Abstract
Purpose
Recent evidence suggests that B cells can both promote and inhibit the development and progression of allergic disease. However, the characteristics of B cell subsets in patients with allergic rhinitis (AR) have not been well documented. This study aimed to analyze the characteristics of B cell subsets in the peripheral blood of AR patients.
Methods
Forty-seven AR patients and 54 healthy controls were enrolled in this study, and the B cell subsets in peripheral blood of all subjects were analyzed by flow cytometry. Moreover, the serum total immunoglobulin E (IgE) and IgE concentrations secreted into the cultured peripheral blood mononuclear cells (PBMCs) were measured by using enzyme-linked immunosorbent assay.
Results
We found the peripheral blood of AR patients contained higher percentages of memory B cells, plasma cells, and CD19+CD24hiCD27+ regulatory B cells (Bregs) than those of age-matched healthy controls (P<0.05), while the percentages of naïve B cells and CD19+CD24hiCD38hi Bregs were significantly lower in AR patients than in healthy individuals (P<0.05). In addition, the serum total IgE and IgE concentrations secreted into the cultured PBMCs were elevated in AR patients than in the healthy controls (P<0.05).
Allergic rhinitis (AR), a common allergic disorder of the airway, affects 10% to 40% of the population, and its increasing prevalence has made it a global health problem worldwide.1 AR is characterized by nasal itching, rhinorrhea, sneezing, and nasal congestion due to persistent local inflammation of nasal mucosa in the upper airway. It is well known that AR frequently precedes bronchial asthma, and the presence of AR is considered a risk factor for new onset of asthma.23 Compared to other medical conditions, AR might not appear to be serious because it is not associated with severe morbidity and mortality. However, the burden and costs are substantial.34
Allergic airway disorders are caused by an immunological defect based on type I hypersensitivity reactions through activation of eosinophils, basophils, and mast cells as well as increased antigen-specific immunoglobulin E (IgE).5 The clinical condition of type I hypersensitivity is attributable to a functional imbalance of type 1 and type 2 helper CD4+ T cells (Th1 and Th2 cells, respectively) caused by epithelial cell-derived cytokines, including thymic stromal lymphopoietin, interleukin (IL)-25, and IL-33. Under this condition, Th2 cells secrete IL-4, IL-5, and IL-13 to promote unfavorable immune responses by helping B cells produce allergen-specific IgE in the airway.6
The potential involvement of different B cell subsets in the pathogenesis and specific immunotherapy of AR has not been well documented.78 Celiksoy et al.9 showed that relative and absolute counts of active and mature sensitive B cells were higher in AR patients than in the healthy children. Kamekura et al.10 demonstrated that the ratio of regulatory B cell (Breg) cells to total B cells was decreased in AR cases and, more extensively, in concomitant AR and asthma cases. Since several B cell subsets, such as naïve B cells, memory B cells, plasma cells, and Bregs, were suggested to be the main subsets in peripheral blood, but the characteristics of these B cell subsets in AR patients have not been well documented. To address this issue, this study aimed to analyze the characteristics of these B cell subsets in peripheral blood of AR patients and healthy controls.
All patients were diagnosed according to the Allergic Rhinitis and its Impact on Asthma (ARIA) guideline.3 A total of 47 patients with AR were recruited from February 2017 to July 2017 in the outpatient service of the Department of Otorhinolaryngology at the Zhongnan Hospital of Wuhan University, China. In addition, 54 age- and gender-matched healthy controls who had not taken any medications for at least 4 weeks before sample collection were also recruited during the same period. These sample sizes were sufficient to detect differences based on our preliminary experiments and other published papers. Written informed consent forms were obtained from all participants or relatives of these participants, and the study was approved by the Medical Ethics Committee of the Zhongnan Hospital of Wuhan University, China. All the patients had not been treated with immunosuppressive agents, such as inhaled corticosteroids or combination therapy, during a period of 4 weeks before the enrollment.
