collagen synthesis in keloid fibroblasts in vitro. Considering the initial pathophysiology of keloid, in which the expression of the TGF-β1 activates the adjacent fibroblasts to produce collagen, LLLT might be effective in preventing keloid formation at the initial stage. Corresponding clinical studies with blue LED and further investigative studies are needed to demonstrate the signaling mechanisms in keloid fibroblasts after LLLT. We hope this study can be a starting point for clinical application of LLLT to patients with keloid scars.
Figures and Tables
Fig. 1
Viable keloid fibroblasts had tendency to decrease after irradiation with all wavelengths compared to non-irradiated keloid control. However, there was no statistically significant decrease compared to the control. The results are represented mean±standard error from two independent experiments with two set of fibroblasts.
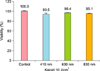
Fig. 2
Only expression of collagen type I showed a significant decrease in cell irradiate with 410 nm light compared to non-irradiated control after 48 hours. The results are represented as mean±standard error from five independent experiments. TGF-β1: transforming growth factor β1. *p<0.05 vs. non-irradiated control.
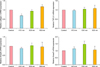
Fig. 3
Low-level light therapy suppressed the expression of collagen type I in keloid fibroblasts irradiated with 410 nm light. TGF-β1: transforming growth factor β1.
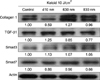
Table 1
Relative mRNA level of collagen type I, TGF-β1, Smad3, and Smad7 after LLLT at 10 J/cm2 in keloid fibroblasts

ACKNOWLEDGMENT
This study was supported by Basic Science Research Program through the National Research Foundation of Korea (NRK) (Grant no. 2013R1A1A2006944). This work was supported by the GRRC program of Gyeonggi province (GRRC AJOU 2016B04, Photonics-Medical Convertgence Technology Research Center).
References
1. Tsujita-Kyutoku M, Uehara N, Matsuoka Y, Kyutoku S, Ogawa Y, Tsubura A. Comparison of transforming growth factor-beta/Smad signaling between normal dermal fibroblasts and fibroblasts derived from central and peripheral areas of keloid lesions. In Vivo. 2005; 19:959–963.
2. Park SY, Park JY, Kim CH, Kang SU, Kim JH, Bark KM, et al. Effects of Xanthium stramarium and Psoralea corylifolia extracts combined with UVA1 irradiation on the cell proliferation and TGF-β1 expression of keloid fibroblasts. Ann Dermatol. 2013; 25:304–309.


3. Chin GS, Liu W, Peled Z, Lee TY, Steinbrech DS, Hsu M, et al. Differential expression of transforming growth factor-beta receptors I and II and activation of Smad 3 in keloid fibroblasts. Plast Reconstr Surg. 2001; 108:423–429.


4. Jagadeesan J, Bayat A. Transforming growth factor beta (TGFbeta) and keloid disease. Int J Surg. 2007; 5:278–285.
5. Phan TT, Lim IJ, Aalami O, Lorget F, Khoo A, Tan EK, et al. Smad3 signalling plays an important role in keloid pathogenesis via epithelial-mesenchymal interactions. J Pathol. 2005; 207:232–242.


6. Mamalis AD, Lev-Tov H, Nguyen DH, Jagdeo JR. Laser and light-based treatment of Keloids--a review. J Eur Acad Dermatol Venereol. 2014; 28:689–699.
7. Barolet D, Boucher A. Prophylactic low-level light therapy for the treatment of hypertrophic scars and keloids: a case series. Lasers Surg Med. 2010; 42:597–601.


8. Carvalho RL, Alcântara PS, Kamamoto F, Cressoni MD, Casarotto RA. Effects of low-level laser therapy on pain and scar formation after inguinal herniation surgery: a randomized controlled single-blind study. Photomed Laser Surg. 2010; 28:417–422.


9. da Silva JP, da Silva MA, Almeida AP, Lombardi Junior I, Matos AP. Laser therapy in the tissue repair process: a literature review. Photomed Laser Surg. 2010; 28:17–21.


10. Hamblin MR, Demidova T. Mechanisms of low level light therapy. Proc of SPIE. 2006; 6140:614001–614012.


11. Mamalis A, Garcha M, Jagdeo J. Light emitting diode-generated blue light modulates fibrosis characteristics: fibroblast proliferation, migration speed, and reactive oxygen species generation. Lasers Surg Med. 2015; 47:210–215.


12. Gorgidze LA, Oshemkova SA, Vorobjev IA. Blue light inhibits mitosis in tissue culture cells. Biosci Rep. 1998; 18:215–224.


13. Eichler M, Lavi R, Shainberg A, Lubart R. Flavins are source of visible-light-induced free radical formation in cells. Lasers Surg Med. 2005; 37:314–319.


14. Opländer C, Hidding S, Werners FB, Born M, Pallua N, Suschek CV. Effects of blue light irradiation on human dermal fibroblasts. J Photochem Photobiol B. 2011; 103:118–125.


15. Bonatti S, Hochman B, Tucci-Viegas VM, Furtado F, Pinfildi CE, Pedro AC, et al. In vitro effect of 470 nm LED (Light Emitting Diode) in keloid fibroblasts. Acta Cir Bras. 2011; 26:25–30.


16. Seo YK, Park JK, Song C, Kwon SY. Comparison of light-emitting diode wavelength on activity and migration of rabbit ACL cells. Lasers Med Sci. 2014; 29:245–255.


17. He S, Liu X, Yang Y, Huang W, Xu S, Yang S, et al. Mechanisms of transforming growth factor beta(1)/Smad signalling mediated by mitogen-activated protein kinase pathways in keloid fibroblasts. Br J Dermatol. 2010; 162:538–546.


18. Lim IJ, Phan TT, Tan EK, Nguyen TT, Tran E, Longaker MT, et al. Synchronous activation of ERK and phosphatidylinositol 3-kinase pathways is required for collagen and extracellular matrix production in keloids. J Biol Chem. 2003; 278:40851–40858.


19. Kuo YR, Wu WS, Jeng SF, Huang HC, Yang KD, Sacks JM, et al. Activation of ERK and p38 kinase mediated keloid fibroblast apoptosis after flashlamp pulsed-dye laser treatment. Lasers Surg Med. 2005; 36:31–37.


20. Kuo YR, Wu WS, Wang FS. Flashlamp pulsed-dye laser suppressed TGF-beta1 expression and proliferation in cultured keloid fibroblasts is mediated by MAPK pathway. Lasers Surg Med. 2007; 39:358–364.


21. Igota S, Tosa M, Murakami M, Egawa S, Shimizu H, Hyakusoku H, et al. Identification and characterization of Wnt signaling pathway in keloid pathogenesis. Int J Med Sci. 2013; 10:344–354.


22. Babu M, Diegelmann R, Oliver N. Keloid fibroblasts exhibit an altered response to TGF-beta. J Invest Dermatol. 1992; 99:650–655.
23. Younai S, Nichter LS, Wellisz T, Reinisch J, Nimni ME, Tuan TL. Modulation of collagen synthesis by transforming growth factor-beta in keloid and hypertrophic scar fibroblasts. Ann Plast Surg. 1994; 33:148–151.