Abstract
Cardiovascular disease remains the leading cause of death worldwide and regenerative medicine is a promising therapeutic option for this disease. We have developed various techniques to attenuate the cardiac remodeling and to regenerate cardiovascular systems via stem cell application. Besides cell therapy, we are interested in the modulation of pathological inflammation mediated by macrophages in the damaged heart tissue to arouse endogenous reparative responses with biocompatible small molecules. Certainly, current understanding of mechanisms of tissue regeneration will lead to the development of innovative regenerative medicine for cardiovascular disease.
Cardiovascular disease remains the leading cause of death. Advances in pharmacological management have resulted in an increasing proportion of patients surviving after acute myocardial infarction (MI). Unfortunately, this decrease in early mortality has resulted in an increased incidence of chronic heart failure (HF) in the survivors. HF is the consequence of cardiomyocyte death or dysfunction, which is most commonly caused by MI. Over the past decades, HF remains common, and the incidence rate has been dramatically increased to almost 300% despite strides forward in the management of acute MI (Fig. 1, Statistics Korea, 2016). More importantly, morbidity of HF after MI and relapse have consistently increased, and recent demographics show the mortality of acute HF has reached 44% within 3 years of discharge (Korean acute HF registry, Korea Centers for Disease Control & Prevention). South Korea is one of the most rapidly aging societies and the prevalence of HF increases with population aging. Current therapies to prevent or delay progression of HF are limited. Conventional pharmacotherapy targets the maladaptive counter-regulatory mechanism activated by left ventricular dysfunction, and this approach has yielded blockbuster agents. Although these drugs reduce mortality, they fail to address the underlying loss of cardiomyocytes and vasculature, and are intrinsically non-curative. That is why we need to better understand the biology and pathophysiology of cardiovascular disease with in-depth investigations.
The regenerative capacity of the adult heart is limited and insufficient to overcome the massive loss of cardiomyocytes during acute damage or prolonged remodeling, in which cardiomyocyte death exceeds cardiomyocyte renewal. Over the past decade, cell therapy has been developed as a means to augment myocyte numbers and to improve cardiac function after MI. Stem cells have been identified as a regeneration therapy for cardiovascular disease. Myoblasts, embryonic stem cells (ESC), induced pluripotent stem cells (iPSC), or mesenchymal stem cells (MSCs) have been developed for regeneration therapy. MSCs, a representative adult stem cell type, are widely used for tissue regeneration strategies in clinical studies with a good safety profile (Fig. 2).
Stem cells represent a cell population with self-renewal properties and the potential to generate daughter cells that are capable of differentiation along specific cell lineages.1 To date, several stem cell types have been considered for the treatment of patients with ischemic and nonischemic chronic HF. An overview of clinical studies according to stem cell types and patient selection is presented in Table 1. Regeneration of the damaged heart tissue is daunting, however, there have been issues considering the massive loss of cardiomyocyte and supporting cells have to be rescued in scarred myocardium. Stem cells usually induced cardiac repair mediated with angiogenesis, cardiomyocyte protection, and growth factor release. Although MSCs have been shown to be safe in preclinical studies, multiple meta-analyses have debated whether functional improvement is significant or not. To enhance the therapeutic efficacy of MSCs, various modifications have been shown successful results. Pro-survival enzyme Akt was largely contributed to increase cell survival and intracoronary infusion of Akt-overexpressed MSCs resulted in improved cardiac function in the porcine MI model.2 Priming umbilical cord blood-derived MSCs with oxytocin for 24 hours increased migration activity via metalloproteinase upregulation.3 Moreover, cultured umbilical cord blood-derived MSCs with oxytocin for 7 days showed considerable cardiac differentiation in the infarcted myocardium.4 In a recent study, apicidin, an inhibitor of histone deacetylase, highly induced cardiac genes in MSCs (Fig. 3), and a combination application with naïve MSCs and apicidin-treated MSCs showed both increased cardiomyogenic differentiation and angiogenesis.5
Throughout the continuous trials, we found that the delivery route for stem cell transplantation is the critical factor that determines the therapeutic efficacy of cell therapy. Unlike in Europe and United States, endocardial injection is not clinically approved in Korea. To validate the accessibility of endocardial injection of stem cells into infarcted myocardium, we established the protocol of left ventricular mapping and endocardial injection in a porcine MI model. MSCs were injected into the peri-infarct zone by using MyoSar® (Biosense Webster, USA) connected to a mapping system (NOGA® XP, Biosense Webster, USA), and found that endocardial injection of MSCs was safe and effective to improve cardiac function (Fig. 4).6
