Abstract
Despite asthma being the most common chronic childhood ailment, there is still much to learn about the disease. Early childhood infections with well-known or emerging viruses can lay the pathophysiologic framework for asthma development and exacerbation later in life, which may be due partly to alteration of the airway microbiome. Once asthma is established, acute exacerbations are usually associated with infections with respiratory viruses, such as rhinoviruses (RVs). Once again, there are bidirectional interactions between viruses and airway bacteria that appear to influence the severity of illness and the likelihood of exacerbation. Studies employing recent advances in viral and bacterial identification analytic techniques will clarify these new concepts and may provide the basis for new treatments or prevention or respiratory infection-associated exacerbation. This paper is a review of the associations among respiratory viruses, bacteria, inflammatory mechanisms, and asthma exacerbation.
In the past few decades, highly sensitive and specific tests for virus detection have been developed, resulting in accurate detection of a long list of viruses associated with wheezing in early life, the risk for developing asthma, and exacerbation of established disease. Human rhinovirus (RV) and respiratory syncytial virus (RSV) top the list, but recently discovered or re-emerging viruses (e.g., human metapneumovirus [HMPV] coronaviruses and enterovirus [EV]-D68) also contribute to acute wheezing in infancy and virus-mediated exacerbations. However, most viral infections are not associated with acute exacerations, and cofactors, including allergic inflammation and airway bacteria, have been described that increase the severity of infection and the probability of exacerbation.12 Detection of both viruses and airway bacteria are associated with acute wheezing illnesses; specifically, co-infections of respiratory viruses and Moraxella catarrhalis, Hemophilus influenza, and/or Streptococcus pneumonia increases the risk for more severe respiratory illnesses and exacerbations of asthma.3 The addition of antibiotics to treatment regimens for asthma exacerbation could materialize in the near future.
Several recent studies have also shown that respiratory allergy may partner with certain viral infections to synergistically produce airway inflammation and increase cold and asthma symptoms.4 Since there is currently no safe and effective antiviral for RV, the targeting of allergic inflammation may prove beneficial in reducing risk of severe virus-induced asthma symptoms.5 There are several possible immunopathologic mechanism(s) that connect viral and bacterial infection, allergy, and acute asthma, as discussed in the following sections.
Asthma exacerbation is defined as an acute or subacute worsening of symptoms and lung function as compared to the patient's usual health status or, occasionally, the initial presentation of asthma.6 Exacerbations often represent essentially an exaggerated lower airway response to an environmental exposure, with airway inflammation being a key component that promotes airflow obstruction and increased airway hyperresponsiveness.7 Early treatment of asthma exacerbation is considered the best strategy for management,8 and increases in eosinophilic inflammatory markers have been shown to be good predictors of asthma exacerbations.9 Utilizing this idea, tailoring patient treatment based on the direct and objective measurement of the eosinophil, a key component of airway inflammation in asthma, has proven more efficacious (e.g., less frequent and severe asthma exacerbations) than treatment based on clinical symptoms and lung function.10
Exacerbations usually occur in response to a variety of external agents (e.g., respiratory pathogens, allergens, air pollutants, smoke, and cold or dry air); however, it is estimated that up to 85% of asthma exacerbations in children11 and a considerable proportion in adults (76%-80%)12 are linked to viral infections. The severity of the situation is compounded by the lack of specific antiviral strategies. Virus-induced asthma exacerbations are most often associated with detection of RV,5 RSV,13 human parainfluenza virus (HPIV),14 human influenza virus (HIFV),15 and human adenovirus (HAdV).16 More recently, human bocavirus (HBoV), HMPV,17 certain strains of human coronavirus (HCoV) (e.g., HCoV-NL63, HCoV-OC43, and HCoV-229E),18 and EV-D6819 have been associated with asthma exacerbation.
Viral identification in early childhood wheezing illness may provide prognostic information regarding short- and long-term consequences. In the short term, RSV-induced bronchiolitis is associated with longer hospitalization than RV-induced bronchiolitis.20 The risk of reinfection with certain viruses varies; for example, there is a strong likelihood of RSV reinfection in the absence of ongoing passive immunity.21 Two studies found this risk to be as high as 74%22 and 76%23 if the initial infection occurred within the first year of life. Fourth, certain respiratory viruses have a well-documented association with subsequent asthma development and then exacerbation. More severe RSV illnesses in early childhood increase the risk of asthma development,24 and the risk for developing asthma is even greater after a RV wheezing episode in early life.25 Some children may have genetic risk factors for wheezing with RV26 or with RSV.
