Abstract
The antidiabetic drug metformin has been found to have beneficial effects in various neurological disorders; however, the molecular mechanisms underlying these effects remain unclear. Here we report that metformin protects neuronal cells from quinolinic acid (QUIN)-induced excitotoxicity. For this, we pretreated N18D3 neuronal cells with metformin prior to QUIN for 24 h. We found that pretreating the cells with metformin significantly improved cell survival rate in a concentration-dependent manner and reduced apoptotic cell death, as revealed by a MTT assay and DAPI staining, respectively. Calcium imaging using fluo-4 showed that metformin (100 µM) inhibited the intracellular calcium increase that was induced by QUIN. In addition, mRNA expression of pro-apoptotic genes, p21 and Bax, was decreased and of anti-apoptotic genes, Bcl-2 and Bcl-xl, was increased with metformin treatment compared to QUIN-induced cells. The immunoreactivity of phosphorylated ERK1/2 was elevated in cells treated with metformin, indicating the ERK1/2 signaling pathway in the neuroprotective effects of metformin in QUIN-induced cell death. Collectively, our data demonstrates that metformin exerts its neuroprotective effects by inhibiting intracellular calcium increases, allowing it to regulate ERK1/2 signaling and modulate cell survival and death genes.
Quinolinic acid (QUIN) is often implicated in the pathogenesis of several neurological disorders, including Alzheimer's disease, Parkinson's disease, multiple sclerosis, and amyotrophic lateral sclerosis.123 QUIN is considered as an endogenous neurotoxin because it induces excitotoxicity by activating N-methyl-D-aspartate (NMDA) receptors, increasing neuronal glutamate release, and inhibiting the reuptake of glutamate by astrocytes.4 NMDA receptors are known to play roles in excitotoxicity, as they can move large quantities of calcium into the cytoplasm. Increasing the intracellular calcium concentration initiates a cascade of apoptosis through caspase cleavages and following increased mitochondrial permeability.5
In the brain, QUIN is found in activated microglia and brain inflammation increases the QUIN levels in brain tissues and extracellular fluids.3 Proinflammatory mediators like interferon-γ, beta amyloid (Aβ), tumor necrosis factor α, and platelet activating factors reportedly induce QUIN overproduction within activated microglia.4 Therefore, QUIN toxicity has been widely used as a model of neurodegenerative disease.678
Metformin is a most common drug to treat hyperglycemia in patients with type 2 diabetes mellitus. Besides its hypoglycemic action metformin has beneficial effects in various central nervous system disorders. For example, metformin appears to protect the brain against cerebral ischemia, in which excitotoxicity is recognized as the primary mechanism of brain injury; these protective effects of metformin were observed regardless of whether it was applied in the acute stage or as a chronic treatment, likely through the pre-activation of autophagy or the phosphoinositide 3-kinase (PI3K)/c-Jun N-terminal kinase (JNK) signaling pathway.91011 Additionally, metformin preconditioning was found to protect neurons in spinal cord injuries by inhibiting apoptosis.12 In another study, metformin exhibited protective effects against cisplatin-induced neuronal cell death following decreasing cellular calcium levels and inhibiting the process of apoptosis.13
Metformin has also been found to increase damage to the nigrostriatal pathway in mice, even though it exhibits strong anti-inflammatory properties.14 Likewise, the effects of metformin in Alzheimer's disease are controversial. Metformin increases Aβ biogenesis15; however, it prevents the Alzheimer's disease pathology by ameliorating neuronal insulin resistance or attenuating long-term potentiation decreases in Aβ-induced toxicity.1617
Given the beneficial and adverse actions of metformin in neurological disorders, we investigated whether metformin protects neuronal cells from QUIN-induced excitotoxicity. In addition, our work revealed how metformin regulates intracellular calcium concentrations and mRNA expression levels of apoptosis-related genes.
N18D3 cells were used to perform the QUIN-induced excitotoxicity study as described previously.6 The cells were cultured in DMEM (Hyclone, Logan, UT, USA) with 10% FBS (Hyclone) and 1% penicillin-streptomycin (Hyclone) in 37℃ humidified incubator and grown to 80–90% confluency. We dissolved the QUIN (Sigma Chemical Co., St. Louis, MO, USA) and metformin hydrochloride (Sigma Chemical Co.) in fresh ddH2O and prepared them as stock solutions for use at different final concentrations.