The subjects underwent both blood tests and skin prick tests (SPTs). The blood tests included serum total IgE levels and serum-specific IgE for allergens (such as house dust mite [HDM], tree and grass pollen, cat, dog, and food). Serum allergen-specific IgE levels below 0.35 IU/mL were classified as undetectable. SPTs were conducted on the patient's left forearm using commercial allergen extracts (Bencard, Brentford, UK). Histamine hydrochloride (1 mg/mL; Bencard) was used as the positive control, and physiological saline was used as the negative control. The reactions were assessed after 15 minutes and classified as negative (0, no reaction; +, a reaction greater than the control reaction, but less than half that caused by histamine) or positive (++, half the level caused by histamine; +++, equal to the level caused by histamine; and ++++, twice the level caused by histamine). Inclusion criteria for patients with AR were as follows: a positive SPT for at least HDM (wheal >5 mm), total IgE ≥30 IU/mL, and detectable specific IgE for at least HDM (>0.35 IU/mL).
Fasting venous blood samples from AR patients and healthy controls were collected between 8:00 and 9:00 a.m. Half of each blood sample was centrifuged to prepare serum samples, and the remainder was used to prepare peripheral blood mononuclear cells (PBMCs). All blood samples were processed within 6 hours after collection.
PBMCs were isolated from the blood samples of AR patients and healthy controls as previously described.11 In brief, heparinized whole blood samples were overlaid onto Ficoll separation media (Tianjin Haoyang Biological Manufacture, Tianjin, China) after 1:1 dilution with Hank's Balanced Salted Solution (Gibco, ThermoFisher Scientific, Waltham, CA, USA). PBMCs were density-gradient centrifuged for 30 minutes at ×1,800 rpm, collected at the plasma interface, and washed 3 times after centrifugation at ×2,000 rpm for 3 minutes. Then, the PBMCs were resuspended in RPMI-1640 medium (HyClone, Logan, UT, USA) and counted, and the final concentration prior to flow cytometry analysis and cell culture was 1×106 cells/mL.
The purified PBMCs were resuspended in a complete RPMI-1640 medium (HyClone) and then incubated on the 96-well plate at a concentration of 1×106 cells/well. The cells were washed once with phosphate-buffered saline (PBS), and fixed and permeabilized using a fixation/permeabilisation kit (BD Biosciences, San Diego, CA, USA). For surface staining, after fixation and permeabilization, the cells were stained with the antibody cocktail: anti-CD19 PerCP/Cy5.5, anti-CD24 PE, anti-CD27 PE/Cy7, and anti-CD38 APC; Biolegend, San Diego, CA, USA. The cells were incubated for 1 hour in the dark and washed twice with 200 µL of PBS containing 0.5% bovine serum albumin. After discarding the supernatant, cells were resuspended in 300 µL of PBS for flow cytometry. Samples were detected by flow cytometry on a FACS Verse flow cytometer (BD Biosciences), which was followed by analysis with FlowJo 10.1 software (Tree Star, San Carlos, CA, USA). At least 50,000 events per sample were analyzed. Cells stained with isotype control antibody served as the negative control. Compensation was made according to single staining. The cells were gated initially for living lymphocytes as CD19+ using forward and side scatter histogram. The expression of CD19+CD24hiCD38hi B cells, CD19+CD24hiCD27+ B cells, CD19+CD27+ B cells, CD19+CD27− B cells, and CD19+CD38+CD24− plasma cells were detected separately.
The isolated PBMCs were resuspended at 1×106 cells/mL and cultured in RPMI-1640 medium (HyClone), supplemented with 10% heat-inactivated fetal bovine serum (FBS; HyClone), 100 U/mL penicillin, and 100 µg/mL streptomycin, a 1-mL aliquot of the suspendion was dispensed into 6-well flat-bottom plates with RPMI-1640 (1×106 cells/well) and incubated at 37℃ in a 5% CO2 environment using humidified incubators for 3, 5, and 7 days.
Serum IgE levels and IgE concentrations secreted into the supernatants in the AR patients and the healthy controls were determined using a commercially available human ELISA kit (Shenzhen Dakewe Biological Manufacture, Shenzhen, Guangdong, China). Assays were performed strictly following the manufacturer's instructions, using an automated ELISA reader. All samples were measured in duplicate. Optical density values were evaluated at 450 nm, and IgE concentrations were calculated from standard curves.