Besides MSCs, intracoronary infusion of cardiospherederived cells (CDCs), the heart tissue-derived cells, showed the cardioprotective regeneration in preclinical and clinical studies. The therapeutic effects of CDCs were known to be mediated mainly by paracrine factors such as microRNAs and exosomes (reviewed in7).
Macrophages are heterogeneous and actively involved in the inflammatory response during the progression of cardiac injury.8 After MI, circulating monocytes are rapidly recruited to the infarcted myocardium to participate in clearance of debris, angiogenesis, and tissue regeneration (Fig. 5).
Most tissue contains resident macrophages that can self-renew independent of blood monocytes.9 However, some tissue macrophages do require continuous turnover from monocytes.10 Resident tissue macrophages are thought to arise from early yolk sac-derived progenitor cells,11 bypassing monocytes, or from fetal liver monocytes that themselves have derived from erythro-myeloid progenitors.1213 When looking at monocyte subsets in a mouse in the first days post MI the classical monocytes were shown to migrate into the infarct while non-classical monocytes infiltrated the tissue beginning on day 5 post MI. In this model the classical and non-classical subsets were shown to go along with inflammatory and reparative functions, respectively.814 Canonically, classically activated M1 type macrophages, expressing the integrin CD11c, are proinflammatory, whereas alternatively activated M2 type macrophages, which do not express CD11c, are anti-inflammatory.15 In human blood the classical monocytes (CD14++CD16−) peaked on day 3 and decreased thereafter while non-classical monocytes (CD14+CD16+) peaked on day 5 and remained high.8 MI patients with ventricular thrombus formation showed lower non-classical monocytes in the peripheral blood, and this finding suggested a protective role of non-classical monocytes in the progress of cardiac pathology.16 Taken together while both monocyte subsets are required for the post MI repair process, it appears that classical monocytes may be detrimental and non-classical may be beneficial when it comes to repair and long term organ function. The role of intermediate monocytes in this process remains to be determined.
In atherogenesis, monocyte extravasation into the vascular wall as well as the response of tissue-resident macrophages to modified lipoproteins can stimulate a cytokine response contributing to further leukocyte recruitment and the generation of lipid-laden foam cells, described as macrophages.1718 Macrophage foam cells within the plaque contribute to an ongoing inflammatory response, with resulting continued recruitment of other immune cells into the plaque.1920
In infarcted heart tissue, monocyte response is temporally biphasic. Pro-inflammatory monocytes are abundant in the early phase (on days 1-4) and remove cell debris, while reparative monocytes dominate in the late phase to lead the resolution of inflammation.8 However this bipolar concept, predominantly described through in vitro and murine gene knockout data, may have oversimplified the true nature of physiological differentiation in vivo where a more complex milieu contributes to final macrophage phenotype.212223 Acute MI patients showed a biphasic monocyte response in a cohort study. Circulating inflammatory monocytes expanded in early phase, followed by anti-inflammatory monocytes.24 These results are in line with studies in mice, but additional studies are needed to determine the monocyte response in the heart tissues.
In addition to local inflammation, monocytes and macrophages are required for MSCs to exert cardioprotective effects. Without monocytes and macrophages, there was no significant cardiac recovery by MSCs injection.25 Interestingly, the inflammatory phenotype of macrophages was substantially suppressed, while anti-inflammatory mediators were markedly upregulated by MSCs.26
A number of studies showed that perturbations in the macrophage-related inflammatory response are known to impair cardiac recovery. Lack of microsomal prostaglandin E2 synthase-1 in bone marrow-derived leukocytes negatively regulates cyclo-oxygenase-1 expression, prostaglandin E2 biosynthesis, and inflammation in the infarct and leads to impaired cardiac function, adverse left ventricular remodeling, and decreased survival after MI.27 Circulating monocytes orchestrate both inflammatory and reparative phases during MI and depend on Nr4a1, a nuclear receptor, to limit their influx and inflammatory cytokine expression. Nr4a1 modulated monocyte-dominant reparative phases to protect against cardiac remodeling in the infarcted myocardium.28
Dendritic cells are antigen-presenting cells that are responsible for adaptive immunity and immune homeostasis,29 and are identified by their origin with some common precursors or circulating monocytes that differentiate into dendritic cells in the peripheral tissue or atherosclerotic plaque.3031 A recent study demonstrated that the application of dendritic cells pretreated with infarcted cardiac lysate resulted in cardiac recovery through regulatory T cells-induced macrophage modulation.32
