Abstract
Tuberculosis (TB) is one of the leading causes of adult death in the Asia-Pacific Region, including Indonesia. As an infectious disease caused by Mycobacterium tuberculosis (MTB), TB remains a major public health issue especially in developing nations due to the lack of adequate diagnostic testing facilities. Diagnosis of TB has entered an era of molecular detection that provides faster and more cost-effective methods to diagnose and confirm drug resistance in TB cases, meanwhile, diagnosis by conventional culture systems requires several weeks. New advances in the molecular detection of TB, including the faster and simpler nucleic acid amplification test (NAAT) and whole-genome sequencing (WGS), have resulted in a shorter time for diagnosis and, therefore, faster TB treatments. In this review, we explored the current findings on molecular diagnosis of TB and drug-resistant TB to see how this advancement could be integrated into public health systems in order to control TB.
In the Global Tuberculosis Report 2014 published by the World Health Organization (WHO), among the 249,866,000 Indonesian people, it was estimated that the epidemiological burden of tuberculosis (TB) per 100,000 populations was: 272 of prevalence (range 138–450); 183 of incidence (range 164–207); and 64 of mortality (range 14–37).1 Recently, a genotyping study of Mycobacterium tuberculosis (MTB) was performed in Indonesia to reveal the TB molecular epidemiology and showed a high genetic diversity that varied by geographical aspects and found that the Beijing strain was the predominant strain in Indonesia.2
Fast and accurate detection of the pathogen and its drug susceptibility patterns is crucial for treatment initiation and disease control.3 New techniques are available to optimize the culture medium including optimization of the culture system; early growth detection; strict control of oxygen tension; and the utilization of matrix-assisted laser desorption/ionization time-of-flight mass spectrometry (MALDI-TOF-MS) for identification.4 Advancements in molecular methods for MTB detection has shortened the time to diagnosis to a few days, whereas diagnosis by conventional culture systems needs several weeks.56 The majority of molecular tests have been aimed at the detection of MTB specific nucleic acids, both in DNA and RNA, by using amplification techniques such as polymerase chain reaction (PCR), and detection of genes mutation that are related with the resistance to anti-TB drugs by sequencing or nucleic acid hybridization.7 Moreover, the WHO has announced the need for diagnostic options that are a sputum-based replacement test for smear microscopy, a non-sputum-based biomarker test that is resource-adjusted at facilities below microscopy laboratories, a simple initial test for first-contact care providers as a rule-out test, and a fast drug sensitivity test at the microscopy laboratory level.8 In middle- and high-income countries, development continued with innovations in microscopy (for example, light emitting diode [LED] microscopes), MTB culture systems (for example, rapid automated liquid culture systems, like the Becton Dickinson MGIT 960), nucleic acid amplification systems line probe assays and automated systems, such as the Cepheid XpertⓇ MTB/RIF system (Cepheid, Inc., Sunnyvale, CA, USA).9 In the next section, we will discuss the role of the nucleic acid amplification test (NAAT) and whole-genome sequencing (WGS) in the diagnosis of TB, specimens transport medium technology, mycobacterial load detection assays, serological diagnosis of active TB, and molecular diagnostic of multi-drug resistant TB (MDR-TB).
Because the conventional bacteriological diagnosis of TB has several limitations, the NAAT has emerged as a potential alternative.10 The NAAT systems, with rapid turn-around times, facilitate testing and treatment initiation in the same visit and, therefore, loss to follow-up cases can be reduced.11 Most NAAT assays detect the mycobacterial insertion element IS6110 for the identification of the MTB complex organisms.12 NAAT detects MTB ribosomal RNA or DNA directly from sputum specimens, both the acid-fast bacilli (AFB) smear-positive and AFB smear-negative.13 The NAAT showed very high sensitivity in sputum smear-positive patients and around 61 to 76% sensitivity in patients with smear-negative sputum.11 Currently, the NAAT that is endorsed by the WHO is the Xpert/RIF MTB assay.14 The other two NAATs, the Amplified Mycobacterium Tuberculosis Direct (MTD) Test (Gen-Probe, Inc),151617 and Amplicor Mycobacterium tuberculosis Test (Roche Molecular Systems, Inc),181920 have also been approved by the Food and Drug Administration (FDA) for testing respiratory AFB smear-positive specimens.21 Other commercial NAATs are also available, including the loop-mediated isothermal amplification-based MTB detection system,222324 the cross-priming amplification-based TB diagnostic system,2526 and the GenedriveⓇ Mycobacterium tuberculosis iDⓇ.27
