Abstract
Figures and Tables
FIG. 1
Algorithm of patient selection in our study. ECMO: extracorporeal membrane oxygenation, ECPR: extracorporeal cardiopulmonary resuscitation, VA: veno-arterial, VAV: veno-arterial venous, VV: veno-venous, PCT: procalcitonin.
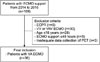
FIG. 2
Trends of Procalcitonin levels during ECMO support for 28dyas, presented as mean value±standard deviation. Peak value was reached within 48 hours after initiation of ECMO support (mean 25.6±9.4 ng/mL), and decreased to ≤5 ng/mL within 1 week. Mean value of PCT levels after first week varied in diverse patterns, but did not show the high levels shown for the first week. ECMO: extracorporeal membrane oxygenation, PCT: procalcitonin.

FIG. 3
Comparison between mean PCT levels within 7 days in survivors and non-survivors. Serial progression of mean PCT level was observed during the first week of ECMO support; the mean level was significantly higher over time in the survivor group (p=0.03). ECMO: extracorporeal membrane oxygenation, PCT: procalcitonin.
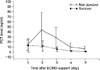
FIG. 4
Kaplan-Meier survival analysis: Peak PCT level ≥10 ng/mL in survivors and non-survivors. PCT level ≥10 ng/mL within the first week of ECMO support was significantly associated with survival probability (p=0.01). ECMO: extracorporeal membrane oxygenation, PCT: procalcitonin.
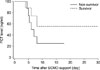
TABLE 1
Patients' characteristics before ECMO support
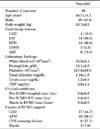
AFM: acute fulminant myocarditis, ECMO: extracorporeal membrane oxygenation, CAD: coronary artery disease, CPB: cardiopulmonary bypass, DM: diabetus mellitus, HTN: hypertension, COPD: chronic obstructive lung disease, AKI: acute kidney injury, CRP: C-reactive protein, ICU: intensive care unit. aData are presented as the number of cases (%) for categorical variables or as means±standard deviations for continuous variables.
TABLE 3
Univariate analysis of risk factors for nosocomial infection before and during ECMO support
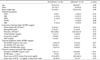
ECMO: extracorporeal membrane oxygenation, CAD: coronary artery disease, DM: diabetus mellitus, HTN: hypertension, COPD: chronic obstructive lung disease, AKI: acute kidney injury, CRP: C-reactive protein, ICU: intensive care, PCT: procalcitonin. aData are presented as the number of cases (%) for categorical variables or as means±standard deviations for continuous variables.
TABLE 4
Univariate analysis of risk factors for survival before and during ECMO support

ECMO: extracorporeal membrane oxygenation, CAD: coronary artery disease, DM: diabetus mellitus, HTN: hypertension, COPD: chronic obstructive lung disease, AKI: acute kidney injury, CRP: C-reactive protein, ICU: intensive care, PCT: procalcitonin. aData are presented as the number of cases (%) for categorical variables or as means±standard deviations for continuous variables.
ACKNOWLEDGEMENTS
References





