To assess the cell viability in cultured N18D3 neurons, the 3-(4,5-dimethylthiazol-2-yl)-2,5-diphenyltetrazolium bromide (MTT; Sigma Chemical Co.) assay was performed in our previously published study.6 In brief, the cells (8×103 cells/well) were seeded in quadruplicate and then incubated with metformin (1, 10, 25, 50, 100 µM) (Sigma Chemical Co.) for 2 h prior to the addition of QUIN (30 mM). After 24 hours, the cells were exposed to 20 µL of MTT (5 mg/mL) and then incubated for 4 h at 37℃ in dark. The formazan precipitate was dissolved in 100 µL of DMSO (Sigma Chemical Co.) and the optical density was measured at 570 nm (Bio-Tek µQuant, ReTiSoft Inc., Ontario, Canada). These experiments were repeated four times independently.
To evaluate the nuclear morphology, DAPI (Sigma Chemical Co.) staining was accomplished according to our previously study's methodology,6 and then the cell nuclei were observed using a fluorescence microscope (Axio Vert. A1, Carl Zeiss, Germany). The number of apoptotic cells, defined DNA fragmentation, nuclear condensation, and segmentation, were identified in the 400 cells.
The concentration of intracellular calcium was measured with a cell-permeable calcium-sensitive fluorescent dye, fluo-4 AM (Thermo Fisher Scientific Inc., Waltham, MA, USA), based on our previous report.6 The N18D3 cells, which were cultured on cover-slips, were incubated with metformin (10 or 100 µM) for 2 h and then treated with QUIN (30 mM) for 24 h. The cells were loaded with fluo-4 AM (5 µM) in a balanced salt solution (BSS) for 1 h and then washed in BSS to eliminate the extracellular fluo-4 AM. The cover-slip with dye-loaded cells was observed on an inverted confocal microscope (Zeiss LSM 700, Carl Zeiss, Jena, Germany). Intracellular calcium is expressed as the relative fluo-4 AM fluorescence intensities (excited at 460–490 nm; emitted at 515–560 nm), and the intensity changes that occurred over time were monitored every 3 sec. Levels of intracellular calcium are described from eight independent experiments (≥50 cells).
Total RNA was isolated following the TriZol based protocol according to the manufacturer's instructions, and cDNA was synthesized using reverse-transcribed system (Takara Bio Inc., Shiga, Japan). The cDNA was amplified by 35 cycles of 94℃ for 2 min, appropriate annealing temperatures for each primer for 1 min, and 72℃ for 1 min. Selected primers were listed in Table 1, and the RT-PCR products were observed. Quantitative analysis was performed with the Image J software. The mRNA expression was normalized based on the level of ubiquitously expressed glyceraldehyde 3-phosphate dehydrogenase (GAPDH). Experiments were repeated five times independently.
The cells were cultured and prepared to perform a western blot assay. The phosphorylated and total forms of the enzymes such as ERK1/2, p38, and JNK were observed via western blot analyses, as described previously.18 Briefly, the cells were lysed in a lysis buffer and the protein concentration was determined with the Quant-iT™ assay kit (Molecular Probes, Eugene, OR, USA). The protein (15 mg) was loaded into a 10% sodium dodecyl sulfate-polyacrylamide gel electrophoresis and transferred to nitrocellulose membranes (Amersham Pharmacia Biotech, Buckinghamshire, UK). The membranes were incubated with specific antibodies for ERK1/2, p-ERK1/2, p38, p-p38, JNK, p-JNK, or GAPDH. Antibodies were purchased from Cell Signaling Technology (Danvers, MA, USA) and the dilution factor was 1:2000. The signals were detected by enhanced chemiluminescence reagents, and images were detected on ImageQuant LAS4000 (GE Healthcare, Piscataway, NJ, USA). The density was quantified using the Image J software. Experiments were repeated at least three times independently.
To evaluate the neuroprotective effects of metformin pretreatment on excitotoxicity, we performed MTT assay to conduct the cell viability and DAPI staining to detect DNA fragmentation and condensation. The viability of N18D3 neuronal cells was examined after metformin and/or QUIN treatment for 24 h. When cultured cells were exposed to 30 mM QUIN, the cell survival rate was 64.3±1.12% (#p<0.05). However, when the neuronal cells were co-incubated with different concentrations (1, 10, 25, 50, or 100 µM) of metformin prior to QUIN treatment, the percentage of survival cells increased significantly ranging from 64.9±1.21% at 1 µM to 88.9±2.54% (**p<0.01) at 100 µM in a concentration-dependent manner (Fig. 1A). We chose 10 µM and 100 µM metformin as representative experimental doses for further study based on MTT results. In parallel, less nuclear condensation and DNA fragmentation, characteristic features of apoptosis, were observed in metformin-pretreated cells while those features were highly increased in QUIN only treated ones (Fig. 1B). In addition, quantification data exhibited the decreased number of apoptotic cells (Fig. 1C). These results support the notion that metformin protects QUIN-induced neurotoxicity in N18D3 cells.