In multi-bacillary diseases with a high mycobacterial load, a positive AFB smear with a positive NAAT would indicate active tuberculosis whereas a positive AFB smear with a negative NAAT in the absence of inhibitors would indicate nontuberculous mycobacterial (NTM) disease.28 If the culture was positive in the above case, the physician could consider the patient as a bacteriologically confirmed case of TB.1 A NAAT could determine whether AFB smear-positive patients had TB or not.29 Moreover, if the NAAT result is positive but the AFB smear result is negative, the decision to begin anti-TB treatment would rely on the clinical judgment while awaiting culture results.30 According to Centers for Disease Control (CDC), if the sputum is smear-negative and the NAAT also negative, an additional specimen should be tested with NAAT.31 However, if the culture results detected MTB bacteria growth, then the patient could also be classified as bacteriologically confirmed for having pulmonary TB. Finally, if the AFB smear, the NAAT, and the MTB culture showed negative results, the physician could classify the patients as a clinically diagnosed case of TB or consider another diagnosis. Fig. 1 described the algorithm of the NAAT in the diagnosis of TB that is in line with the recommendation of both the WHO and the CDC.
In locations where the rate of cultures positive for TB is low, it might be more efficient to limit the NAAT to cases with positive smear results; on the other hand, in locations where TB cases are high, a NAAT should be used in cases with negative smear results.32 A meta-analysis that included 125 studies showed that a commercial NAAT alone should not replace conventional tests for diagnosing pulmonary TB.33
The Xpert MTB/RIF assay is an nuclear acid amplification-based test using a cartridge based on the GeneXpert Instrument System.34 The basis of the Xpert MTB/RIF assay is a real-time PCR that can be used to detect DNA sequences specific to the MTB in sputum samples.35 a ingle Xpert MTB/RIF test directly from sputum can detect 99% of smear-positive patients and more than 80% of smear-negative cases.36 According to the WHO in 2013, a Xpert MTB/RIF assay could be used for: an add-on test following microscopic TB examination; a replacement examination for AFB smear microscopy; detection of MTB in both AFB smear-positive and smear-negative culture-positive cases; detection of MTB in pleural in pleural fluid; detection of MTB in lymph node in samples from biopsy or fine-needle aspiration; detection of MTB in gastric fluid; detection of MTB in samples of cerebrospinal fluid; and detection of MTB in tissue samples.14 In 2014, the WHO stated that the Xpert MTB/RIF assay could be used as the initial diagnostic test in all subjects suspected on having pulmonary TB.37
The Xpert MTB/RIF assay detects rifampicin resistance by PCR amplification of the 81-bp fragment of the MTB rpoB gene and subsequent probing of this region for rifampicin resistant-associated mutations and the results can be obtained within 2 hours.3839 The WHO recommends subjects who are at high risk of MDR-TB should always have their sputum checked using the Xpert MTB/RIF test.40 The Xpert MTB/RIF can be used as an initial test and as an add-on test after a negative AFB smear microscopy result.41 The use of Xpert MTB/RIF test has shortened the median time to treatment for AFB smear-negative TB from 56 days (range 39–81 days) to 5 days (range 2–8 days).42 However, Xpert MTB is more expensive than conventional sputum microscopy.43 Nevertheless, in one analysis, if a rapid sputum-based test unit cost is of US$ 2–4, it would be lower to a similar cost of the conventional sputum smear microscopy.44 In order to facilitate access, the Foundation for Innovative New Diagnostics (FIND) has negotiated significant price reductions.45 Nevertheless, implementation of Xpert MTB/RIF would not be able to improve the control of drug-sensitive TB without improvements to the health system, especially as to reducing the initial loss to follow-up and reducing the time to treatment initiation.46
Microbial genomics has allow us to investigate the organisms genetic markers that may impact treatment and infection prognosis.47 Whole-genome sequencing (WGS) is becoming an affordable and accessible method that can identify microevolution within MTB lineages as they are transmitted between hosts.48 Fig. 2 describes the workflow of WGS from specimen input until clinical diagnostic report. There are two classes of sequencers that exist: the first generation sequencer and the second generation (widely known as the next-generation sequencer [NGS]). The first generation sequencer is relatively slow, but has a high throughput and low cost (approximately $65 per bacterial genome). The second generation has a lower throughput, higher cost (approximately $150 per genome in the case of the IlluminaⓇ MiSeq) and is able to sequence multiple genomes in less than a day.49 Table 1 describes the principles of the first generation sequencer and the next-generation sequencer.50515253