Neutrophils, the most abundant leukocyte in human peripheral blood, are proinflammatory phagocytes and are one of the first responders of inflammation. They rapidly release proteolytic enzymes to traverse through extracellular matrix to lesion, and this release plays a role in further recruitment and activation of other innate immune cells.33 Neutrophils infiltrate coronary plaques and the infarcted myocardium and mediate tissue damage by releasing matrix-degrading enzymes and reactive oxygen species, which are contributable to post-infarction adverse cardiac remodeling.34 Neutrophils are the first immune cell population to reach a peak within the first days after onset of ischemia, and are crucially involved in cardiac repair after MI by polarizing macrophages towards a reparative phenotype.35 Therapeutic strategies to reduce acute neutrophil-driven inflammation after MI should be carefully balanced as they might interfere with the healing response and cardiac remodeling.36
The cardiac microenvironment includes cardiomyocytes, fibroblasts and macrophages, which regulate remodeling and inflammation after MI. There have been numerous studies to pick out the most reliable candidates for novel drug development. Among them, we found 5-azacytidine (5AZ), a DNA methylation inhibitor, can ameliorate cardiac injury by shifting macrophages toward an anti-inflammatory phenotype through sumoylation of interferon regulatory factor-1 in cardiac macrophages.3738 Additionally, 5AZ upregulated cell survival signaling phosphorylated ERK, phosphorylated Akt, and bcl-2 in cardiomyocytes. On the other hand, pro-fibrotic connective tissue growth factor and collagen type I were downregulated in 5-AZ treated cardiac fibroblasts. Moreover, a natural compound derivative, 6-Bromoindirubin-3-oxime (BIO) modulated the cardiac microenvironment to exert a therapeutic effect.39 In activated macrophages, BIO inhibited the expression of pro-inflammatory factors. In the zebrafish and rat models, BIO induced cardiomyocyte proliferation, reduced fibrosis and improved cardiac performance. Histological analysis revealed modulation of the cardiac microenvironment by BIO, with increased presence of anti-inflammatory macrophages. Macrophages are known to participate in cardiac remodeling via local proliferation in the failing heart, and inflammatory cardiac macrophages lead to adverse remodeling.81040 In accordance with these studies, the paradigm shift in terms of target cells for cardiac regeneration from cardiomyocyte to cardiac immune cells offers new therapeutic options. For instance, it could be beneficial to modulate the ratio of subsets of infiltrated macrophages to ease chronic inflammation and tissue repair by application of small molecules such as 5-azacytidine and BIO.
Various strategies with stem cells and biocompatible chemical compounds for cardiac regeneration intensively developed and resulted in multiple effects on the major cell types comprising the cardiac microenvironment to induce the resolution of excessive inflammation and to improve cardiac performance (Fig. 6). From a different perspective, potential immune-targeted therapy can be motivated by increased knowledge of monocyte/macrophage biology. Strict assessment of the efficacy and adverse effects of these therapies will undoubtedly drive ongoing researches to complement cardiac regeneration for the prevention and treatment of cardiovascular disease.
Figures and Tables
FIG. 1
Prevalence of myocardial infarction and the incidence rate of heart failure in Korea. AMI: acute myocardial infarction, IHD: ischemic heart disease, HF: heart failure, yr: years, mo: months.
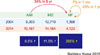
FIG. 3
(A) Representative images of immunocytofluorescence staining showed the cardiogenic MSCs expressed cardiac-specific proteins GATA4 (green) and cTnI (red). Nuclei were stained with DAPI (blue). (B) Treatment of MSCs with differentiation inducer upregulated cardiac marker expressions such as MEF2C, cTnI, and GATA4. MSCs: mesenchymal stem cells, MEF2C: myocyte enhancer factor 2c, cTnI: cardiac troponin I.
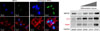
FIG. 4
Mapping images of left ventricle in a porcine myocardial infarction model. red: infarct zone, yellow: peri-infarct zone, green: viable myocardium. Stem cells are injected into the myocardium endocardially by using MYOSTAR injection catheter.
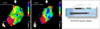
FIG. 5
Phenotype changes of infiltrated macrophages in the infarcted myocardium over time. M1 type represents pro-inflammatory macrophages, while M2 represents anti-inflammatory subtype. MI: myocardial infarction, iNOS: inducible nitric oxide synthase, IL-1β: interleukin-1β, TNF-α: tumor necrosis factor-α, Arg1: arginase 1.
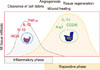
FIG. 6
Stem cells and small molecules such as 5-azacytidine and BIO modulate multiple aspects of the cardiac microenvironment to improve cardiac remodeling after injury. Cardiomyocytes are protected from cell death to provide a therapeutic opportunity to replace a portion of the dead cardiomyocytes, whereas proliferation of cardiac myofibroblasts was inhibited. Macrophage phenotype was shifted toward anti-inflammatory in the cardiac microenvironment.
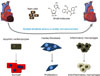
ACKNOWLEDGEMENTS
This research was supported by the National Research Foundation of Korea (NRF-2015M3A9B4066496, 2015-M3A9C6031684, 2016R1D1A1A09917796).
References
1. Blau HM, Brazelton TR, Weimann JM. The evolving concept of a stem cell: entity or function? Cell. 2001; 105:829–841.
2. Lim SY, Kim YS, Ahn Y, Jeong MH, Hong MH, Joo SY, et al. The effects of mesenchymal stem cells transduced with Akt in a porcine myocardial infarction model. Cardiovasc Res. 2006; 70:530–542.