RV is the most prevalent human respiratory virus and is classified into 3 species (A, B, and C), with each of these species comprised of a number of different types: A (80 types), B (32 types), and C (up to 55 types).28 Within each species, there is considerable genetic and antigenic heterogeneity;28 while among species, A and C are phylogenetically closer than B and C. RV-A and RV-B were originally classified according to sensitivity to capsid-binding drugs, and RV-C was classified by sequence-based criteria.29 RV circulates throughout the year but in many geographic areas appears to have distinct peaks of incidence in the fall and spring.30 Infection rates remain substantial for the rest of the year.
In both adults and children, RV is the most frequently identified virus associated with acute asthma exacerbation.31 RV enters the body through the respiratory route and infects and replicates in epithelial cells. It was first thought that RV infected only the upper airway, but it is now known that it can also infect the lower airway.32 One of the proposed reasons why asthmatics are predisposed to viral infection is damaged epithelium, which may increase susceptibility to infection and ultimately lead to airway obstruction. Cellular mechanisms involved in this response include higher intracellular adhesion molecule-1 (ICAM-1) expression on respiratory epithelial cells, disruption of epithelial barrier function, impaired apoptosis, increased cell lysis, deficient Th1 response (e.g., interferon [IFN]-γ), and enhanced neutrophil recruitment.7 RV infection also induces pro-inflammatory mediators, including interleukin (IL)-6, IL-8, tumor necrosis factor-α (TNF-α), IL-β, regulated on activation normal T expressed and secreted (RANTES), and granulocyte-macrophage colony stimulating factor (GM-CSF).33
In addition to increasing airway neutrophils, RV-induced asthma exacerbation can also be associated with increases in airway eosinophils (Eos).2 Airway Eos bind to RV and may participate in virus-specific immune responses through antigen presentation and T cell activation.34 Eos may contribute to antiviral responses to RSV in vitro,35 but the clinical significance of these effects is unclear. Eos can have immunomodulating effects, including inducing T cell activation, and eosinophilic cationic protein (ECP) can upregulate ICAM-1 expression on respiratory epithelial cells.36 It should be noted that 90% of RV-A and RV-B serotypes use ICAM-1 as a receptor, which has a pivotal role in movement of inflammatory cells into the airways.37 RV-C types bind to cadherin-related protein-3 (CDHR3), and the corresponding gene has been linked to childhood asthma associated with severe wheezing episodes in early life.38
The 2 antigenic/genetic subtypes of RSV (A and B) exhibit seasonality, with RSV-A predominating in the fall/early winter and RSV-B in the winter and early spring.39 RSV-A is associated with more severe illness, while RSV-B has been characterized as the milder strain.40 Knowing which subtype a patient is infected with may, therefore, have some clinical value—such as treatment, prognosis, and even prevention—as more therapeutic and prophylactic modalities become available.39
In addition to causing acute wheezing and bronchiolitis in infants, RSV can also contribute to asthma exacerbations in children and adults.41 RSV infection of the airway induces airway inflammation and can promote airway hyperresponsiveness. Airway epithelial cells initially respond to RSV infection by releasing type I IFNs, IL-12, IL-18,42 and a variety of cytokines and chemokines, including IL-8, IL-10, RANTES, macrophage inflammatory protein (MIP)-1α, monocyte chemotactic protein (MCP)-1, and eotaxin.43 After migration to the lymph nodes for viral antigen presentation to T cells, dendritic cells migrate back to the infected epithelium, release mediators again (e.g., IFN-γ, IL-2, IL-4, IL-5, IL-9, and IL-12), and recruit additional inflam-matory cells (e.g., CD8+ T cells and B cells), and granulocytes (neutrophils and Eos).44
Under some circumstances, RSV infection may promote a T helper 2 (Th2) bias in immune responses in humans41 and animals.42 This was also found in a study comparing differential airway inflammatory responses in RSV- and influenza-induced asthma exacerbations. IFN-γ and TNF-α levels were significantly lower in RSV-induced asthma exacerbation when compared to asthmatics infected with influenza.45 Indeed, the weaker Th1 response during viral infection in these individuals may lead to reduced viral clearance and prolonged and/or more serious disease. RSV infection is a potent inducer of chemokines,46 and RANTES and eotaxin in particular have been associated with increased eosinophilia and RSV disease severity in mice.47 Eotaxin appears to be a key component of Th2-driven disease,48 and eotaxin levels correlate closely with eosinophil degranula-tion (i.e., eosinophil-derived neurotoxin [EDN] and ECP) in pediatric asthma.49 Consequently, it may be a good therapeutic target in asthma and other eosinophil-related disease.
Host factors may be critical in determining RSV-induced disease severity, even when taking age-related effects, current/recent infection, air pollution, and allergen exposure into account.44 Host genetic studies have found several polymorphisms to relate to RSV severity, including those in the chemokine receptor CCR550 (which binds a number of inflammatory CC-chemokines, including MIP-1α, MIP-1β, and RANTES), CXCL8, IL-4, and promoter variants of IL-9, IL-10, and TNF-α.51 Thus, gene-environment interactions may contribute to susceptibility to virus-induced disease.
