Abstract
Purpose
Bisphenol A (BPA) exposure may increase the risk of asthma. Genetic polymorphisms of oxidative stress-related genes, glutathione S-transferases (GSTM1, GSTP1), manganese superoxide dismutase, catalase, myeloperoxidase, and microsomal epoxide hydrolase may be related to BPA exposure. The aim is to evaluate whether oxidative stress genes modulates associations of BPA exposure with asthma.
Methods
We conducted a case-control study comprised of 126 asthmatic children and 327 controls. Urine Bisphenol A glucuronide (BPAG) levels were measured by ultra-performance liquid chromatography/tandem mass spectrometry, and genetic variants were analyzed by a TaqMan assay. Information on asthma and environmental exposure was collected. Analyses of variance and logistic regressions were performed to determine the association of genotypes and urine BPAG levels with asthma.
Results
BPAG levels were significantly associated with asthma (adjusted odds ratio [aOR], 1.29 per log unit increase in concentration; 95% confidence interval [CI], 1.081.55). Compared to the GG genotype, children with a GSTP1 AA genotype had higher urine BPAG concentrations (geometric mean [standard error], 12.72 [4.16] vs 11.42 [2.82]; P=0.036). In children with high BPAG, the GSTP1 AA genotype was related to a higher odds of asthma than the GG genotype (aOR, 4.84; 95% CI, 1.0223.06).
Bisphenol A (BPA) is a xenoestrogen and found in toys, drinking containers, dental sealants, water pipes, and food containers, including those with infant formula.123456 The primary route of exposure to BPA is through oral intake, but exposure can also occur via dermal contact or through inhalation.3 BPA has been detected at higher concentrations in children than in adults, a finding that has been explained by children's greater food intake in proportion to body weight or variances in metabolism.78 Reports of a relationship between BPA exposure and asthma-related consequences have been restricted to case reports of occupational asthma, especially among workers exposed to BPA in epoxy resins.910 The actual pathogenesis of BPA metabolism and related allergic disease remains to be clarified. Increasing evidence suggests that BPA-induced damage results in oxidative stress within the targeted cells.11 Disturbed oxidative homeostasis, through either in direct or indirect functional pathways, including mitochondrial function,12 modulation of antioxidant enzymes, and increased thiobarbituric acid reactive substances, can be seen in the brain, kidney, and testis of mice exposed to BPA throughout the embryonic/fetal route.13
Prenatal exposure to BPA, followed by postnatal allergic sensitization and challenges, is considered to promote the development of experimental allergic asthma.14 An association between increased odds ratio (OR) of a child developing wheeze before age 3 years and high (>2.2 µg/g creatinine) maternal urinary BPA concentrations in gestational week 16 has been reported by Spanier et al.15 Donohue et al.16 performed the first cohort study to report an association between postnatal urinary BPA concentrations and asthma in young children. They found that urinary BPA concentrations at ages 3, 5, and 7 years were associated with asthma assessed between 5 to 12 years of age. We also previously reported that early-life BPA exposure may increase the risk of atopic disorders.17 These findings add to the suspicion that environmental exposure to BPA might be associated with adverse respiratory outcomes in early childhood and may contribute to the risk of developing asthma in later life.
Airflow limitation and airway remodeling in asthma can result from an imbalance in redox systems favoring an oxidative environment.18 Glutathione S-transferase (GST) is thought to be involved in detoxification of BPA metabolites and reactive oxygen species (ROS).19 Several studies have also demonstrated that GST polymorphisms are associated with certain inflammatory diseases, including asthma.20
SOD2 rs4880, EPHX1 rs2740171, and GSTP1 rs1695 variants were also found to be highly associated with diisocyanate-induced asthma.21 This association with isocyanate-induced asthma supports the hypothesis that genetic variability within antioxidant defense systems may provide an environmental mechanism for developing asthma.21 In addition, related to other exposures, GSTM1 null and GSTP1 Ile105Val genotypes have been described to be associated with increased susceptibility to diesel exhaust particles (DEPs) and ozone exposure.2223 Finally, the SOD2 Ala allele has been reported to be linked to childhood asthma.24
Therefore, we tested a possible association between exposure to BPA and genes involved in oxidative ROS in a case-control study on asthma. First, in a screening step we will evaluate whether genetic polymorphisms of oxidative stress markers, GSTM1, GSTP1, SOD2, catalase (CAT), myeloperoxidase (MPO), and EPHX1 are related to a higher urine concentration of BPA metabolites. Second, for identified oxidative stress genes we will determine whether these modify the association of BPA exposure with asthma.
We conducted a case-control study comprised of 126 asthmatic children and 327 controls. Three control subjects were matched to each case subject by date of enrollment (within 3 months) if both had urine specimens and oral scrapes. All of the children were part of the Childhood Environment and Allergic diseases Study (CEAS) cohort recruited in 2010.25 Full enrollment in the study required the completion of BPA exposure monitoring of urine BPA metabolite levels and genotyping. At age 3 years, BPA metabolites were measured in urine samples, and genotyping was based on buccal cell samples. Parents were interviewed using a standardized questionnaire regarding basic demographics, birth history, parental age and education levels, family income, parental history of allergic diseases, duration of breast feeding, and environmental exposures of the child. Information about asthma was also collected. Written informed consent was obtained from all parents. Taipei Hospital's Institutional Review Board approved the study protocol (TH-IRB-0014-0011), which complied with the principles of the Helsinki Declaration.