The WGS can detect various types of mutations better than the Xpert MTB assay. Moreover, WGS could avoid false positives when a polymorphism in the rifampicin-resistance determining region (RRDR) of rpoB is detected.54 WGS has not been used as a routine diagnostic tool for TB, partly because of the need to culture MTB for several weeks until an adequate amount of DNA can be extracted.55 Culturing slow-growing MTB before DNA extraction is a major time-consuming step in WGS , therefore, workflow to extract DNA from frozen isolates without re-culturing is important.56 There is a reliable and low-cost DNA extraction method from 1 mL of early positive mycobacterial growth indicator tube (MGIT) cultures that is enough for the WGS to identify mycobacterial species and predict antibiotic resistance in clinical samples.57 Recently, an important method that allows WGS without prior specimen culture has been discovered. The method utilizes MTB DNA-specific biotinylated RNA baits to capture full MTB genomes directly from non-cultured sputum samples.58 WGS data can be obtained several weeks before the drug susceptibility test (DST) data is available.59 DNA sequencing could also be used to confirm rifampin resistance from Xpert MTB/RIF.60 However, with the price in the hundreds of dollars for this method, routine WGS for clinical specimens is limited to high-income countries.61
Sputum samples processing at a central diagnostic laboratory requires an efficient and safe system that is not compromised by potential prolonged transport times. Generally, a prolongation of sample storage results in reduced positivity of the target.62 Therefore, transport medium technology plays an important role in the chain of TB diagnosis. The transport medium system consists of an MTB inactivation step and a DNA stabilization step with the goal to preserve the DNA quality and, at the same time, obtain high DNA output. Some of the known transport technologies are the PrimeStoreⓇ-Molecular Transport Medium (PS-MTM)6364 and cetylpyridinium chloride (CPC).65 PS-MTM inactivates the MTB within the specimens and stabilizes both the DNA/RNA at an ambient temperature for further molecular processing and analysis.64 As for the Xpert MTB assay, incubation of sputum with a sample reagent (SR) could maintain the sputum quality up to 72 h without a further decrease in MTB detection.66
Quantification of the bacillary load has an important prognostic role in TB patients.67 There are several assays that can be used to quantify mycobacterial load, such as the BD ProbeTec system,68 and AdvanSure TB/NTM real-time PCR kit.69 Lee and colleagues evaluated the quantitative capability of the AdvanSure TB/NTM real-time PCR kit (LG Life Science, Korea) to determine the cycle threshold (Ct) for the mycobacterial burden.70 A cut-off Ct value of <33.2 best-predicted stain positivity, with a sensitivity of 95.0% and a specificity of 32.0%. These findings suggest the potential use of AdvanSure TB/NTM real-time PCR kits for quantitatively determining mycobacterial burden. Advan-Sure TB/NTM real-time PCR could be used to detect Mycobacterium and distinguish whether it is MTB or the NTM because it used the IS6110 primer for the detection of MTB complex and rpoB gene specific primer and probe for the detection of NTM.71 However, this assay does not determine if there are viable Mycobacterium organisms that truly exist in the specimen.
Serological tests for TB are widely used in developing countries.72 In one study conducted in Indonesia, Senosaputra and colleagues found that plasma levels of anti-α-crystallin (ACR), anti-lipoarabinomannan, anti-trehalose 6,6'-dimycolate, and anti-tubercular-glycolipid antigen antibodies were higher in active TB patients compared to those in the latent TB infection (LTBI) and control subjects.73 In another study, diverse IgG antibody responsiveness was demonstrated against five lipid antigens: cord factor (trehalose 6,6'-dimycolate) (TDM-T), monoacyl phosphatidylinositol dimannoside (Ac-PIM2), trehalose monomycolate isolated from Mycobacterium bovis Bacillus Calmette-Guérin (BCG) (TMM-T), trehalose monomycolate (TMM-M), and GPL-core from Mycobacterium avium complex (MAC).74 However, a systematic reviews of the diagnostic accuracy of serological tests suggest that these tests are inaccurate and imprecise for both pulmonary and extra-pulmonary TB.75 The serological assay of TB is limited in determining whether a person has had a previous TB infection, therefore it cannot inform whether the person has an active TB infection. Moreover, the WHO strongly recommends that serological commercial tests should not be used for the diagnosis of clinical pulmonary and extra-pulmonary TB.76