All EVs, including poliovirus, circulate mostly in the summer and fall in temperate and year-round in tropical climes.52 There are more than 100 nonpolio human EVs, with the newer numbered ones being classified into 4 species, EV-A, EV-B, EV-C, and EV-D. EV-D includes 3 serotypes known to cause disease in humans: EV-D68, EV-D70, and EV-D94.53 Although EV-D68 is not new, having first been isolated in 1962 from a small group of US children with lower respiratory tract infection, it has emerged in the past decade as an important respiratory pathogen, with reports from North America, Europe, and Asia. Its association with severe respiratory disease is of special note in children with asthma.19 Exactly how it is able to cause lower respiratory tract disease is currently unknown because the virus binds specifically to cell receptors found predominantly in the upper respiratory tract;54 however, additional mediators are required for EV-D68 to enter cells.55 There is evidence that recent strains of EV-D68 that are associated with lower respiratory tract infection may have gene mutations that influence antigenicity or trophism for lower airway cells.
Most asthma exacerbations are associated with viral infection. However, infections with RV and other respiratory viruses are quite common, and most natural (or experimentally induced) infections in asthmatics do not result in exacerbations.12 As discussed above, RV species and host genetics can influence the severity of illness and the probability of wheezing illnesses. Additional factors that influence the severity of viral infection and the risk of developing an asthma exacerbation are allergic sensitization and the airway bacteria (Figure).
RV infection and allergic sensitization synergistically increase the risk of wheezing illnesses.56 Atopic asthma can be associated with reduced virus-induced IFN responses, decreased viral clearance57 and increased viral shedding.58 In some studies, atopic asthmatics vs non-atopic controls have reduced peripheral blood mononuclear cell (PBMC) IFN responses.59 Furthermore, some studies have found that bronchial epithelial cells of atopic asthmatics permit increased RV replication, enhanced cell lysis, and deficient release of IFN-β.60 Studies of children with allergic asthma treated with omalizumab indicate that neutralizing immunoglobulin E (IgE)-mediated inflammation can lead to improvements in IFN responses and reduced virus-induced exacerbations.61 This suggests that IgE-mediated inflammation can inhibit antiviral responses and increase the risk for virus-induced exacerbations of asthma.
Several studies have shown that viral infections precede bacterial infections of the upper and lower airways and may affect disease severity (e.g., increased invasive pneumococcal disease during high RV and RSV activity).62 This may occur for several reasons, including virus-induced expression of airway receptors used by bacteria for cell invasion and disruption of airway epithelial layers.63 Viruses and bacteria also increase release of inflammatory cytokines and mediators from epithelial cells and leukocytes, causing increased inflammation with a concomitant increase in symptoms and risk of asthma exacerbation.3
Patients with stable asthma have even exhibited increased colonization of upper and lower airways with Proteobacteria, a group of bacteria predominantly composed of gram-negative species.64 The possible role of bacteria in the early stages of asthma has been demonstrated by Bisgaard et al.65 who found that S. pneumonia, H. influenzae, or M. catarrhalis colonization of the upper airway in early infancy was associated with increased risk of recurrent wheezing leading to asthma. Furthermore, wheezing illnesses in young children are similarly associated with detection of either viral or bacterial pathogens in the airway.66 RV infections can promote increased detection of bacterial pathogens, and the combination of RV infection with detection of respiratory pathogens is associated with a greater risk for illness, including exacerbation of asthma.3 Similarly, acute wheezing illnesses in infants or school-aged children are associated with both viral infections and airway microbiota that are dominated by bacterial pathogens.67
This raises the possibility that selective use of antibiotics or vaccination could be useful in asthma. In wheezing infants, 2 controlled studies have reported that azithromycin produced clinical benefit in infants with acute wheezing.68 Whether this is due to antimicrobial effects or anti-inflammatory effects of the macrolide is uncertain. Given that repeated antibiotic therapy could have adverse effects on commensal bacteria, and could also add to the problem of drug-resistant bacteria, more research is needed on long-term effects of this approach.
Improved testing methods have helped demonstrate that infections with several respiratory viruses are important contributors to asthma exacerbation and possibly the development of asthma in early childhood. Cofactors, such as genetics, allergic inflammation, and the airway microbiome, also modify the risk of more severe viral illness and exacerbation of established asthma. While practical antiviral strategies are lacking, there may be other opportunities to prevent or treat virus-induced exacerbations by reducing airway inflammation or perhaps targeting microbial cofactors.
ACKNOWLEDGMENT
This research was supported in part by the Sabbatical Program 2012 from Inje University.
References
1. Cheung D, Dick EC, Timmers MC, de Klerk EP, Spaan WJ, Sterk PJ. Rhinovirus inhalation causes long-lasting excessive airway narrowing in response to methacholine in asthmatic subjects in vivo. Am J Respir Crit Care Med. 1995; 152:1490–1496. PMID: 7582282.
2. Fraenkel DJ, Bardin PG, Sanderson G, Lampe F, Johnston SL, Holgate ST. Lower airways inflammation during rhinovirus colds in normal and in asthmatic subjects. Am J Respir Crit Care Med. 1995; 151:879–886. PMID: 7881686.