An International Study of Asthma and Allergies in Childhood (ISAAC) questionnaire was used for collecting data on the children's allergic symptoms and information on duration of breast-feeding, number of older siblings, furry pets, carpets, or incensing at home, fungi at house walls, and tobacco smoke exposure were collected. The parents also answered a standardized questionnaire for basic demographics, birth history, parental history of allergic diseases, and family income.
Asthma was determined by pediatric allergists based at least on one of the following 3 criteria: 1) recurrence of at least 2 of the 3 symptoms: cough, wheeze, and shortness of breath within the previous 12 months without having a cold, 2) physician's diagnosis of asthma with ongoing treatment, and 3) response to treatment with β2-agonists or inhaled corticosteroids.26 Patients visiting the clinic without fulfilling the above criteria were defined as controls.
First mid-stream urine in the morning was collected from children at 3 years of age and stored at −20℃ until analysis. The urine samples were processed by solid-phase extraction. BPA metabolite (bisphenol A glucuronide [BPAG]) concentrations were determined using ultra-performance liquid chromatography/tandem mass spectrometry (UPLC-MS/MS) with isotopedilution techniques as described elsewhere.27 The limit of detection (LOD) was 1.61 ng/mL. For concentrations below the detection limit, a value of half the LOD was assigned. All results involved duplicate analysis. Urine creatinine levels were analyzed by enzymatic assay (Cayman Chemical, Ann Arbor, MI, USA).28 In all analyses, we adjusted for urine creatinine levels.
Genomic DNA was extracted from oral epithelial cells using a Gentra Purgene DNA Buccal Cell Kit (QIAGEN Sciences, Germantown, MD, USA). Candidate genes were selected based on their functional role in oxidative stress and inflammation. Quick-SNP version 1.1 (Carlsbad, CA, USA) was used to select 9 tag single nucleotide polymorphisms (SNPs) within the candidate genes that had a minor allele frequency (MAF) >5% and an r2>0.8 in Asian populations.29 The GSTM1, GSTP1 (rs1695), SOD2 (rs5746136), SOD2 (rs4880), CAT (rs769218), MPO (rs2071409), EPHX1 (rs1051740), and EPHX1 (rs2740171) polymorphisms (Supplementary Table 1), selected from hot spots of Asian populations on the National Center for Biotechnology information (NCBI) website, were analyzed by TaqMan SNP Genotyping Assay (ABI, Foster City, CA, USA) as described elsewhere.30 In addition, a deletion (null-mutation) of the GSTM1 gene was analyzed. All samples were measured using the same assay in duplicate to reduce the influence of unavoidable inter-assay variances. To control for errors and technical problems in genotyping, derived genotype frequencies were compared with the expected allelic population equilibrium based on the Hardy-Weinberg equilibrium test. Incomplete data missing at least 1 of 2 SNPs were excluded, and then individual haplotypes were estimated from genotype data.
Baseline characteristics of the case and control subjects were compared using the 2-sample t test for continuous variables or χ2 tests for categorical variables. Differences in geometric means of BPA metabolite concentrations (BPAG) among different genotypes were analyzed using the analysis of covariance (ANCOVA) test. To correct the skewed distribution of BPAG, the variable is log-transformed (LnBPAG) or geometric means are presented. To study the potentially modifying effect of oxidative stress genes, we analyze genotypes in asthma cases and controls. To estimate associations of genetic variants with asthma, ORs with 95% confidence intervals (CIs) were calculated by logistic regression. We determined whether one of the oxidative stress genes had an effect on the distribution of BPAG. To analyze combined effects of BPAG and genes, we then focus on those genes that affected the BPAG distribution in children. Since BPAG concentration seems to depend on oxidative stress genotypes, we were not allowed to simply test the interaction of BPAG with the oxidative stress genotypes, as unbiased assessments of interaction need to be based on 2 independent variables.31 To remove the dependency, we first regressed the BPAG levels on the oxidative stress genotypes and estimated the residuals of the BPAG concentration that is not explained by oxidative stress genotypes. Then, we tested the interaction of the BPAG residuals with the oxidative stress genes on asthma. The interactive effect was estimated only in the presence of statistically significant interaction term (Pinteraction≤0.05) as follows:
In all asthma models, potential confounders from literature reviews, such as gender, premature birth, maternal age and education, maternal history of atopy, family income, duration of breast feeding, number of older siblings, pet raising, environmental tobacco smoke (ETS) exposure, use of carpets at home, and fungi on house walls were taken into consideration. We adjusted for those confounders that resulted in a 10% change in point estimates when removed from the model. All hypothesis testing was 2-sided at a significance level of 0.05 and was performed with SAS software version 9.1 (SAS Institute, Cary, NC, USA).
Data on the family and environmental characteristics of the cases and controls revealed no significant differences between the 2 groups, with the exception of maternal history of atopy and ETS exposure (Table 1). Distribution of BPAG levels are shown in Supplementary Table 2.
The BPAG levels between asthma and controls was (geometric mean [standard error {SE}] 14.70 [1.07] vs 10.93 [1.12], P=0.019). Asthma was associated with the BPAG urine concentration (aOR, 1.29 per log unit increase in concentration; 95% CI, 1.08–1.55) (Table 2).
Children with the GSTP1 AA genotype had higher BPAG levels than GG carriers after adjusting for urine creatinine, age, gender, maternal history of atopy, and ETS exposure (geometric mean [SE] 12.72 [4.16] vs 11.42 [2.82]; P=0.036) (Table 3). No other oxidative stress gene affected the distribution of BPAG.