3. Kim YS, Kwon JS, Hong MH, Kim J, Song CH, Jeong MH, et al. Promigratory activity of oxytocin on umbilical cord blood-derived mesenchymal stem cells. Artif Organs. 2010; 34:453–461.


4. Kim YS, Ahn Y, Kwon JS, Cho YK, Jeong MH, Cho JG, et al. Priming of mesenchymal stem cells with oxytocin enhances the cardiac repair in ischemia/reperfusion injury. Cells Tissues Organs. 2012; 195:428–442.


5. Cho DI, Kang WS, Hong MH, Kang HJ, Kim MR, Kim MC, et al. The optimization of cell therapy by combinational application with apicidin-treated mesenchymal stem cells after myocardial infarction. Oncotarget. 2017; 8:44281–44294.


6. Kim MC, Kim YS, Kang WS, Lee KH, Cho M, Hong MH, et al. Intramyocardial injection of stem Cells in pig myocardial infarction model: the first trial in Korea. J Korean Med Sci. 2017; 32:1708–1712.


8. Nahrendorf M, Swirski FK, Aikawa E, Stangenberg L, Wurdinger T, Figueiredo JL, et al. The healing myocardium sequentially mobilizes two monocyte subsets with divergent and complementary functions. J Exp Med. 2007; 204:3037–3047.


9. Takahashi K. Development and differentiation of macrophages and their related cells. Hum Cell. 1994; 7:109–115.
10. Yona S, Kim KW, Wolf Y, Mildner A, Varol D, Breker M, et al. Fate mapping reveals origins and dynamics of monocytes and tissue macrophages under homeostasis. Immunity. 2013; 38:79–91.


11. Epelman S, Lavine KJ, Beaudin AE, Sojka DK, Carrero JA, Calderon B, et al. Embryonic and adult-derived resident cardiac macrophages are maintained through distinct mechanisms at steady state and during inflammation. Immunity. 2014; 40:91–104.


12. Bain CC, Bravo-Blas A, Scott CL, Perdiguero EG, Geissmann F, Henri S, et al. Constant replenishment from circulating monocytes maintains the macrophage pool in the intestine of adult mice. Nat Immunol. 2014; 15:929–937.


13. Perdiguero EG, Geissmann F. The development and maintenance of resident macrophages. Nat Immunol. 2016; 17:2–8.


14. Dutta P, Sager HB, Stengel KR, Naxerova K, Courties G, Saez B, et al. Myocardial infarction activates CCR2(+) hematopoietic stem and progenitor cells. Cell Stem Cell. 2015; 16:477–487.