3. Kloepfer KM, Lee WM, Pappas TE, Kang TJ, Vrtis RF, Evans MD, et al. Detection of pathogenic bacteria during rhinovirus infection is associated with increased respiratory symptoms and asthma exacerbations. J Allergy Clin Immunol. 2014; 133:1301–1307. 1307.e1–1307.e3. PMID: 24698319.


4. Murray CS, Poletti G, Kebadze T, Morris J, Woodcock A, Johnston SL, et al. Study of modifiable risk factors for asthma exacerbations: virus infection and allergen exposure increase the risk of asthma hospital admissions in children. Thorax. 2006; 61:376–382. PMID: 16384881.


5. Olenec JP, Kim WK, Lee WM, Vang F, Pappas TE, Salazar LE, et al. Weekly monitoring of children with asthma for infections and illness during common cold seasons. J Allergy Clin Immunol. 2010; 125:1001–1006.e1. PMID: 20392488.


6. Global Asthma Network (NZ). The global asthma report 2014 [Internet]. Auckland: Global Asthma Network;2014. cited 2016 Jun 27. Available from: http://www.globalasthmareport.org/burden/burden.php.
7. Wark PA, Gibson PG. Asthma exacerbations · 3: pathogenesis. Thorax. 2006; 61:909–915. PMID: 17008482.
8. National Asthma Education and Prevention Program (US). Expert panel report 3 (EPR-3): guidelines for the diagnosis and management of asthma: full report. Bethesda (MD): National Heart, Lung, and Blood Institute;2007.
9. Pizzichini MM, Pizzichini E, Clelland L, Efthimiadis A, Pavord I, Dolovich J, et al. Prednisone-dependent asthma: inflammatory indices in induced sputum. Eur Respir J. 1999; 13:15–21. PMID: 10836317.


10. Green RH, Brightling CE, McKenna S, Hargadon B, Parker D, Bradding P, et al. Asthma exacerbations and sputum eosinophil counts: a randomised controlled trial. Lancet. 2002; 360:1715–1721. PMID: 12480423.


11. Johnston SL, Pattemore PK, Sanderson G, Smith S, Lampe F, Josephs L, et al. Community study of role of viral infections in exacerbations of asthma in 9–11 year old children. BMJ. 1995; 310:1225–1229. PMID: 7767192.


12. Grissell TV, Powell H, Shafren DR, Boyle MJ, Hensley MJ, Jones PD, et al. Interleukin-10 gene expression in acute virus-induced asthma. Am J Respir Crit Care Med. 2005; 172:433–439. PMID: 15894599.