Significant differences in genotype frequency were found between asthma cases and controls for GSTP1 (rs1695), SOD2 (rs5746136), and EPHX1 (rs2740171) polymorphisms. GSTP1 AA, SOD2 TT, and EPHX1 AA genotypes were associated with a higher risk of asthma with an aOR (95% CI) of 3.00 (1.23–7.33), 2.78 (1.54–5.02), and 2.07 (1.00–4.24), compared with the GG, CC, and CC genotypes, respectively (Supplementary Table 3).
We further investigated the association of genotypes and asthma stratified by median BPAG levels. However, in this analysis we focused on GSTP1, since the genetic polymorphism of this gene was only associated with BPAG levels. In children with high BPAG level ≥6.55 ng/mL, the GSTP1 AA genotype was related to a higher odds of asthma than GG genotype (aOR, 4.84; 95% CI, 1.02–23.06) (Table 4). In children with BPAG level <6.55 ng/mL, there was no statistical significance.
Further, since BPAG urine concentrations differ among genotypes of GSTP1, to analyze combined effects we focused on the interplay of GSTP1 and BPAG with asthma. Table 4 shows that the adverse effects of BPA exposure are largely restricted to children carrying the GSTP1 AA genotype with higher BPAG levels. Residuals were estimated by subtracting the effect estimated for GSTP1 (rs1695). This was necessary, since the independence criterion for combined effects requires that gene and environmental effects are not associated, which they were, as shown above. Further, since GSTP1 was associated with asthma and BPAG levels differ among genotypes of GSTP1 (Table 3), we focused on the interplay of GSTP1 and BPAG levels with asthma. Before we can estimate whether there is an interaction between GSTP1 and BPAG resulting in a higher odds of having asthma, we needed to estimate the part of BPAG that is not explained by GSTP1 (the residuals). Then, we tested the interaction of the BPAG residuals with GSTP1 gene on asthma. Table 4 shows that the residual of BPAG in interaction with GSTP1 statistically significantly increases the odds of asthma. To further demonstrate this effect in a simpler model, we stratified the BPAG median levels. In addition, we controlled for known confounding factors, such as urine creatinine, age, gender, maternal history of atopy, maternal education, and ETS exposure. In the stratified analysis (Table 4, upper part, stratified by the median of BPAG levels), we can see that among the cases with lower BPAG levels (≤6.55 ng/mL) there are 65.8% with the GSTP1 AA genotype, similarly among the controls (63.7%). However, among cases with higher BPAG levels (>6.55 ng/mL), there are 74.0% with the GSTP1 AA genotype, but only 60.0% in the controls (OR, 4.84). From the lower half of Table 4, addressing the interaction on a multiplicative level, it is obvious that the OR for an individual with asthma is 2.92 times higher when both BPAG residual levels are higher and the child has a GSTP1 AA genotype. The interaction term on a multiplicative level is not statistically significant. However, additive interaction, determined by stratification, provides a better idea of the public health importance of this combined effect (OR, 4.84).
In this case-control study, we found an association between GSTP1 AA genetic variants in asthmatic patients and higher urine BPA metabolite concentrations. To the best of our knowledge, the associations between oxidative stress genotypes and urine BPA metabolite levels in children with asthma have not been studied before. We found that children with GSTP1 AA genotypes and higher urine BPA metabolite levels had 4.84-fold higher susceptibility to asthma. This finding supports our hypothesis that—assuming the GSTP1 gene participates in detoxification of BPA metabolites—the polymorphism of genes coding for GSTP1 may affect individual differences in urinary excretion of BPAG. Although a number of other studies supported a combined effect of BPA metabolites and GSTP1 polymorphisms,26 our findings need to be replicated in future studies, since we tested multiple oxidative stress genes and did not adjust for multiple testing in our case-control study. In addition, it is necessary to improve our understanding of the possible connection between exposure to environmental BPA, oxidative stress, and GSTP1 polymorphism in the process of developing asthma.
Our findings add to the body of findings supporting an association between postnatal urine BPAG concentrations and asthma in young children reported by Kuo Chou et al.24 and Bowler et al.32 We also identified that higher urine BPAG concentrations were associated with asthma occurrence. These results add to the evidence that environmental exposure to BPA might be associated with adverse respiratory outcomes.
A growing body of findings suggests that BPA-induced damage is associated with oxidative stress.26 The GSTP1 gene variant has also been reported to have a significantly lower level of GST enzyme activity. Indeed, several studies have shown that GST polymorphisms are associated with some inflammatory diseases, including asthma.33 Our analyses stratified by GSTP1 genotypes revealed that children with the AA genotype had higher BPAG concentrations, especially children with asthma. However, direct evidence for exogenous exposure of BPA and urine metabolites, and its modulation by GSTP1 enzyme polymorphisms is scant.34 The GSTP1 gene plays a significant role in antioxidant defense mechanisms. The balance between regression and progression of inflammation after exposure to an environmental insult can be determined by these genes.35 In zebrafish related to morphological changes, expression of the GSTP1 gene was reported to be up-regulated by high concentrations of tamoxifen. Tamoxifen may reverse the effects of estradiol and BPA.36 It has also been reported that BPA metabolites can be converted into glutathione conjugates in order to be excreted in urine. Thus, the expression of the GSTP1 gene may be responsible for conjugation of BPA metabolites with glutathione, which could increase with BPA exposure.37