Since the QUIN-induced activation of NMDA receptors causes massive amounts of calcium to enter into neurons, we investigated the changes in intracellular calcium using Fluo-4 based calcium imaging experiments that occur in QUIN-induced excitotoxic neuronal death. As shown in Fig. 2, QUIN (30 mM) elevated the concentration of intracellular calcium in the cells, whereas pretreatment with 100 µM metformin significantly inhibited this rise in intracellular calcium (**p<0.01 at the peak concentrations). Treatment with 10 µM metformin did not significantly change the intracellular calcium concentrations. These findings indicate that metformin exerts its effects by modulating the changes in intracellular calcium that occur in QUIN-induced excitotoxicity.
To validate the neuroprotective action of metformin, mRNA analysis was performed using RT-PCR. We previously demonstrated that QUIN regulates mRNA expression through inducing pro-apoptotic genes and reducing anti-apoptotic genes.6 Here, we studied the action of metformin pretreatment in the expression of the apoptosis-related genes induced by QUIN in N18D3 neurons. We found that QUIN stimulated the expression of pro-apoptotic genes including those for p21 and Bax while pretreatment with metformin (10 or 100 µM) inhibited these gene expression elevations (Fig. 3). In contrast, QUIN inhibited the anti-apoptotic gene expression, such as Bcl-2 and Bclxl, whereas metformin pretreatment prevented this inhibition. Interestingly, the anti-apoptotic gene expression increased in the metformin 100 µM alone group as compared with the control. These results imply that metformin has protective effects on a variety of apoptosis-related processes.
To determine the neuroprotective mechanisms of metformin, we investigated the levels of protein, especially mitogen-activated protein (MAP) kinase family, in QUIN-treated cells using western blotting. The MAP kinase family regulates diverse biological processes, including cell survival and apoptosis, and is comprised of three subfamilies, namely ERK, JNK, and p-38 MAP kinase.1920 As such, we examined the possible involvement of the ERK, JNK, and p38 MAP kinase pathways in the effect of metformin in excitotoxicity. Following the western blot analysis (Fig. 4), application of QUIN reduced the immunoreactivity of phosphorylated ERK1/2 (p-ERK1/2), whereas metformin pretreatment elevated the immunoreactivity of p-ERK1/2. These findings indicate that the metformin inhibits the QUIN-induced reductions and then elevates the p-ERK1/2 levels.
The present study demonstrated that the antidiabetic drug metformin exerted a neuroprotective effect on QUIN-induced excitotoxicity via two distinct mechanisms. First, metformin reduced the amount of intracellular calcium entering the cells during excitotoxic neuronal death. Second, metformin attenuated apoptotic processes, possibly via the ERK1/2 pathway. These results are consistent with other recent reports demonstrating that metformin protected cerebellar granule neurons against glutamate-induced neurotoxicity21 and protected PC12 cells against H2O2-induced oxidative stress through Akt/mammalian target of rapamycin restoration.22 In addition, the antidiabetic drug metformin was found to exert protective effects against apoptotic cell death in auditory cell lines.2324
It is known that QUIN stimulates NMDA receptors as well as glutamate and aspartate but remains longer in the synaptic cleft because quinolinate phosphoribosyl transferase, an enzyme that degrades QUIN into NAD+, is rapidly saturated by its metabolite. Therefore, QUIN can stimulate NMDA receptors longer than can glutamate, subsequently causing extensive neuronal damage through massive calcium influxes. This process, called excitotoxicity, results in neuronal cell death and is implicated in acute central nervous system damage and chronic neurodegenerative diseases.52526 Here, QUIN treatment significantly induced neuronal death and intracellular calcium increases. However, metformin pretreatment improved cell viability and dramatically inhibited the QUIN-induced elevations in the intracellular calcium concentration. These results support that metformin plays a role in limiting the rise in intracellular calcium that occurs during apoptotic processes. Our data are consistent with other studies demonstrating that metformin inhibited the intracellular calcium increases in ethanol-induced apoptotic neurodegeneration and cisplatin-induced ototoxicity.1324