Statistical analyses were performed with GraphPad Prism version 6.0 (GraphPad Software, San Diego, CA, USA). Data are described as the mean±standard error of mean. The percentage of CD19+ B cells, CD19+CD27+ (CD27−) B cells, CD19+CD38+CD24− plasma cells, CD19+CD24hiCD28hi, and CD19+CD24hiCD27+ B cells, as well as IgE level of each patient and health control, was analyzed using the unpaired 2-tailed Student's t test. The non-parametric Mann-Whitney U test was used to determine the significance of differences between the patient and control groups, unless stated otherwise. Correlations between the Bregs subsets and serum total IgE levels were evaluated using Spearman's coefficient of correlation. For all analyses, P values <0.05 were considered statistically significant.
Forty-seven AR patients were selected according to the diagnostic criteria of the ARIA guideline. The clinical characteristics of the patients are summarized in Table 1. We assessed the composition of the B cell populations in PBMCs of 47 AR patients and compared it to 54 age- and sex-matched healthy controls.
Gating strategy for the delineation of the 5 major circulating B cell subsets in the PBMCs characterized in this study are shown in Fig. 1. Fluorochrome-conjugated surface markers (CD19, CD24, CD27, and CD38) used for the surface characterization of the subsets are shown in the respective plot. The initial CD19+ gate (CD19 vs side-scatter), was derived from a lymphocyte gate (defined on forward- and side-scatter) followed by single-cell discrimination. Plots and frequencies shown in these plots correspond to the delineation of the subsets in 1 illustrative individual among gated CD19+ cells. Numbers represent the percentage of cells within the gate.
The percentages of CD19+ B cells among lymphocytes and PBMCs in the peripheral blood were significantly increased in the peripheral blood in the AR patients than in the healthy controls (20.08% vs 11.05%, P<0.001; and 6.119% vs 1.922%, P<0.0001, respectively) (Fig. 2A and B). Meanwhile, the composition of the B cell subsets showed large differences. The percentage of memory B cells (defined as CD19+CD27+) in lymphocytes was higher in the AR patients than in the healthy controls (28.92% vs 19.61%, P=0.004; Fig. 2C), suggesting an impact of AR on B cell homeostasis. Conversely, PBMCs contained more virgin naïve (CD19+CD27−) B cells (70.97% vs 80.32%, P=0.0037; Fig. 2D).
A regulatory function has been described for transitional B cells (CD19+CD24hiCD38hi, Fig. 3). We measured the percentages and numbers of this subset of Bregs in the AR patients and the healthy controls. Although the total Bregs the peripheral blood were similar between the AR patients and the healthy controls (0.3874%±0.07426% vs 0.4465%±0.06302%, P=0.5428; and 0.1405%±0.04167% vs 0.06751%±0.009684%, P=0.0672, respectively) (Fig. 3A and B), significantly lower percentage of CD19+CD24hiCD38hi Bregs on CD19+ B cells were observed in the AR patients compared to the healthy controls (1.729%±0.244% vs 4.160%±0.5497%, P=0.0002) in the B cell subsets of this Bregs phenotype as shown in Fig. 3C. The CD19+CD24hiCD27+ B cell population has recently been described as another Breg subset.16 This population was regularly detected within lymphocytes and PBMCs from the AR patients and was significantly elevated compared to those from the healthy controls (3.795% vs 0.8118%, P<0.0001; and 1.263% vs 0.1352%, P=0.0002, respectively) (Fig. 3D and E). The percentage of CD19+CD24hiCD27+ Bregs on CD19+ B cells was elevated in the AR patients compared to the healthy controls (18.25%±2.848% vs 7.659%±0.6826%, P=0.0002) as shown in Fig. 3F.
The percentage of plasma cells was elevated in PBMCs from the AR patients compared to the healthy controls (2.085% vs 1.224%, P=0.0151; Fig. 4A). Serum IgE levels and IgE concentrations secreted into the supernatants were measured by ELISA. The serum total IgE level of the AR patients was significantly higher than the healthy controls (137.2±11.66 IU/mL vs 59.33±9.829 IU/mL, P<0.001). IgE concentrations secreted into the supernatants at 3, 5, and 7 days in the AR patients were also elevated compared to the healthy controls, especially at 5 days (P=0.0051).
Finally, we analyzed the relationship of different Bregs subsets with IgE levels. Our results showed that there were no significant correlations between IgE levels and CD19+CD24hiCD38hi Bregs/CD19+CD24hiCD27+ Bregs (P>0.05) as shown in Fig. 5.