Numerous reports have suggested indirect associations of oxidative stress-related genes with asthma occurrence. Inhaled diesel exhaust particles, ETS, ozone, and mold can each confer an increased risk of asthma symptom exacerbation in carriers of the Val105 allele.3839
GSTP1 polymorphisms have recently been reported to have a significant association with asthma risk.4041 Minor allele carriers of GSTP1 rs1695 were also related to an increased risk of asthma in several different study populations. 3842 Such traits were mainly manifested when children were exposed to ETS or to high levels of air pollution.3843 In this study, we found involvement of GST, SOD2, and EPHX1 genetic polymorphisms in asthma, suggesting the hypothesis that genetic variability within antioxidant defense systems can contribute to the pathogenesis of this inflammatory disease.
Using stratification for high and low BPAG concentration, we found that the GSTP1 AA genotype increased the odds of asthma in children with high BPAG concentrations. This supports the hypothesis that a less efficient defense against oxidative stress products may contribute to the pathogenesis of asthma. BPA is considered to induce oxidative stress by disrupting the activities of antioxidant enzymes.44 In addition, it has been reported that variations in SOD2, GST, and EPHX1 genes contribute to BPA susceptibility.21 Then, oxidative stress may lead to airway inflammation and decrease pulmonary function as reported in rats exposed to pollutant mixtures.45 In support of our findings, children living in high PM10 communities, the GSTP1 Ile/Val or Val/Val genotype were the high-risk genotype for childhood asthma.40 The Taiwan Children Health Study Group also reported that exposure to PM2.5 and O3 was associated positively with a greater risk of asthma among those with at least 1 Val105 allele of the GSTP1 gene. In the Cincinnati Childhood Allergy and Air Pollution Study birth cohort, high exposure to DEP was associated with wheezing phenotypes only in children with the GSTP1 Val105 allele (rs1695).46
GSTP1 polymorphism has never been reported to modify the association of urine BPA metabolite levels and asthma. Also, the role of polymorphism of GSTP1 in urine excretion of BPA metabolites has not been assessed to date. Our study was based on the hypothesis that if GSTP1 participate in detoxification of BPA metabolites, the polymorphism of genes coding for GSTP1 may affect individual differences in urine excretion of BPAG. This mechanism can be very similar to processes identified by the association between exogenous exposure of polycyclic aromatic hydrocarbons (PAHs) and urine metabolites and its modulation by GSTP1 enzyme polymorphisms.47 One hundred seventy German workers exposed to PAHs were surveyed by a cross-sectional study to explore the role of 11 polymorphisms of CYP1A1, CYP1A2, CYP1B1, CYP3A4, EPHX1, GSTM1, GSTT1, and GSTP1 in the connection between occupational exposure to PAH and urine PAH metabolites. A significantly increased 1-OHP level was associated with GSTP1 variants.
Some potential limitations may affect the conclusion of this study. First, our study was conducted using a targeted candidate gene approach. In the past, we relied on a prior understanding of the functional features of possible genetic candidates, which may result in blocking the detection of novel. However, prior knowledge helped us focus. Hence, we deliberately selected 7 candidate genes involved in the pathogenesis of oxidative pathways for screening and validation in this study. This focused approach was cost-effective compared to a genome-wide approach. Second, we are aware that BPA measurements in spot urine samples can be limited as other studies also have shown low intra-class correlation coefficient of spot urine sample. However, they are considered to adequately reflect average population exposure when sample sizes are large.48 Moreover, other investigations showed that single spot samples obtained from children provided a reliable characterization of absolute and relative exposure over the short time window studied.49 Moreover, the reliability of assessment of BPA metabolites was also enhanced by analyzing urine BPA metabolites using UPLC-MS/MS with decent validity.
We have strengthened our study using direct measurement of exposure through objective quantitative biomarkers for BPA metabolites and assessment of the outcome by physician diagnoses of asthma. We also intended to reduce the recall bias of exposure assessment derived from questionnaires by directly assessing BPA exposure through quantifying urine BPA metabolites. Thus, we were able to test for our genetic associations with adjustment for potential confounders, such as urine creatinine, age, gender, maternal history of atopy, maternal education, and ETS exposure.
In conclusion, this case-control study found that the GSTP1 rs1695 variants are significantly associated with asthma particularly in children with higher BPA urine concentrations, thus supporting the hypothesis that genetic variability within antioxidant defense systems contributes to the pathogenesis of this disease. The oxidative stress gene GSTP1 rs1695 polymorphism seems to modify the association of BPA exposures with asthma. Since adverse effects of BPA exposure were largely restricted to children carrying GSTP1 AA genotypes, preventive measures to avoid BPA exposure should be introduced in these susceptible children. However, to reach a firm conclusion, future studies need to replicate our findings. In addition, there is a requirement to standardize methods for environmental exposure assessment.
References
1. Bhan A, Hussain I, Ansari KI, Bobzean SA, Perrotti LI, Mandal SS. Bisphenol-A and diethylstilbestrol exposure induces the expression of breast cancer associated long noncoding RNA HOTAIR in vitro and in vivo. J Steroid Biochem Mol Biol. 2014; 141:160–170. PMID: 24533973.
2. Dodson RE, Nishioka M, Standley LJ, Perovich LJ, Brody JG, Rudel RA. Endocrine disruptors and asthma-associated chemicals in consumer products. Environ Health Perspect. 2012; 120:935–943. PMID: 22398195.