In this study, we determined that metformin suppresses apoptosis, as revealed by the reduced pro-apoptotic gene expression (those for p21 and Bax), elevates anti-apoptotic gene expression (those for Bcl-2 and Bcl-xl), and reduces nuclear condensation and DNA fragmentation. These data agree with the findings of previous studies showing that metformin reduces the ethanol-induced increases in Bax and enhances the decrease in Bcl-2.24 Metformin also alleviates Aβ-induced apoptosis in hippocampal neurons and inhibits the caspases expression that are activated by doxorubicin in cardiomyocytes.27
In our results, QUIN reduced immunoreactivity of p-ERK1/2 proteins, whereas metformin pretreatment attenuated these QUIN-induced reductions. Generally, the activation of ERK1/2 is known to inhibit apoptosis and promote cell survival.2028 Previously, it was reported that the ERK acts as a key modulator in the protective action of bis(propyl)-cognitin or interleukin-6 in excitatory amino acid-induced neuronal excitotoxicity.2930 Here, metformin pretreatment elevated not only ERK1/2 protein phosphorylation but also Bcl-2 and Bcl-xl gene expression, which is consistent with previous reports.
Taken together, this study elucidates the molecular mechanisms underlying metformin's protective effects against QUIN-induced neuronal excitotoxicity including reducing intracellular calcium increases, modulating cell survival and death genes, and activating the ERK1/2 signaling pathway. These results imply that metformin regulates the apoptosis-related genes and downstream MAP kinase mechanisms to protect fromcell death and has the potential to serve as a treatment for excitotoxicity-mediated diseases.
Figures and Tables
FIG. 1
Effects of metformin in QUIN-induced excitotoxicity. (A) We treated metformin (1, 10, 25, 50, or 100 µM) for 2 h prior to exposing them to QUIN (30 mM) for 24 h in N18D3 cells. Pretreatment with metformin improved the cell viability. (B) DAPI staining showed the condensation and fragmentation, which characterized features of apoptosis. Untreated cells (control) and those treated with metformin alone displayed a round or oval shape. The treatment of QUIN (30 mM) caused apoptotic-like cell, whereas, metformin pretreatment prevented such changes from occurring. Scale bar indicates 20 µm. (C) Apoptotic cell population was reduced by metformin pretreatment. *p<0.05 and **p<0.01 compared with QUIN (30 mM)-treated cells; #p<0.05 compared with control.
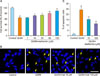
FIG. 2
Changes in intracellular calcium ([Ca2+]i) induced by QUIN or metformin. (A) The [Ca2+]i were elevated with QUIN (30 mM) alone and reduced by pretreatment with metformin (100 µM). Scale bar indicates 20 µm. (B) Time course curve of fluo-4 fluorescence intensity demonstrated that metformin (100 µM) significantly blocked the QUIN-induced elevations in [Ca2+]i. **p<0.01 compared with QUIN-treated cells.
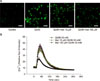
FIG. 3
Modulation of anti-apoptotic and pro-apoptotic genes by QUIN and metformin. (A) The cells were incubated with QUIN (30 mM) and/or metformin (10 or 100 µM) for 6 h. Cellular mRNA expression for p21, Bax, Bcl-2, and Bcl-xl was analyzed using RT-PCR. The pro-apoptotic gene expression was reduced by metformin pretreatment, whereas the expression of anti-apoptotic genes was elevated. (B) The expression level of each gene was normalized to GAPDH. *p<0.05 and **p<0.01 compared with QUIN-treated cells; #p<0.05 compared with control.
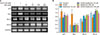
FIG. 4
Expression of MAP kinase signaling pathway. (A) To evaluate the MAP kinase expression, western blot analysis was performed following treatment of metformin (10 or 100 µM) and/or QUIN (30 mM) for 6 h in N18D3 cells. The JNK, ERK1/2, and p38 MAP kinase proteins were investigated and the phosphorylated ERK1/2 was increased. (B–D) The expression level of each gene was normalized to GAPDH. *p<0.05 compared with QUIN-treated cells; #p<0.05 compared with control.
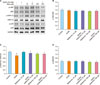
ACKNOWLEDGEMENTS
This study was supported by the Basic Science Research Program through the National Research Foundation of Korea (NRF) funded by the Ministry of Education (2016R1A6A3A11936076).
References
1. Busse M, Hettler V, Fischer V, Mawrin C, Hartig R, Dobrowolny H, et al. Increased quinolinic acid in peripheral mononuclear cells in Alzheimer's dementia. Eur Arch Psychiatry Clin Neurosci. 2017; DOI: 10.1007/s00406-017-0785-y. [Epub ahead of print].