In the present study, we characterized the phenotypes of B cell subsets in the peripheral blood from the AR patients and the healthy controls by flow cytometry. Our results demonstrated that the percentages and numbers of memory B cells, plasma cells and CD19+CD24hiCD27+ Bregs were significantly increased in AR patients compared to the healthy individuals. However, significant declines in naïve B cells and CD19+CD24hiCD38hi Bregs were observed in the AR patients.
CD19 is a B cell-specific cell-surface molecule and a member of the immunoglobulin superfamily. It is only expressed on B cells from the earliest stages of pre-B cell development to B cell terminal differentiation into plasma cells or follicular dendritic cells. It also plays a critical role in B cell activation and autoimmunity. CD19 is a signaling molecule known to transduce signals initiated through the B cell receptor and positively regulates B cell responses.12 Memory B cells are generated in germinal centers and contribute to serological immunity by rapidly differentiating into high-affinity plasma cells following a re-encounter with the immunizing antigen. Currently, human memory B cells can be identified by the expression of CD27, although some memory B cells may be CD27 negative.13 B lymphocytes shape the humoral immunity and are classically considered to amplify the immune response because of their capability to produce antibodies (including autoantibodies) as well as act as antigen-presenting cells (APCs) to modulate T cell-mediated immune responses. In this study, our findings show significantly increased percentages and numbers of memory B cells and plasma cells from the AR patients compared to the healthy individuals, which was consistent with those of a previous study.9
Interestingly, we also found significant decreases in CD19+CD24hiCD38hi Bregs, but not CD19+CD24hiCD27+ Bregs, in AR patients. It has generally been accepted that several B cell subsets are immunoregulatory cells that protect against chronic inflammatory condition from excessive immune response,14 which are generally referred to as Bregs. Bregs mediate protective immune responses via the production of regulatory cytokines, such as IL-10, transforming growth factor-β, and IL-35, and express inhibitory molecules to suppress pathogenic T cells and autoreactive B cells in a cell-to-cell contact-dependent manner.15 The absence or loss of Bregs may exacerbate symptoms in autoimmune and chronic inflammatory diseases as well as promote tumor progression.16 Recent investigations have revealed that the levels of IgE can be regulated by Bregs, which produce the negative regulatory cytokine IL-10, and that Breg cells may be increased in AR patients in response to allergen-specific immunotherapy.17 These findings provide evidence that Bregs is considered a therapeutic indicator of clinical efficacy for AR patients with allergen-specific immunotherapy. Various Breg subsets in humans, characterized by the expression of CD19+CD24hiCD38hi or by CD19+CD24hiCD27+ phenotypes, have been described and shown a reduced function in certain autoimmune diseases, such as systemic lupus erythematosus.18 However, our study showed that CD19+CD24hiCD38hi Bregs, but not CD19+CD24hiCD27+ Bregs, were significant decreased in the AR patients, indicating that CD19+CD24hiCD38hi Bregs may solely be responsible for the inhibition of AR development during allergen-specific immunotherapy.78 Sumimoto et al.19 demonstrated that CD19+CD24hiCD38hi Bregs may be reactively increased like ICOS+ Tregs to suppress disease activity. Iwata et al.20 demonstrated that the regulatory capacity of CD19+CD24hiCD27+ B cells reduces cytokine production by monocytes. Interestingly, this reduction was not IL-10-dependent and these cells could not reduce cytokine production by T cells. Furthermore, CD19+CD24hiCD27+ B cells may represent a distinct activated memory B cell subset that shares common cell surface markers with memory B cells, but not equivalent to memory B cells,21 and are more mature than transitional CD19+CD24hiCD38hi Bregs; thus, they are more likely to differentiate into antibody-producing cells that no longer possess a regulatory function.22 Therefore, the increased percentage of CD19+CD24hiCD27+ Bregs in AR indicated that this Bregs subset might contribute to the development of AR. Further studies addressing B cells data from nasal mucosa or nasal lavage fluids are needed to directly reflect the immunologic events in AR.