3. Gregoraszczuk EL, Ptak A. Endocrine-disrupting chemicals: some actions of pops on female reproduction. Int J Endocrinol. 2013; 2013:828532. PMID: 23762054.


4. Teeguarden JG, Hanson-Drury S. A systematic review of Bisphenol A “low dose” studies in the context of human exposure: a case for establishing standards for reporting “low-dose” effects of chemicals. Food Chem Toxicol. 2013; 62:935–948. PMID: 23867546.


5. Rochester JR. Bisphenol A and human health: a review of the literature. Reprod Toxicol. 2013; 42:132–155. PMID: 23994667.


6. Chapin RE, Adams J, Boekelheide K, Gray LE Jr, Hayward SW, Lees PS, et al. NTP-CERHR expert panel report on the reproductive and developmental toxicity of bisphenol A. Birth Defects Res B Dev Reprod Toxicol. 2008; 83:157–395. PMID: 18613034.


7. Calafat AM, Ye X, Wong LY, Reidy JA, Needham LL. Exposure of the U.S. population to bisphenol A and 4-tertiary-octylphenol: 2003-2004. Environ Health Perspect. 2008; 116:39–44. PMID: 18197297.
8. Matsumoto J, Yokota H, Yuasa A. Developmental increases in rat hepatic microsomal UDP-glucuronosyltransferase activities toward xenoestrogens and decreases during pregnancy. Environ Health Perspect. 2002; 110:193–196. PMID: 11836149.


9. Hannu T, Frilander H, Kauppi P, Kuuliala O, Alanko K. IgE-mediated occupational asthma from epoxy resin. Int Arch Allergy Immunol. 2009; 148:41–44. PMID: 18716402.
10. Kwak ES, Just A, Whyatt R, Miller RL. Phthalates, pesticides, and bisphenol-A exposure and the development of nonoccupational asthma and allergies: how valid are the links? Open Allergy J. 2009; 2:45–50. PMID: 20622976.
11. Anjum S, Rahman S, Kaur M, Ahmad F, Rashid H, Ansari RA, et al. Melatonin ameliorates bisphenol A-induced biochemical toxicity in testicular mitochondria of mouse. Food Chem Toxicol. 2011; 49:2849–2854. PMID: 21840368.


12. Ooe H, Taira T, Iguchi-Ariga SM, Ariga H. Induction of reactive oxygen species by bisphenol A and abrogation of bisphenol A-induced cell injury by DJ-1. Toxicol Sci. 2005; 88:114–126. PMID: 16093527.


13. Kabuto H, Amakawa M, Shishibori T. Exposure to bisphenol A during embryonic/fetal life and infancy increases oxidative injury and causes underdevelopment of the brain and testis in mice. Life Sci. 2004; 74:2931–2940. PMID: 15051418.


14. Nakajima Y, Goldblum RM, Midoro-Horiuti T. Fetal exposure to bisphenol A as a risk factor for the development of childhood asthma: an animal model study. Environ Health. 2012; 11:8. PMID: 22353195.


15. Spanier AJ, Kahn RS, Kunselman AR, Hornung R, Xu Y, Calafat AM, et al. Prenatal exposure to bisphenol A and child wheeze from birth to 3 years of age. Environ Health Perspect. 2012; 120:916–920. PMID: 22334053.