The increasing incidence of drug-resistant, MDR (resistant to at least rifampicin and isoniazid), and extensively drug-resistant (XDR) (additionally resistant to a fluoroquinolone and kanamycin/amikacin/capreomycin) strains of MTB have been implicated as a major threat to TB control.77 Detection of drug resistance to both first- and second-line anti-TB drugs is a key component of TB control programs.78 Knowledge of the full drug susceptibility profile would enable customized treatment to increase efficacy and avoid exposure to ineffective toxic drugs.79 Conventional phenotypic DST requires a minimum of 2 weeks: one week for the initial detection of microbial growth and another week to assess critical concentrations of first-line anti-TB drugs.80 Obviously, there is an urgent demand for new, rapid, and accurate DST methods.81 A large, multinational field trial, to compare the performance of the Line Probe Assay (LPA), PyrosequencingⓇ (PSQ), and Microscopic Observation of Drug Susceptibility (MODS) and compared to the BACTEC MGIT960 as reference standard to detect MDR/XDR-TB directly from patient sputum samples was performed, and the results indicated that mean time-to-result was 1.1 days for LPA and PSQ, 14.3 days for MODS, and 24.7 days for MGIT.82
LPAs are available in several methods, such as the AID LPA (AID Diagnostika, Germany),83 the Hain LPA (Hain Lifescience, Germany),84 the INNO LPA (Innogenetics, Belgium),85 the GenoType MTBDRplus,8687 and the GenoType MTBDRsl.88 The INNO LPA and GenoType MTBDRplus have been assessed and validated by the WHO in their Expert Group Report.89 The AID LPA was designed to detect the most prevalent mutations that confer resistance to rifampin, isoniazid, streptomycin, capreomycin, amikacin, ethambutol, and fluoroquinolones.90 Meanwhile, a comparison study suggested that MTBDRplus LPA was superior compared to Xpert MTB/RIF in the detection of rifampin-monoresistant MTB in terms of sensitivity.91 Table 2 summarizes the molecular diagnostic of mono-resistant and MDR-TB detection.779293949596979899100101102103 Based on the list, different methods could explore different resistance-associated mutations, therefore variation of methods allows us to detect various resistances.
The PSQ method consists of seven PSQ assays for the mutations detection of the genes or promoter regions, which are commonly responsible for resistance to the first line and second line drugs.104105 In one study, the correlations between the PSQ results and the phenotypic DST results were 98.7% for rifampin, 94.3% for isoniazid, 99.2% for capreomycin, 99.2% for amikacin, 96.4% for kanamycin, and 97.6% for quinolones (levofloxacin, ofloxacin, or moxifloxacin).106
GenoType MTBDRsl (MTBDRsl) is the only molecular test that is commercially-available for detecting resistance to the fluoroquinolones (levofloxacin, ofloxacin, and moxifloxacin) and the second-line injectable drugs (kanamycin, capreomycin, and amikacin).107 MTBDRsl detects rrs and gyrA genes mutations that confers resistance to injectable antibiotics and fluoroquinolones,, respectively.108 Moreover, MTBDRsl also capable of detecting embB mutations that confer resistance to ethambuthol.109 The sensitivity and specificity of the MTBDRsl test were as follows: 87% and 96%, respectively, for fluoroquinolones; 77% and 100%, respectively, for kanamycin; 80% and 98%, respectively, for capreomycin; 100% and 100%, respectively, for amikacin; and 57% and 92%, respectively, for ethambutol.110 In one meta-analysis, it was reported that MTBDRsl showed good accuracy for detecting drug resistance to fluoroquinolones, capreomycin, and amikacin, but it may not be an appropriate choice for ethambutol and kanamycin.111 However, despite the advances in the resistance detection tools, key challenges still exist including difficulties in documenting the impact on programmatic performance, long-term financial support, and a limited number of scientific publications.112
More rapid and appropriate TB detection and treatment leads to reduced hospitalization and complications, as well as avoiding unnecessary treatment and isolation of false-positive cases.113 The molecular diagnostic testing for active pulmonary TB is promising for the future and should be encouraged in a way that could improve population health.114 Consideration is given to the requirements of future diagnostic tests and how these should be evaluated for their diagnostic accuracy and operational feasibility, and ultimately their clinical impact.115
Figures and Tables
FIG. 1
The role of nucleic acid amplification test (NAAT) in the diagnosis algorithm of TB. TB: tuberculosis, NAAT: nucleic acid amplification test, NTM: nontuberculous mycobacterium.
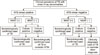
References
1. World Health Organization. Global tuberculosis report 2014. Geneva: World Health Organization;2014.
2. Lisdawati V, Puspandari N, Rif'ati L, Soekarno T, M M, K S, et al. Molecular epidemiology study of Mycobacterium tuberculosis and its susceptibility to anti-tuberculosis drugs in Indonesia. BMC Infect Dis. 2015; 15:366.