13. Kim CK, Choi J, Callaway Z, Kim HB, Chung JY, Koh YY, et al. Clinical and epidemiological comparison of human metapneumovirus and respiratory syncytial virus in Seoul, Korea, 2003–2008. J Korean Med Sci. 2010; 25:342–347. PMID: 20191030.


14. Matsuse H, Kondo Y, Saeki S, Nakata H, Fukushima C, Mizuta Y, et al. Naturally occurring parainfluenza virus 3 infection in adults induces mild exacerbation of asthma associated with increased sputum concentrations of cysteinyl leukotrienes. Int Arch Allergy Immunol. 2005; 138:267–272. PMID: 16215328.


15. Kloepfer KM, Olenec JP, Lee WM, Liu G, Vrtis RF, Roberg KA, et al. Increased H1N1 infection rate in children with asthma. Am J Respir Crit Care Med. 2012; 185:1275–1279. PMID: 22366048.


16. Roh EJ, Jin YM, Chung EH, Rheem I, Kim JK. The prevalence of respiratory viral infection in exacerbation of asthma in hospitalized children. Korean J Asthma Allergy Clin Immunol. 2007; 27:241–247.


17. Chung JY, Han TH, Kim BE, Kim CK, Kim SW, Hwang ES. Human metapneumovirus infection in hospitalized children with acute respiratory disease in Korea. J Korean Med Sci. 2006; 21:838–842. PMID: 17043416.


18. Chung JY, Han TH, Kim SW, Kim CK, Hwang ES. Detection of viruses identified recently in children with acute wheezing. J Med Virol. 2007; 79:1238–1243. PMID: 17597481.


19. Foster CB, Friedman N, Carl J, Piedimonte G. Enterovirus D68: a clinically important respiratory enterovirus. Cleve Clin J Med. 2015; 82:26–31. PMID: 25552624.


20. Ralston SL, Lieberthal AS, Meissner HC, Alverson BK, Baley JE, Gadomski AM, et al. Clinical practice guideline: the diagnosis, management, and prevention of bronchiolitis. Pediatrics. 2014; 134:e1474–e1502. PMID: 25349312.


21. Lin JA, Madikians A. From bronchiolitis guideline to practice: a critical care perspective. World J Crit Care Med. 2015; 4:152–158. PMID: 26261767.


22. Henderson FW, Collier AM, Clyde WA Jr, Denny FW. Respiratorysyncytial-virus infections, reinfections and immunity. A prospective, longitudinal study in young children. N Engl J Med. 1979; 300:530–534. PMID: 763253.
23. Glezen WP, Taber LH, Frank AL, Kasel JA. Risk of primary infection and reinfection with respiratory syncytial virus. Am J Dis Child. 1986; 140:543–546. PMID: 3706232.


24. Carroll KN, Wu P, Gebretsadik T, Griffin MR, Dupont WD, Mitchel EF, et al. The severity-dependent relationship of infant bronchiolitis on the risk and morbidity of early childhood asthma. J Allergy Clin Immunol. 2009; 123:1055–1061. 1061.e1PMID: 19361850.


25. Jackson DJ, Gangnon RE, Evans MD, Roberg KA, Anderson EL, Pappas TE, et al. Wheezing rhinovirus illnesses in early life predict asthma development in high-risk children. Am J Respir Crit Care Med. 2008; 178:667–672. PMID: 18565953.


26. Calşkan M, Bochkov YA, Kreiner-Møller E, Bønnelykke K, Stein MM, Du G, et al. Rhinovirus wheezing illness and genetic risk of childhood-onset asthma. N Engl J Med. 2013; 368:1398–1407. PMID: 23534543.


27. Centers for Disease Control and Prevention (US). CDC develops a new, faster lab test for Enterovirus D68 [Internet]. Atlanta (GA): Centers for Disease Control and Prevention;2014. cited 2017 May 13. Available from: http://www.cdc.gov/media/releases/2014/p1014-test-enterovirus-d68.
28. McIntyre CL, Knowles NJ, Simmonds P. Proposals for the classification of human rhinovirus species A, B and C into genotypically assigned types. J Gen Virol. 2013; 94:1791–1806. PMID: 23677786.