16. Donohue KM, Miller RL, Perzanowski MS, Just AC, Hoepner LA, Arunajadai S, et al. Prenatal and postnatal bisphenol A exposure and asthma development among inner-city children. J Allergy Clin Immunol. 2013; 131:736–742. PMID: 23452902.


17. Wang IJ, Chen CY, Bornehag CG. Bisphenol A exposure may increase the risk of development of atopic disorders in children. Int J Hyg Environ Health. 2016; 219:311–316. PMID: 26765087.


18. Comhair SA, Erzurum SC. Redox control of asthma: molecular mechanisms and therapeutic opportunities. Antioxid Redox Signal. 2010; 12:93–124. PMID: 19634987.


19. Sheehan D, Meade G, Foley VM, Dowd CA. Structure, function and evolution of glutathione transferases: implications for classification of non-mammalian members of an ancient enzyme superfamily. Biochem J. 2001; 360:1–16. PMID: 11695986.


20. Piacentini S, Polimanti R, Simonelli I, Donno S, Pasqualetti P, Manfellotto D, et al. Glutathione S-transferase polymorphisms, asthma susceptibility and confounding variables: a meta-analysis. Mol Biol Rep. 2013; 40:3299–3313. PMID: 23307299.


21. Yucesoy B, Johnson VJ, Lummus ZL, Kissling GE, Fluharty K, Gautrin D, et al. Genetic variants in antioxidant genes are associated with diisocyanate-induced asthma. Toxicol Sci. 2012; 129:166–173. PMID: 22610343.


22. Gilliland FD. Outdoor air pollution, genetic susceptibility, and asthma management: opportunities for intervention to reduce the burden of asthma. Pediatrics. 2009; 123(Suppl 3):S168–S173. PMID: 19221160.


23. Romieu I, Ramirez-Aguilar M, Sienra-Monge JJ, Moreno-Macías H, del Rio-Navarro BE, David G, et al. GSTM1 and GSTP1 and respiratory health in asthmatic children exposed to ozone. Eur Respir J. 2006; 28:953–959. PMID: 16870661.
24. Kuo Chou TN, Li YS, Lue KH, Liao CF, Lin CY, Tzeng PR, et al. Genetic polymorphism of manganese superoxide dismutase is associated with childhood asthma. J Asthma. 2010; 47:532–538. PMID: 20536280.


25. Wang IJ, Lin TJ. FLG P478S polymorphisms and environmental risk factors for the atopic march in Taiwanese children: a prospective cohort study. Ann Allergy Asthma Immunol. 2015; 114:52–57. PMID: 25528737.


26. Soto-Martínez ME, Avila L, Soto-Quirós ME. New criteria for the diagnosis and management of asthma in children under 5 years old: GINA Guidelines 2009. An Pediatr (Barc). 2009; 71:91–94. PMID: 19608470.
27. Zhou X, Kramer JP, Calafat AM, Ye X. Automated on-line column-switching high performance liquid chromatography isotope dilution tandem mass spectrometry method for the quantification of bisphenol A, bisphenol F, bisphenol S, and 11 other phenols in urine. J Chromatogr B Analyt Technol Biomed Life Sci. 2014; 944:152–156.


28. Cayman Chemical (US). Creatinine (urinary) [Internet]. Ann Arbor (MI): Cayman Chemical;2012. cited 2017 Sep 15. Available from: https://www.caymanchem.com/pdfs/500701.pdf.
29. Grover D, Woodfield AS, Verma R, Zandi PP, Levinson DF, Potash JB. QuickSNP: an automated web server for selection of tagSNPs. Nucleic Acids Res. 2007; 35:W115–W120. PMID: 17517769.


30. Woodward J. Bi-allelic SNP genotyping using the TaqMan® assay. Methods Mol Biol. 2014; 1145:67–74. PMID: 24816660.


31. McClelland GH, Judd CM. Statistical difficulties of detecting interactions and moderator effects. Psychol Bull. 1993; 114:376–390. PMID: 8416037.


32. Bowler RP, Barnes PJ, Crapo JD. The role of oxidative stress in chronic obstructive pulmonary disease. COPD. 2004; 1:255–277. PMID: 17136992.


33. Hwang BF, Young LH, Tsai CH, Tung KY, Wang PC, Su MW, et al. Fine particle, ozone exposure, and asthma/wheezing: effect modification by glutathione S-transferase P1 polymorphisms. PLoS One. 2013; 8:e52715. PMID: 23357926.


34. Zielińska E, Zubowska M, Misiura K. Role of GSTM1, GSTP1, and GSTT1 gene polymorphism in ifosfamide metabolism affecting neurotoxicity and nephrotoxicity in children. J Pediatr Hematol Oncol. 2005; 27:582–589. PMID: 16282887.
35. Selgrade MK, Blain RB, Fedak KM, Cawley MA. Potential risk of asthma associated with in utero exposure to xenobiotics. Birth Defects Res C Embryo Today. 2013; 99:1–13. PMID: 23723168.


36. Xia L, Zheng L, Zhou JL. Transcriptional and morphological effects of tamoxifen on the early development of zebrafish (Danio rerio). J Appl Toxicol. 2016; 36:853–862. PMID: 26584595.