In summary, our results showed significantly increased percentages and numbers of memory B cells, plasma cells, as well as CD19+CD24hiCD27+ Bregs, in the AR patients compared to the healthy individuals, and significant declines in naïve B cells, as well as CD19+CD24hiCD38hi Bregs, in the AR patients. These results provide indirect evidence that CD19+CD24hiCD38hi Bregs, but not CD19+CD24hiCD27+ Bregs, may be considered a therapeutic indicator of clinical efficacy for AR patients with allergenspecific immunotherapy. Further research may be necessary to identify pathways and molecules that enhance the number and/or activity of CD19+CD24hiCD38hi Bregs to improve the therapeutic efficacy for AR patients during allergen-specific immunotherapy.
Figures and Tables
![]() | Fig. 1Distribution of major circulating B cell subsets in the AR patients and the healthy controls. (A) FSC measures cell size, and SSC measures cell granularity. After lymphocyte gate, the CD19+ (B cell) population gates of the healthy controls and the AR patients are depicted. (B) Memory B cells (CD19+CD27+) and naïve B cells (CD19+CD27−) of the healthy controls and the AR patients. (C) Bregs (CD19+CD24hiCD28hi) and plasma cells (CD19+CD38+CD24−) of the healthy controls and AR patients. (D) Bregs (CD19+CD24hiCD27+) of the healthy controls and the AR patients. AR, allergic rhinitis; FSC, forward scatter; SSC, side scatter; Breg, regulatory B cell. |
![]() | Fig. 2B cells and B cell subsets in lymphocytes and PBMCs of the AR patients and the healthy controls. (A) The percentage of CD19+ B cells in lymphocytes is significantly higher in the AR patients than in the healthy controls (20.08% vs 11.05%, P<0.0001, for lymphocytes). (B) The percentage of CD19+ B cells in PBMCs is significantly higher in the AR patients than in the healthy controls (6.119% vs 1.922%, P<0.0001, for PBMCs). (C) The PBMCs contain a significantly higher percentage of memory (CD19+CD27+) B cells in the AR patients than in the healthy controls (28.92% vs 19.61%, P=0.0040). (D) With increasing memory B cells, virgin naïve (CD19+CD27−) B cells are decreased in PBMCs from the AR patients compared to the healthy controls (70.97% vs 80.32%, P=0.0037). PBMC, peripheral blood mononuclear cell; AR, allergic rhinitis; ns, no significance. *P<0.05; †P<0.01; ‡P<0.001; §P<0.0001. |
![]() | Fig. 3Regulatory B cell subsets in the AR patients. (A–C) The percentages of CD19+CD24hiCD38hi (transitional) B cells in lymphocyte, PBMCs, and CD19+ B cells in the AR patients compared to the healthy controls (0.3873% vs 0.4465%, P=0.5428 for lymphocytes; 0.1405% vs 0.06751% P=0.0672 for PBMCs, 18.25% vs 7.659% for CD19+ B cells). (D–F) The percentages of CD19+CD24hiCD27+ (transitional) B cells in lymphocyte, PBMCs, and CD19+ B cells in the AR patients compared to the healthy controls. CD19+CD24hiCD27+ B cells accounted for higher percentages of B cells in lymphocytes and PBMCs and had significantly larger numbers of lymphocytes and PBMCs in the AR patients compared to the healthy controls (3.795% vs 0.8118%, P<0.0001 for lymphocytes; 1.263% vs 0.1352% P=0.0002 for PBMCs; 18.25% vs 7.659%, P=0.0002 for CD19+ B cells). AR, allergic rhinitis; PBMC, peripheral blood mononuclear cell; ns, no significance. *P<0.05; †P<0.01; ‡P<0.001; §P<0.0001. |
![]() | Fig. 4Levels of serum total IgE and IgE concentrations secreted into supernatants of the PBMCs cultured for 3, 5, and 7 days the AR patients and the healthy controls. (A) The percentage of plasma cells was elevated in PBMCs in the AR patients compared to the healthy controls (P=0.0151). (B) Levels of serum total IgE. (C, D) Levels of IgE concentrations secreted into the supernatants of the cultured PBMCs. IgE, immunoglobulin E; PBMC, peripheral blood mononuclear cell; AR, allergic rhinitis; ns, no significance. *P<0.05; †P<0.01; ‡P<0.001; §P<0.0001. |
![]() | Fig. 5(A) Correlation between CD19+CD24hiCD38hi Breg percentage and serum IgE level. (B) Correlation between CD19+CD24hiCD27+ Breg percentage and serum IgE level. Breg, regulatory B cell; IgE, immunoglobulin E. |
Table 1
Clinical characteristics of the AR patients and the healthy controls
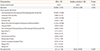
ACKOWLEDGMENTS
This study was supported by the grants from National Natural Science of China (No. 81470673, 81725004, 81600788, 81500781) and Shanghai Science and Technology Committee (No. 16410723600).
References
1. Greiner AN, Hellings PW, Rotiroti G, Scadding GK. Allergic rhinitis. Lancet. 2011; 378:2112–2122.