2. Breda C, Sathyasaikumar KV, Sograte Idrissi S, Notarangelo FM, Estranero JG, Moore GG, et al. Tryptophan-2,3-dioxygenase (TDO) inhibition ameliorates neurodegeneration by modulation of kynurenine pathway metabolites. Proc Natl Acad Sci U S A. 2016; 113:5435–5440.


3. Lugo-Huitrón R, Ugalde Muñiz P, Pineda B, Pedraza-Chaverrí J, Ríos C, Pérez-de la. Quinolinic acid: an endogenous neurotoxin with multiple targets. Oxid Med Cell Longev. 2013; 2013:104024.


5. Kritis AA, Stamoula EG, Paniskaki KA, Vavilis TD. Researching glutamate - induced cytotoxicity in different cell lines: a comparative/ collective analysis/study. Front Cell Neurosci. 2015; 9:91.


6. Jang S, Jeong HS, Park JS, Kim YS, Jin CY, Seol MB, et al. Neuroprotective effects of (-)-epigallocatechin-3-gallate against quinolinic acid-induced excitotoxicity via PI3K pathway and NO inhibition. Brain Res. 2010; 1313:25–33.


7. Latif-Hernandez A, Shah D, Ahmed T, Lo AC, Callaerts-Vegh Z, Van der Linden A, et al. Quinolinic acid injection in mouse medial prefrontal cortex affects reversal learning abilities, cortical connectivity and hippocampal synaptic plasticity. Sci Rep. 2016; 6:36489.


8. Mishra J, Chaudhary T, Kumar A. Rosiglitazone synergizes the neuroprotective effects of valproic acid against quinolinic acid-induced neurotoxicity in rats: targeting PPARγ and HDAC pathways. Neurotox Res. 2014; 26:130–151.


9. Jiang T, Yu JT, Zhu XC, Wang HF, Tan MS, Cao L, et al. Acute metformin preconditioning confers neuroprotection against focal cerebral ischaemia by pre-activation of AMPK-dependent autophagy. Br J Pharmacol. 2014; 171:3146–3157.


10. Venna VR, Li J, Hammond MD, Mancini NS, McCullough LD. Chronic metformin treatment improves post-stroke angiogenesis and recovery after experimental stroke. Eur J Neurosci. 2014; 39:2129–2138.


11. Ge XH, Zhu GJ, Geng DQ, Zhang HZ, He JM, Guo AZ, et al. Metformin protects the brain against ischemia/reperfusion injury through PI3K/Akt1/JNK3 signaling pathways in rats. Physiol Behav. 2017; 170:115–123.