3. Engström A. Fighting an old disease with modern tools: characteristics and molecular detection methods of drug-resistant Mycobacterium tuberculosis. Infect Dis (Lond). 2016; 48:1–17.


4. Ghodbane R, Raoult D, Drancourt M. Dramatic reduction of culture time of Mycobacterium tuberculosis. Sci Rep. 2014; 4:4236.


5. Cho WH, Won EJ, Choi HJ, Kee SJ, Shin JH, Ryang DW, et al. Comparison of AdvanSure TB/NTM PCR and COBAS TaqMan MTB PCR for detection of mycobacterium tuberculosis complex in routine clinical practice. Ann Lab Med. 2015; 35:356–361.


6. Huggett JF, McHugh TD, Zumla A. Tuberculosis: amplification- based clinical diagnostic techniques. Int J Biochem Cell Biol. 2003; 35:1407–1412.
7. Balasingham SV, Davidsen T, Szpinda I, Frye SA, Tønjum T. Molecular diagnostics in tuberculosis: basis and implications for therapy. Mol Diagn Ther. 2009; 13:137–151.
8. Denkinger CM, Kik SV, Cirillo DM, Casenghi M, Shinnick T, Weyer K, et al. Defining the needs for next generation assays for tuberculosis. J Infect Dis. 2015; 211:Suppl 2. S29–S38.


9. Drobniewski F, Nikolayevskyy V, Maxeiner H, Balabanova Y, Casali N, Kontsevaya I, et al. Rapid diagnostics of tuberculosis and drug resistance in the industrialized world: clinical and public health benefits and barriers to implementation. BMC Med. 2013; 11:190.


10. Qin L, Zheng R, Fan C, Cai J, Liu Z, Wang J, et al. Identification and evaluation of a new nucleic acid amplification test target for specific detection of Mycobacterium tuberculosis. Clin Chem Lab Med. 2010; 48:1501–1505.


11. Niemz A, Boyle DS. Nucleic acid testing for tuberculosis at the point-of-care in high-burden countries. Expert Rev Mol Diagn. 2012; 12:687–701.


12. Tiwari S, Nataraj G, Kanade S, Mehta P. Diagnosis of pediatric pulmonary tuberculosis with special reference to polymerase chain reaction based nucleic acid amplification test. Int J Mycobacteriol. 2015; 4:48–53.


13. Sia IG, Wieland ML. Current concepts in the management of tuberculosis. Mayo Clin Proc. 2011; 86:348–361.


14. Automated real-time nucleic acid amplification technology for rapid and simultaneous detection of tuberculosis and rifampicin resistance: xpert MTB/RIF assay for the diagnosis of pulmonary and extrapulmonary TB in adults and children. WHO Global TB Programme. Geneva: World Health Organization;2013.
15. Coll P, Garrigó C, Moreno C, Martì N. Routine use of Gen-Probe Amplified Mycobacterium Tuberculosis Direct (MTD) test for detection of Mycobacterium tuberculosis with smear-positive and smear-negative specimens. Int J Tuberc Lung Dis. 2003; 7:886–891.
16. Kunduracğlu A, Karasu I, Biçmen C, Ozsöz A, Erbaycu AE. Comparison of the performances of MTD Gene-Probe® test, BACTEC 960™ system and Löwenstein-Jensen culture methods in the diagnosis of smear-negative tuberculosis cases. Mikrobiyol Bul. 2013; 47:417–431.