37. Sienkiewicz M. In vitro effects of bisphenol a on prostate cells: searching for clues of environmental carcinogenesis [Internet]. Ottawa: University of Ottawa;2012. cited 2017 July 1. Available from: https://www.ruor.uottawa.ca/bitstream/10393/22805/3/Sienkiewicz_Marta_2012_thesis.pdf.
38. Tamer L, Calikoğlu M, Ates NA, Yildirim H, Ercan B, Saritas E, et al. Glutathione-S-transferase gene polymorphisms (GSTT1, GSTM1, GSTP1) as increased risk factors for asthma. Respirology. 2004; 9:493–498. PMID: 15612961.
39. Palmer CN, Doney AS, Lee SP, Murrie I, Ismail T, Macgregor DF, et al. Glutathione S-transferase M1 and P1 genotype, passive smoking, and peak expiratory flow in asthma. Pediatrics. 2006; 118:710–716. PMID: 16882827.


40. Su MW, Tsai CH, Tung KY, Hwang BF, Liang PH, Chiang BL, et al. GSTP1 is a hub gene for gene-air pollution interactions on childhood asthma. Allergy. 2013; 68:1614–1617. PMID: 24117884.
41. Lee YL, Hsiue TR, Lee YC, Lin YC, Guo YL. The association between glutathione S-transferase P1, M1 polymorphisms and asthma in Taiwanese schoolchildren. Chest. 2005; 128:1156–1162. PMID: 16162701.


42. Yu J, Ahn K, Shin YH, Kim KW, Suh DI, Yu HS, et al. Interaction between prenatal exposure to home renovation and reactive oxygen species genes in cord blood IgE response is modified by maternal atopy. Allergy Asthma Immunol Res. 2016; 8:41–48. PMID: 26540500.
43. MacIntyre EA, Brauer M, Melén E, Bauer CP, Bauer M, Berdel D, et al. GSTP1 and TNF Gene variants and associations between air pollution and incident childhood asthma: the traffic, asthma and genetics (TAG) study. Environ Health Perspect. 2014; 122:418–424. PMID: 24465030.
44. Zhang J, Li X, Zhou L, Wang L, Zhou Q, Huang X. Analysis of effects of a new environmental pollutant, bisphenol A, on antioxidant systems in soybean roots at different growth stages. Sci Rep. 2016; 6:23782. PMID: 27030053.


45. Seagrave J, Campen MJ, McDonald JD, Mauderly JL, Rohr AC. Oxidative stress, inflammation, and pulmonary function assessment in rats exposed to laboratory-generated pollutant mixtures. J Toxicol Environ Health A. 2008; 71:1352–1362. PMID: 18704828.


46. Schroer KT, Biagini Myers JM, Ryan PH, LeMasters GK, Bernstein DI, Villareal M, et al. Associations between multiple environmental exposures and Glutathione S-Transferase P1 on persistent wheezing in a birth cohort. J Pediatr. 2009; 154:401–408. 408.e1PMID: 18950799.


47. Rihs HP, Pesch B, Kappler M, Rabstein S, Rossbach B, Angerer J, et al. Occupational exposure to polycyclic aromatic hydrocarbons in German industries: association between exogenous exposure and urinary metabolites and its modulation by enzyme polymorphisms. Toxicol Lett. 2005; 157:241–255. PMID: 15917149.


48. Nepomnaschy PA, Baird DD, Weinberg CR, Hoppin JA, Longnecker MP, Wilcox AJ. Within-person variability in urinary bisphenol A concentrations: measurements from specimens after long-term frozen storage. Environ Res. 2009; 109:734–737. PMID: 19463991.


49. Heffernan AL, Aylward LL, Samidurai AJ, Davies PS, Toms LM, Sly PD, et al. Short term variability in urinary bisphenol A in Australian children. Environ Int. 2014; 68:139–143. PMID: 24727068.


Table 1
Characteristics of the study population
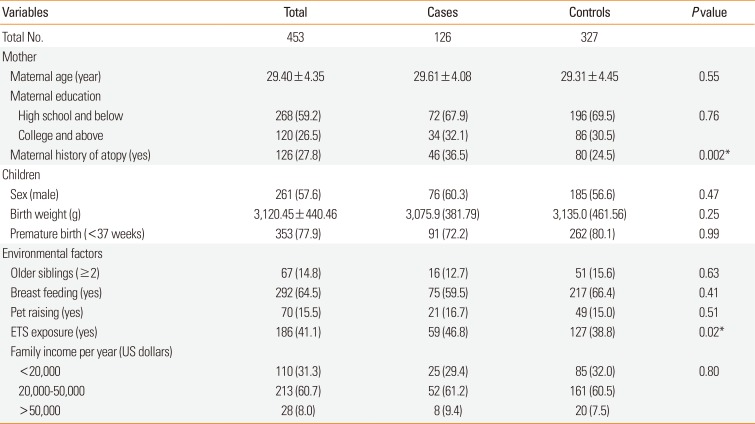
Table 2
Association of urine BPAG levels (ng/mL) with asthma