29. Bochkov YA, Palmenberg AC, Lee WM, Rathe JA, Amineva SP, Sun X, et al. Molecular modeling, organ culture and reverse genetics for a newly identified human rhinovirus C. Nat Med. 2011; 17:627–632. PMID: 21483405.


30. Monto AS. The seasonality of rhinovirus infections and its implications for clinical recognition. Clin Ther. 2002; 24:1987–1997. PMID: 12581541.


31. Johnston NW, Johnston SL, Duncan JM, Greene JM, Kebadze T, Keith PK, et al. The September epidemic of asthma exacerbations in children: a search for etiology. J Allergy Clin Immunol. 2005; 115:132–138. PMID: 15637559.


32. Gern JE, Galagan DM, Jarjour NN, Dick EC, Busse WW. Detection of rhinovirus RNA in lower airway cells during experimentally induced infection. Am J Respir Crit Care Med. 1997; 155:1159–1161. PMID: 9117003.


33. Kim JH, Kim YS, Cho GS, Kim NH, Gong CH, Lee BJ, et al. Human rhinovirus-induced proinflammatory cytokine and Interferon-β responses in nasal epithelial cells from chronic rhinosinusitis patients. Allergy Asthma Immunol Res. 2015; 7:489–496. PMID: 26122508.


34. Handzel ZT, Busse WW, Sedgwick JB, Vrtis R, Lee WM, Kelly EA, et al. Eosinophils bind rhinovirus and activate virus-specific T cells. J Immunol. 1998; 160:1279–1284. PMID: 9570544.
35. Rosenberg HF, Domachowske JB. Eosinophils, eosinophil ribonucleases, and their role in host defense against respiratory virus pathogens. J Leukoc Biol. 2001; 70:691–698. PMID: 11698487.
36. Chihara J, Urayama O, Tsuda A, Kakazu T, Higashimoto I, Yamada H. Eosinophil cationic protein induces insulin-like growth factor I receptor expression on bronchial epithelial cells. Int Arch Allergy Immunol. 1996; 111(Suppl 1):43–45. PMID: 8906112.


37. Papi A, Johnston SL. Rhinovirus infection induces expression of its own receptor intercellular adhesion molecule 1 (ICAM-1) via increased NF-kappaB-mediated transcription. J Biol Chem. 1999; 274:9707–9720. PMID: 10092659.
38. Bønnelykke K, Sleiman P, Nielsen K, Kreiner-Møller E, Mercader JM, Belgrave D, et al. A genome-wide association study identifies CDHR3 as a susceptibility locus for early childhood asthma with severe exacerbations. Nat Genet. 2014; 46:51–55. PMID: 24241537.


39. Papadopoulos NG, Gourgiotis D, Javadyan A, Bossios A, Kallergi K, Psarras S, et al. Does respiratory syncytial virus subtype influences the severity of acute bronchiolitis in hospitalized infants? Respir Med. 2004; 98:879–882. PMID: 15338801.


40. Oliveira TF, Freitas GR, Ribeiro LZ, Yokosawa J, Siqueira MM, Portes SA, et al. Prevalence and clinical aspects of respiratory syncytial virus A and B groups in children seen at Hospital de Clínicas of Uberlândia, MG, Brazil. Mem Inst Oswaldo Cruz. 2008; 103:417–422. PMID: 18797752.


41. Liu AH, Covar RA, Spahn JD, Leung DY. Childhood asthma. In : Kliegman RM, Behrman RE, Jenson HB, Stanton BF, editors. Nelson textbook of pediatrics. 18th ed. Philadelphia (PA): Saunders Elsevier;2007. p. 953–970.
42. Peebles RS Jr, Graham BS. Pathogenesis of respiratory syncytial virus infection in the murine model. Proc Am Thorac Soc. 2005; 2:110–115. PMID: 16113477.


43. Welliver RC, Garofalo RP, Ogra PL. Beta-chemokines, but neither T helper type 1 nor T helper type 2 cytokines, correlate with severity of illness during respiratory syncytial virus infection. Pediatr Infect Dis J. 2002; 21:457–461. PMID: 12150192.


44. Openshaw PJ, Tregoning JS. Immune responses and disease enhancement during respiratory syncytial virus infection. Clin Microbiol Rev. 2005; 18:541–555. PMID: 16020689.