17. Papaventsis D, Ioannidis P, Karabela S, Nikolaou S, Syridou G, Marinou I, et al. Impact of the Gen-Probe Amplified MTD® Test on tuberculosis diagnosis in children. Int J Tuberc Lung Dis. 2012; 16:384–390.


18. Carpentier E, Drouillard B, Dailloux M, Moinard D, Vallee E, Dutilh B, et al. Diagnosis of tuberculosis by Amplicor Mycobacterium tuberculosis test: a multicenter study. J Clin Microbiol. 1995; 33:3106–3110.


19. Bergmann JS, Woods GL. Clinical evaluation of the Roche AMPLICOR PCR Mycobacterium tuberculosis test for detection of M. tuberculosis in respiratory specimens. J Clin Microbiol. 1996; 34:1083–1085.


20. Devallois A, Legrand E, Rastogi N. Evaluation of Amplicor MTB test as adjunct to smears and culture for direct detection of Mycobacterium tuberculosis in the French Caribbean. J Clin Microbiol. 1996; 34:1065–1068.


21. Woods GL. Molecular methods in the detection and identification of mycobacterial infections. Arch Pathol Lab Med. 1999; 123:1002–1006.


22. Iwamoto T, Sonobe T, Hayashi K. Loop-mediated isothermal amplification for direct detection of Mycobacterium tuberculosis complex, M. avium, and M. intracellulare in sputum samples. J Clin Microbiol. 2003; 41:2616–2622.


23. Neonakis IK, Spandidos DA, Petinaki E. Use of loop-mediated isothermal amplification of DNA for the rapid detection of Mycobacterium tuberculosis in clinical specimens. Eur J Clin Microbiol Infect Dis. 2011; 30:937–942.


24. Mitarai S, Okumura M, Toyota E, Yoshiyama T, Aono A, Sejimo A, et al. Evaluation of a simple loop-mediated isothermal amplification test kit for the diagnosis of tuberculosis. Int J Tuberc Lung Dis. 2011; 15:1211–1217.


25. Fang R, Li X, Hu L, You Q, Li J, Wu J, et al. Cross-priming amplification for rapid detection of Mycobacterium tuberculosis in sputum specimens. J Clin Microbiol. 2009; 47:845–847.


26. Ou X, Song Y, Zhao B, Li Q, Xia H, Zhou Y, et al. A multicenter study of cross-priming amplification for tuberculosis diagnosis at peripheral level in China. Tuberculosis (Edinb). 2014; 94:428–433.


27. Castan P, de Pablo A, Fern . dez-Romero N, Rubio JM, Cobb BD, Mingorance J, et al. Point-of-care system for detection of Mycobacterium tuberculosis and rifampin resistance in sputum samples. J Clin Microbiol. 2014; 52:502–507.


28. Cheng VC, Yew WW, Yuen KY. Molecular diagnostics in tuberculosis. Eur J Clin Microbiol Infect Dis. 2005; 24:711–720.


29. Laraque F, Griggs A, Slopen M, Munsiff SS. Performance of nucleic acid amplification tests for diagnosis of tuberculosis in a large urban setting. Clin Infect Dis. 2009; 49:46–54.


30. Centers for Disease Control and Prevention (CDC). Updated guidelines for the use of nucleic acid amplification tests in the diagnosis of tuberculosis. MMWR Morb Mortal Wkly Rep. 2009; 58:7–10.
31. Centers for Disease Control and Prevention (CDC). Update: Nucleic acid amplification tests for tuberculosis. MMWR Morb Mortal Wkly Rep. 2000; 49:593–594.
32. Dylewski J. Nucleic Acid amplification testing for the diagnosis of tuberculosis: not for all. Clin Infect Dis. 2009; 49:1456–1457.