2. Shaaban R, Zureik M, Soussan D, Neukirch C, Heinrich J, Sunyer J, et al. Rhinitis and onset of asthma: a longitudinal population-based study. Lancet. 2008; 372:1049–1057.


3. Bousquet J, Khaltaev N, Cruz AA, Denburg J, Fokkens WJ, Togias A, et al. Allergic Rhinitis and its Impact on Asthma (ARIA) 2008 update (in collaboration with the World Health Organization, GA(2) LEN and AllerGen). Allergy. 2008; 63:Suppl 86. 8–160.
4. Yoo KH, Ahn HR, Park JK, Kim JW, Nam GH, Hong SK, et al. Burden of respiratory disease in Korea: an observational study on allergic rhinitis, asthma, COPD, and rhinosinusitis. Allergy Asthma Immunol Res. 2016; 8:527–534.


5. Wu LC, Zarrin AA. The production and regulation of IgE by the immune system. Nat Rev Immunol. 2014; 14:247–259.


7. Jutel M, Agache I, Bonini S, Burks AW, Calderon M, Canonica W, et al. International Consensus on Allergen Immunotherapy II: mechanisms, standardization, and pharmacoeconomics. J Allergy Clin Immunol. 2016; 137:358–368.


8. Rhee CS. Current specific immunotherapy for allergic rhinitis: perspectives from otorhinolaryngologists. Allergy Asthma Immunol Res. 2014; 6:273–275.


9. Celiksoy MH, Sancak R, Yildiran A. The role of active B cells in allergen immunotherapy. Allergol Immunopathol (Madr). 2017; 45:439–444.


10. Kamekura R, Shigehara K, Miyajima S, Jitsukawa S, Kawata K, Yamashita K, et al. Alteration of circulating type 2 follicular helper T cells and regulatory B cells underlies the comorbid association of allergic rhinitis with bronchial asthma. Clin Immunol. 2015; 158:204–211.


11. Xiao L, Wei Y, Zhang YN, Luo X, Yang BY, Yu SF, et al. Increased IL-21 expression in chronic rhinosinusitis with nasal polyps. Clin Exp Allergy. 2015; 45:404–413.
12. Noh J, Noh G. Allergen-specific responses of CD19(high) and CD19 (low) B cells in Non-IgE-mediated food allergy of late eczematous reactions in atopic dermatitis: presence of IL-17- and IL-32-producing regulatory B cells (Br17 & Br32). Inflamm Allergy Drug Targets. 2012; 11:320–329.
13. Klein U, Rajewsky K, Kuppers R. Human immunoglobulin (Ig)M+ IgD+ peripheral blood B cells expressing the CD27 cell surface antigen carry somatically mutated variable region genes: CD27 as a general marker for somatically mutated (memory) B cells. J Exp Med. 1998; 188:1679–1689.
14. Lee KM, Stott RT, Zhao G, SooHoo J, Xiong W, Lian MM, et al. TGF-β-producing regulatory B cells induce regulatory T cells and promote transplantation tolerance. Eur J Immunol. 2014; 44:1728–1736.


16. Mauri C, Menon M. Human regulatory B cells in health and disease: therapeutic potential. J Clin Invest. 2017; 127:772–779.


17. Palomares O, Akdis M, Martín-Fontecha M, Akdis CA. Mechanisms of immune regulation in allergic diseases: the role of regulatory T and B cells. Immunol Rev. 2017; 278:219–236.


18. Blair PA, Norena LY, Flores-Borja F, Rawlings DJ, Isenberg DA, Ehrenstein MR, et al. CD19(+)CD24(hi)CD38(hi) B cells exhibit regulatory capacity in healthy individuals but are functionally impaired in systemic Lupus Erythematosus patients. Immunity. 2010; 32:129–140.


19. Sumimoto K, Uchida K, Kusuda T, Mitsuyama T, Sakaguchi Y, Fukui T, et al. The role of CD19+ CD24high CD38high and CD19+ CD24high CD27+ regulatory B cells in patients with type 1 autoimmune pancreatitis. Pancreatology. 2014; 14:193–200.
20. Iwata Y, Matsushita T, Horikawa M, Dilillo DJ, Yanaba K, Venturi GM, et al. Characterization of a rare IL-10-competent B-cell subset in humans that parallels mouse regulatory B10 cells. Blood. 2011; 117:530–541.


21. Jin L, Weiqian C, Lihuan Y. Peripheral CD24hi CD27+ CD19+ B cells subset as a potential biomarker in naive systemic lupus erythematosus. Int J Rheum Dis. 2013; 16:698–708.