16. Frantz S, Hofmann U, Fraccarollo D, Schäfer A, Kranepuhl S, Hagedorn I, et al. Monocytes/macrophages prevent healing defects and left ventricular thrombus formation after myocardial infarction. FASEB J. 2013; 27:871–881.


17. Galis ZS, Sukhova GK, Kranzhöfer R, Clark S, Libby P. Macrophage foam cells from experimental atheroma constitutively produce matrix-degrading proteinases. Proc Natl Acad Sci U S A. 1995; 92:402–406.


18. Clinton SK, Underwood R, Hayes L, Sherman ML, Kufe DW, Libby P. Macrophage colony-stimulating factor gene expression in vascular cells and in experimental and human atherosclerosis. Am J Pathol. 1992; 140:301–316.
19. Randolph GJ. Emigration of monocyte-derived cells to lymph nodes during resolution of inflammation and its failure in atherosclerosis. Curr Opin Lipidol. 2008; 19:462–468.


20. Melián A, Geng YJ, Sukhova GK, Libby P, Porcelli SA. CD1 expression in human atherosclerosis. A potential mechanism for T cell activation by foam cells. Am J Pathol. 1999; 155:775–786.
22. Gordon S, Plüddemann A, Martinez Estrada F. Macrophage heterogeneity in tissues: phenotypic diversity and functions. Immunol Rev. 2014; 262:36–55.


24. Tsujioka H, Imanishi T, Ikejima H, Kuroi A, Takarada S, Tanimoto T, et al. Impact of heterogeneity of human peripheral blood monocyte subsets on myocardial salvage in patients with primary acute myocardial infarction. J Am Coll Cardiol. 2009; 54:130–138.


25. Ben-Mordechai T, Holbova R, Landa-Rouben N, Harel-Adar T, Feinberg MS, Abd Elrahman I, et al. Macrophage subpopulations are essential for infarct repair with and without stem cell therapy. J Am Coll Cardiol. 2013; 62:1890–1901.


26. Cho DI, Kim MR, Jeong HY, Jeong HC, Jeong MH, Yoon SH, et al. Mesenchymal stem cells reciprocally regulate the M1/M2 balance in mouse bone marrow-derived macrophages. Exp Mol Med. 2014; 46:e70.


27. Degousee N, Simpson J, Fazel S, Scholich K, Angoulvant D, Angioni C, et al. Lack of microsomal prostaglandin E(2) synthase-1 in bone marrow-derived myeloid cells impairs left ventricular function and increases mortality after acute myocardial infarction. Circulation. 2012; 125:2904–2913.


28. Hilgendorf I, Gerhardt LM, Tan TC, Winter C, Holderried TA, Chousterman BG, et al. Ly-6Chigh monocytes depend on Nr4a1 to balance both inflammatory and reparative phases in the infarcted myocardium. Circ Res. 2014; 114:1611–1622.


29. Cella M, Sallusto F, Lanzavecchia A. Origin, maturation and antigen presenting function of dendritic cells. Curr Opin Immunol. 1997; 9:10–16.


30. Swirski FK, Libby P, Aikawa E, Alcaide P, Luscinskas FW, Weissleder R, et al. Ly-6Chi monocytes dominate hypercholesterolemia-associated monocytosis and give rise to macrophages in atheromata. J Clin Invest. 2007; 117:195–205.


31. Chistiakov DA, Sobenin IA, Orekhov AN, Bobryshev YV. Myeloid dendritic cells: development, functions, and role in atherosclerotic inflammation. Immunobiology. 2015; 220:833–844.


32. Choo EH, Lee JH, Park EH, Park HE, Jung NC, Kim TH, et al. Infarcted Myocardium-primed dendritic cells improve remodeling and cardiac function after myocardial infarction by modulating the regulatory T cell and macrophage polarization. Circulation. 2017; 135:1444–1457.


33. Soehnlein O, Weber C, Lindbom L. Neutrophil granule proteins tune monocytic cell function. Trends Immunol. 2009; 30:538–546.


34. Carbone F, Nencioni A, Mach F, Vuilleumier N, Montecucco F. Pathophysiological role of neutrophils in acute myocardial infarction. Thromb Haemost. 2013; 110:501–514.


35. Jung K, Kim P, Leuschner F, Gorbatov R, Kim JK, Ueno T, et al. Endoscopic time-lapse imaging of immune cells in infarcted mouse hearts. Circ Res. 2013; 112:891–899.