12. Wang C, Liu C, Gao K, Zhao H, Zhou Z, Shen Z, et al. Metformin preconditioning provide neuroprotection through enhancement of autophagy and suppression of inflammation and apoptosis after spinal cord injury. Biochem Biophys Res Commun. 2016; 477:534–540.


13. Chang J, Jung HH, Yang JY, Lee S, Choi J, Im GJ, et al. Protective effect of metformin against cisplatin-induced ototoxicity in an auditory cell line. J Assoc Res Otolaryngol. 2014; 15:149–158.


14. Ismaiel AA, Espinosa-Oliva AM, Santiago M, García-Quintanilla A, Oliva-Martín MJ, Herrera AJ, et al. Metformin, besides exhibiting strong in vivo anti-inflammatory properties, increases mptp-induced damage to the nigrostriatal dopaminergic system. Toxicol Appl Pharmacol. 2016; 298:19–30.


15. Chen Y, Zhou K, Wang R, Liu Y, Kwak YD, Ma T, et al. Antidiabetic drug metformin (GlucophageR) increases biogenesis of Alzheimer's amyloid peptides via up-regulating BACE1 transcription. Proc Natl Acad Sci U S A. 2009; 106:3907–3912.


16. Gupta A, Bisht B, Dey CS. Peripheral insulin-sensitizer drug metformin ameliorates neuronal insulin resistance and Alzheimer's-like changes. Neuropharmacology. 2011; 60:910–920.


17. Asadbegi M, Yaghmaei P, Salehi I, Ebrahim-Habibi A, Komaki A. Neuroprotective effects of metformin against Aβ-mediated inhibition of long-term potentiation in rats fed a high-fat diet. Brain Res Bull. 2016; 121:178–185.


18. Jang S, Park JS, Jeong HS. Neural differentiation of human adipose tissue-derived stem cells involves activation of the Wnt5a/JNK signalling. Stem Cells Int. 2015; 2015:178618.


19. Xia Z, Dickens M, Raingeaud J, Davis RJ, Greenberg ME. Opposing effects of ERK and JNK-p38 MAP kinases on apoptosis. Science. 1995; 270:1326–1331.


21. Zhou C, Sun R, Zhuang S, Sun C, Jiang Y, Cui Y, et al. Metformin prevents cerebellar granule neurons against glutamate-induced neurotoxicity. Brain Res Bull. 2016; 121:241–245.


22. Khallaghi B, Safarian F, Nasoohi S, Ahmadiani A, Dargahi L. Metformin-induced protection against oxidative stress is associated with AKT/mTOR restoration in PC12 cells. Life Sci. 2016; 148:286–292.


23. Chang J, Jung HH, Yang JY, Choi J, Im GJ, Chae SW. Protective role of antidiabetic drug metformin against gentamicin induced apoptosis in auditory cell line. Hear Res. 2011; 282:92–96.


24. Ullah I, Ullah N, Naseer MI, Lee HY, Kim MO. Neuroprotection with metformin and thymoquinone against ethanol-induced apoptotic neurodegeneration in prenatal rat cortical neurons. BMC Neurosci. 2012; 13:11.


25. Rahman A, Ting K, Cullen KM, Braidy N, Brew BJ, Guillemin GJ. The excitotoxin quinolinic acid induces tau phosphorylation in human neurons. PLoS One. 2009; 4:e6344.


26. Lai TW, Zhang S, Wang YT. Excitotoxicity and stroke: identifying novel targets for neuroprotection. Prog Neurobiol. 2014; 115:157–188.


27. Chen B, Teng Y, Zhang X, Lv X, Yin Y. Metformin alleviated Aβ-induced apoptosis via the suppression of JNK MAPK signaling pathway in cultured hippocampal neurons. Biomed Res Int. 2016; 2016:1421430.


28. Li Q, Chen M, Liu H, Yang L, Yang T, He G. The dual role of ERK signaling in the apoptosis of neurons. Front Biosci (Landmark Ed). 2014; 19:1411–1417.