33. Ling DI, Flores LL, Riley LW, Pai M. Commercial nucleic-acid amplification tests for diagnosis of pulmonary tuberculosis in respiratory specimens: meta-analysis and meta-regression. PLoS One. 2008; 3:e1536.


34. Centers for Disease Control and Prevention (CDC). Availability of an assay for detecting Mycobacterium tuberculosis, including rifampin-resistant strains, and considerations for its use - United States, 2013. MMWR Morb Mortal Wkly Rep. 2013; 62:821–827.
35. Friedrich SO, Rachow A, Saathoff E, Singh K, Mangu CD, Dawson R, et al. Assessment of the sensitivity and specificity of Xpert MTB/RIF assay as an early sputum biomarker of response to tuberculosis treatment. Lancet Respir Med. 2013; 1:462–470.


36. World Health Organization. Automated real-time nucleic acid amplification technology for rapid and simultaneous detection of tuberculosis and rifampicin resistance: Xpert MTB/RIF system. Geneva: World Health Organization;2011.
37. WHO Global TB Programme. Xpert MTB/RIF implementation manual: technical and operational 'how-to': practical considerations. Geneva: World Health Organization;2014.
38. Zeka AN, Tasbakan S, Cavusoglu C. Evaluation of the Gene- Xpert MTB/RIF assay for rapid diagnosis of tuberculosis and detection of rifampin resistance in pulmonary and extrapulmonary specimens. J Clin Microbiol. 2011; 49:4138–4141.


39. Boehme CC, Nabeta P, Hillemann D, Nicol MP, Shenai S, Krapp F, et al. Rapid molecular detection of tuberculosis and rifampin resistance. N Engl J Med. 2010; 363:1005–1015.


40. World Health Organization. Systematic screening for active tuberculosis: principles and recommendations. Geneva: World Health Organization;2013.
41. Steingart KR, Schiller I, Horne DJ, Pai M, Boehme CC, Dendukuri N. Xpert® MTB/RIF assay for pulmonary tuberculosis and rifampicin resistance in adults. Cochrane Database Syst Rev. 2014; (1):CD009593.


42. Boehme CC, Nicol MP, Nabeta P, Michael JS, Gotuzzo E, Tahirli R, et al. Feasibility, diagnostic accuracy, and effectiveness of decentralised use of the Xpert MTB/RIF test for diagnosis of tuberculosis and multidrug resistance: a multicentre implementation study. Lancet. 2011; 377:1495–1505.


43. Steingart KR, Sohn H, Schiller I, Kloda LA, Boehme CC, Pai M, et al. Xpert® MTB/RIF assay for pulmonary tuberculosis and resistance in adults. Cochrane Database Syst Rev. 2013; (1):CD009593.
44. Pantoja A, Kik SV, Denkinger CM. Costs of novel tuberculosis diagnostics--will countries be able to afford it? J Infect Dis. 2015; 211:Suppl 2. S67–S77.


45. Bodmer T, Ströhle A. Diagnosing pulmonary tuberculosis with the Xpert MTB/RIF test. J Vis Exp. 2012; (62):e3547.


46. Churchyard GJ, Stevens WS, Mametja LD, McCarthy KM, Chihota V, Nicol MP, et al. Xpert MTB/RIF versus sputum microscopy as the initial diagnostic test for tuberculosis: a cluster- randomised trial embedded in South African roll-out of Xpert MTB/RIF. Lancet Glob Health. 2015; 3:e450–e457.
47. Kwong JC, McCallum N, Sintchenko V, Howden BP. Whole genome sequencing in clinical and public health microbiology. Pathology. 2015; 47:199–210.


48. Walker TM, Ip CL, Harrell RH, Evans JT, Kapatai G, Dedicoat MJ, et al. Whole-genome sequencing to delineate Mycobacterium tuberculosis outbreaks: a retrospective observational study. Lancet Infect Dis. 2013; 13:137–146.


49. Köser CU, Ellington MJ, Peacock SJ. Whole-genome sequencing to control antimicrobial resistance. Trends Genet. 2014; 30:401–407.


51. Strausberg RL, Levy S, Rogers YH. Emerging DNA sequencing technologies for human genomic medicine. Drug Discov Today. 2008; 13:569–577.


52. Rizzo JM, Buck MJ. Key principles and clinical applications of "next-generation" DNA sequencing. Cancer Prev Res (Phila). 2012; 5:887–900.