36. Horckmans M, Ring L, Duchene J, Santovito D, Schloss MJ, Drechsler M, et al. Neutrophils orchestrate post-myocardial infarction healing by polarizing macrophages towards a reparative phenotype. Eur Heart J. 2017; 38:187–197.


37. Jeong HY, Kang WS, Hong MH, Jeong HC, Shin MG, Jeong MH, et al. 5-Azacytidine modulates interferon regulatory factor 1 in macrophages to exert a cardioprotective effect. Sci Rep. 2015; 5:15768.


38. Kim YS, Kang WS, Kwon JS, Hong MH, Jeong HY, Jeong HC, et al. Protective role of 5-azacytidine on myocardial infarction is associated with modulation of macrophage phenotype and inhibition of fibrosis. J Cell Mol Med. 2014; 18:1018–1027.


39. Kim YS, Jeong HY, Kim AR, Kim WH, Cho H, Um J, et al. Natural product derivative BIO promotes recovery after myocardial infarction via unique modulation of the cardiac microenvironment. Sci Rep. 2016; 6:30726.


40. Sager HB, Hulsmans M, Lavine KJ, Moreira MB, Heidt T, Courties G, et al. Proliferation and recruitment contribute to myocardial macrophage expansion in chronic heart failure. Circ Res. 2016; 119:853–864.


41. Menasché P, Alfieri O, Janssens S, McKenna W, Reichenspurner H, Trinquart L, et al. The Myoblast Autologous Grafting in Ischemic Cardiomyopathy (MAGIC) trial: first randomized placebo-controlled study of myoblast transplantation. Circulation. 2008; 117:1189–1200.


42. Hare JM, Fishman JE, Gerstenblith G, DiFede Velazquez DL, Zambrano JP, Suncion VY, et al. Comparison of allogeneic vs autologous bone marrow–derived mesenchymal stem cells delivered by transendocardial injection in patients with ischemic cardiomyopathy: the POSEIDON randomized trial. JAMA. 2012; 308:2369–2379.


43. Bolli R, Chugh AR, D'Amario D, Loughran JH, Stoddard MF, Ikram S, et al. Cardiac stem cells in patients with ischaemic cardiomyopathy (SCIPIO): initial results of a randomised phase 1 trial. Lancet. 2011; 378:1847–1857.


44. Bartunek J, Behfar A, Dolatabadi D, Vanderheyden M, Ostojic M, Dens J, et al. Cardiopoietic stem cell therapy in heart failure: the C-CURE (Cardiopoietic stem Cell therapy in heart failURE) multicenter randomized trial with lineage-specified biologics. J Am Coll Cardiol. 2013; 61:2329–2338.
45. Makkar RR, Smith RR, Cheng K, Malliaras K, Thomson LE, Berman D, et al. Intracoronary cardiosphere-derived cells for heart regeneration after myocardial infarction (CADUCEUS): a prospective, randomised phase 1 trial. Lancet. 2012; 379:895–904.


46. Heldman AW, DiFede DL, Fishman JE, Zambrano JP, Trachtenberg BH, Karantalis V, et al. Transendocardial mesenchymal stem cells and mononuclear bone marrow cells for ischemic cardiomyopathy: the TAC-HFT randomized trial. JAMA. 2014; 311:62–73.


47. Karantalis V, DiFede DL, Gerstenblith G, Pham S, Symes J, Zambrano JP, et al. Autologous mesenchymal stem cells produce concordant improvements in regional function, tissue perfusion, and fibrotic burden when administered to patients undergoing coronary artery bypass grafting: The Prospective Randomized Study of Mesenchymal Stem Cell Therapy in Patients Undergoing Cardiac Surgery (PROMETHEUS) trial. Circ Res. 2014; 114:1302–1310.


48. Fischer-Rasokat U, Assmus B, Seeger FH, Honold J, Leistner D, Fichtlscherer S, et al. A pilot trial to assess potential effects of selective intracoronary bone marrow-derived progenitor cell infusion in patients with nonischemic dilated cardiomyopathy: final 1-year results of the transplantation of progenitor cells and functional regeneration enhancement pilot trial in patients with nonischemic dilated cardiomyopathy. Circ Heart Fail. 2009; 2:417–423.