45. Matsuse H, Tsuchida T, Fukahori S, Kawano T, Tomari S, Matsuo N, et al. Differential airway inflammatory responses in asthma exacerbations induced by respiratory syncytial virus and influenza virus a. Int Arch Allergy Immunol. 2013; 161:378–382. PMID: 23689185.


46. Noah TL, Becker S. Chemokines in nasal secretions of normal adults experimentally infected with respiratory syncytial virus. Clin Immunol. 2000; 97:43–49. PMID: 10998316.


47. Haeberle HA, Kuziel WA, Dieterich HJ, Casola A, Gatalica Z, Garofalo RP. Inducible expression of inflammatory chemokines in respiratory syncytial virus-infected mice: role of MIP-1α in lung pathology. J Virol. 2001; 75:878–890. PMID: 11134301.


48. Borish L, Rosenwasser LJ. Cytokines in allergic inflammation. In : Adkinson NF, Yunginger JW, Busse WW, Bochner BS, Holgate ST, Simons FE, editors. Middleton's allergy principles & practice. 6th ed. St. Louis (MO): Mosby;2003. p. 135–157.
49. Kim CK, Kita H, Callaway Z, Kim HB, Choi J, Fujisawa T, et al. The roles of a Th2 cytokine and CC chemokine in children with stable asthma: potential implication in eosinophil degranulation. Pediatr Allergy Immunol. 2010; 21:e697–e704. PMID: 20444156.


50. Hull J, Rowlands K, Lockhart E, Moore C, Sharland M, Kwiatkowski D. Variants of the chemokine receptor CCR5 are associated with severe bronchiolitis caused by respiratory syncytial virus. J Infect Dis. 2003; 188:904–907. PMID: 12964123.


51. Hoebee B, Bont L, Rietveld E, van Oosten M, Hodemaekers HM, Nagelkerke NJ, et al. Influence of promoter variants of interleukin-10, interleukin-9, and tumor necrosis factor-alpha genes on respiratory syncytial virus bronchiolitis. J Infect Dis. 2004; 189:239–247. PMID: 14722888.
52. Meissner C, Storch GA. Picornaviruses: polio virus, other enteroviruses, and the rhinoviruses. In : Schaechter M, Engleberg NC, Eisenstein BI, Medoff G, editors. Mechanisms of microbial disease. 3rd ed. Philadelphia (PA): Lippincott Williams & Wilkins;1999. p. 305–314.
53. Smura T, Ylipaasto P, Klemola P, Kaijalainen S, Kyllönen L, Sordi V, et al. Cellular tropism of human enterovirus D species serotypes EV-94, EV-70, and EV-68 in vitro: implications for pathogenesis. J Med Virol. 2010; 82:1940–1949. PMID: 20872722.
54. Imamura T, Okamoto M, Nakakita S, Suzuki A, Saito M, Tamaki R, et al. Antigenic and receptor binding properties of enterovirus 68. J Virol. 2014; 88:2374–2384. PMID: 24371050.


55. Karnauchow TM, Tolson DL, Harrison BA, Altman E, Lublin DM, Dimock K. The HeLa cell receptor for enterovirus 70 is decay-accelerating factor (CD55). J Virol. 1996; 70:5143–5152. PMID: 8764022.


56. Soto-Quiros M, Avila L, Platts-Mills TA, Hunt JF, Erdman DD, Carper H, et al. High titers of IgE antibody to dust mite allergen and risk for wheezing among asthmatic children infected with rhinovirus. J Allergy Clin Immunol. 2012; 129:1499–1505.e5. PMID: 22560151.


57. Gern JE, Vrtis R, Grindle KA, Swenson C, Busse WW. Relationship of upper and lower airway cytokines to outcome of experimental rhinovirus infection. Am J Respir Crit Care Med. 2000; 162:2226–2231. PMID: 11112143.


58. Parry DE, Busse WW, Sukow KA, Dick CR, Swenson C, Gern JE. Rhinovirus-induced PBMC responses and outcome of experimental infection in allergic subjects. J Allergy Clin Immunol. 2000; 105:692–698. PMID: 10756217.


59. Papadopoulos NG, Stanciu LA, Papi A, Holgate ST, Johnston SL. Rhinovirus-induced alterations on peripheral blood mononuclear cell phenotype and costimulatory molecule expression in normal and atopic asthmatic subjects. Clin Exp Allergy. 2002; 32:537–542. PMID: 11972599.