Variables* | LnBPAG† |
---|---|
Asthma crude OR (95% CI) | 1.21 (1.03–1.43) |
Asthma aOR (95% CI) | 1.29 (1.08–1.55) |
Table 3
Association of urine BPAG levels (ng/mL) with genotypes
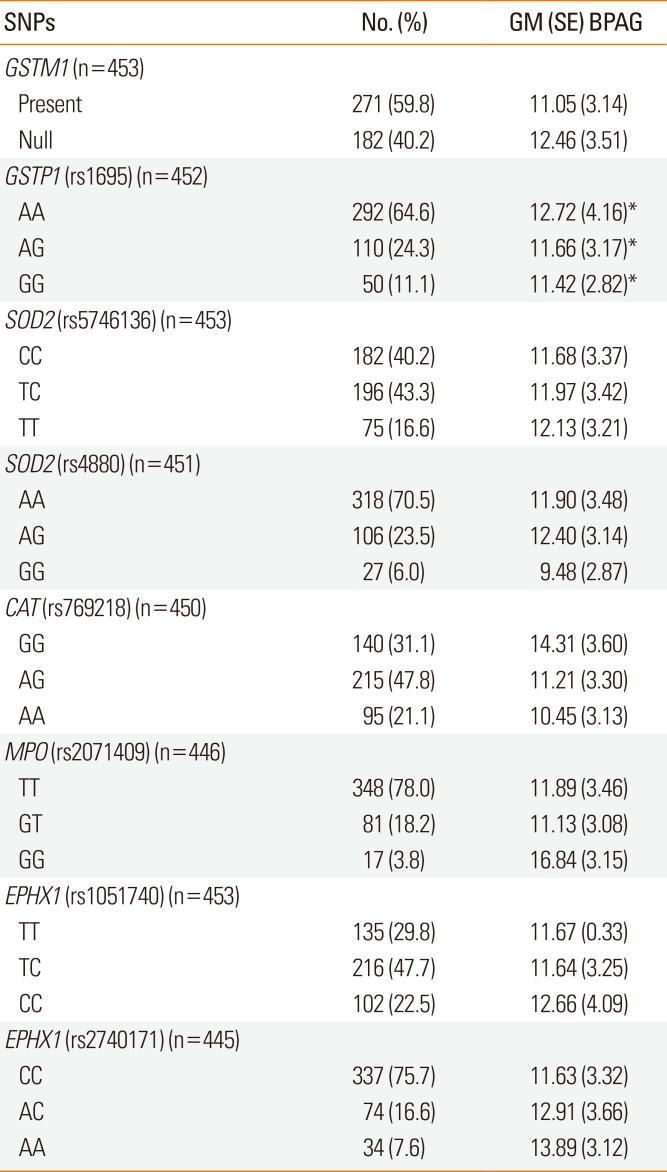
Table 4
The combined effects of BPAG levels and genotypes on asthma. The additive interaction of GSTP1 genotype identified by stratification and BPAG median levels and the multiplicative interaction effect of the GSTP1 gene and BPAG residuals on asthma
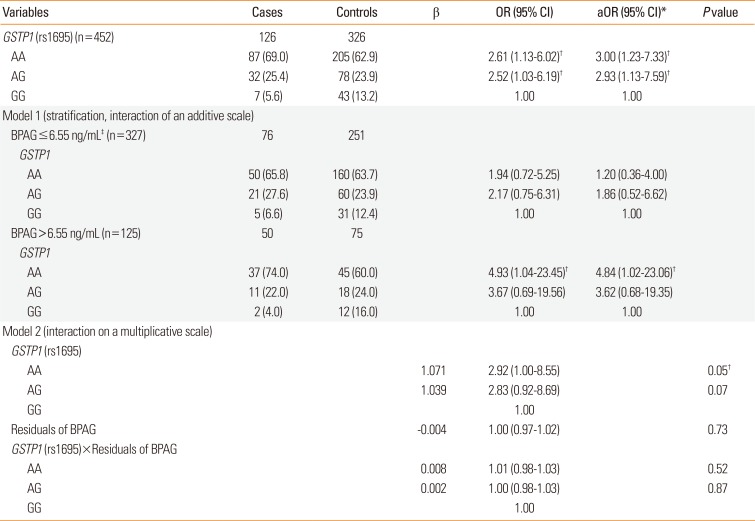
Data are shown as number (%). Model 1 is GSTP1 (rs1695) for BPAG<6.55 ng/mL and/or ≥6.55 ng/mL, and model 2 is GSTP1 (rs1695)+residuals of BPAG+GSTP1 (rs1695)×residuals of BPAG.
BPAG, bisphenol A glucuronide; OR, odds ratio; CI, confidence interval; aOR, adjusted odds ratio.
*Adjusted for urine creatinine, age, gender, maternal history of atopy, maternal education, and environmental tobacco smoke exposure; †P<0.05; ‡6.55 ng/mL is the median.