60. Wark PA, Johnston SL, Bucchieri F, Powell R, Puddicombe S, Laza-Stanca V, et al. Asthmatic bronchial epithelial cells have a deficient innate immune response to infection with rhinovirus. J Exp Med. 2005; 201:937–947. PMID: 15781584.


61. Busse WW, Morgan WJ, Gergen PJ, Mitchell HE, Gern JE, Liu AH, et al. Randomized trial of omalizumab (anti-IgE) for asthma in innercity children. N Engl J Med. 2011; 364:1005–1015. PMID: 21410369.


62. Peltola V, Heikkinen T, Ruuskanen O, Jartti T, Hovi T, Kilpi T, et al. Temporal association between rhinovirus circulation in the community and invasive pneumococcal disease in children. Pediatr Infect Dis J. 2011; 30:456–461. PMID: 21200362.


63. Harford CG, Leidler V, Hara M. Effect of the lesion due to influenza virus on the resistance of mice to inhaled pneumococci. J Exp Med. 1949; 89:53–68. PMID: 18099165.


64. Cardenas PA, Cooper PJ, Cox MJ, Chico M, Arias C, Moffatt MF, et al. Upper airways microbiota in antibiotic-naïve wheezing and healthy infants from the tropics of rural Ecuador. PLoS One. 2012; 7:e46803. PMID: 23071640.


65. Bisgaard H, Hermansen MN, Buchvald F, Loland L, Halkjaer LB, Bønnelykke K, et al. Childhood asthma after bacterial colonization of the airway in neonates. N Engl J Med. 2007; 357:1487–1495. PMID: 17928596.


66. Gern JE. Viral respiratory infection and the link to asthma. Pediatr Infect Dis J. 2004; 23:S78–S86. PMID: 14730274.


67. Kloepfer KM, Sarsani VK, Poroyko V, Lee WM, Pappas TE, Kang T, et al. Community-acquired rhinovirus infection is associated with changes in the airway microbiome. J Allergy Clin Immunol. 2017; 140:312–315.e8. PMID: 28327377.


68. Stokholm J, Chawes BL, Vissing NH, Bjarnadóttir E, Pedersen TM, Vinding RK, et al. Azithromycin for episodes with asthma-like symptoms in young children aged 1–3 years: a randomised, double-blind, placebo-controlled trial. Lancet Respir Med. 2016; 4:19–26. PMID: 26704020.


Figure
Factors that can influence the severity of RV infections and the risk of RV-induced exacerbations of asthma. In children and adults with asthma, the severity of RV infection is positively related to the probability of exacerbation. Factors that increase the likelihood of more severe RV illnesses and exacerbations of asthma include the species of RV, the presence of bacterial pathogens in the airway, allergic inflammation, and polymorphisms in CDHR3 and the 17q21 region of the genome. RV, rhinovirus; CDHR3, cadherin-related protein-3; IgE, immunoglobulin E; Eos, eosinophils.
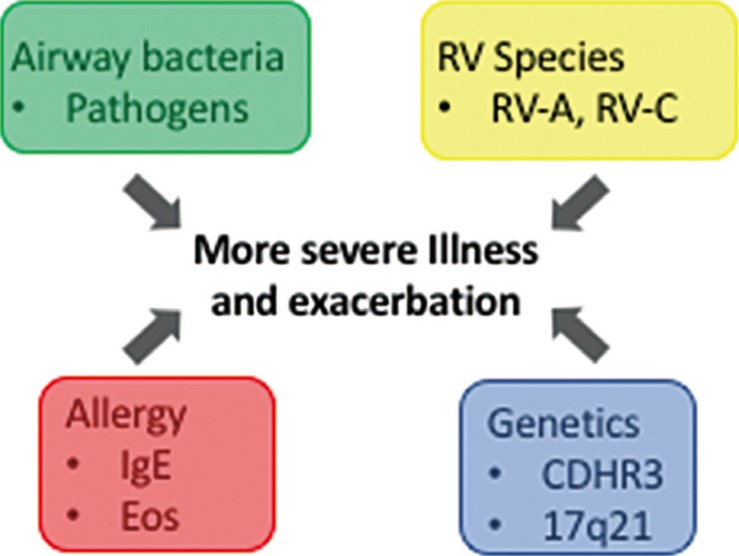