54. Witney AA, Cosgrove CA, Arnold A, Hinds J, Stoker NG, Butcher PD. Clinical use of whole genome sequencing for Mycobacterium tuberculosis. BMC Med. 2016; 14:46.


55. Köser CU, Bryant JM, Becq J, Török ME, Ellington MJ, Marti-Renom MA, et al. Whole-genome sequencing for rapid susceptibility testing of M. tuberculosis. N Engl J Med. 2013; 369:290–292.


56. Bjorn-Mortensen K, Zallet J, Lillebaek T, Andersen AB, Niemann S, Rasmussen EM, et al. Direct DNA Extraction from Mycobacterium tuberculosis Frozen Stocks as a Reculture-Independent Approach to Whole-Genome Sequencing. J Clin Microbiol. 2015; 53:2716–2719.


57. Votintseva AA, Pankhurst LJ, Anson LW, Morgan MR, Gascoyne-Binzi D, Walker TM, et al. Mycobacterial DNA extraction for whole-genome sequencing from early positive liquid (MGIT) cultures. J Clin Microbiol. 2015; 53:1137–1143.


58. Brown AC, Bryant JM, Einer-Jensen K, Holdstock J, Houniet DT, Chan JZ, et al. Rapid whole-genome sequencing of mycobacterium tuberculosis isolates directly from clinical samples. J Clin Microbiol. 2015; 53:2230–2237.


59. Witney AA, Gould KA, Arnold A, Coleman D, Delgado R, Dhillon J, et al. Clinical application of whole-genome sequencing to inform treatment for multidrug-resistant tuberculosis cases. J Clin Microbiol. 2015; 53:1473–1483.


60. McAlister AJ, Driscoll J, Metchock B. DNA sequencing for confirmation of rifampin resistance detected by Cepheid Xpert MTB/RIF assay. J Clin Microbiol. 2015; 53:1752–1753.


61. Takiff HE, Feo O. Clinical value of whole-genome sequencing of Mycobacterium tuberculosis. Lancet Infect Dis. 2015; 15:1077–1090.


62. Hasan MR, Tan R, Al-Rawahi GN, Thomas E, Tilley P. Short-term stability of pathogen-specific nucleic acid targets in clinical samples. J Clin Microbiol. 2012; 50:4147–4150.


63. Omar SV, Peters RP, Ismail NA, Dreyer AW, Said HM, Gwala T, et al. Laboratory evaluation of a specimen transport medium for downstream molecular processing of sputum samples to detect Mycobacterium tuberculosis. J Microbiol Methods. 2015; 117:57–63.


64. Daum LT, Peters RP, Fourie PB, Jonkman K, Worthy SA, Rodriguez JD, et al. Molecular detection of Mycobacterium tuberculosis from sputum transported in PrimeStore(®) from rural settings. Int J Tuberc Lung Dis. 2015; 19:552–557.


65. Das D, Dwibedi B, Kar SK. Transportation of sputum samples in cetylpyridinium chloride for drug resistance studies from remote areas of Odisha, India. J Infect Dev Ctries. 2014; 8:1059–1062.


66. Banada PP, Sivasubramani SK, Blakemore R, Boehme C, Perkins MD, Fennelly K, et al. Containment of bioaerosol infection risk by the Xpert MTB/RIF assay and its applicability to point-of-care settings. J Clin Microbiol. 2010; 48:3551–3557.


67. Alnimr AM, Hassan MI. Potential of two nucleic acid amplification assays for quantifying mycobacterial load in respiratory and non-respiratory specimens: a prospective study. Diagn Microbiol Infect Dis. 2014; 78:237–241.


68. Wang JY, Lee LN, Lai HC, Hsu HL, Jan IS, Yu CJ, et al. Performance assessment of the Capilia TB assay and the BD ProbeTec ET system for rapid culture confirmation of Mycobacterium tuberculosis. Diagn Microbiol Infect Dis. 2007; 59:395–399.


69. Kim YJ, Park MY, Kim SY, Cho SA, Hwang SH, Kim HH, et al. Evaluation of the performances of AdvanSure TB/NTM real time PCR kit for detection of mycobacteria in respiratory specimens. Korean J Lab Med. 2008; 28:34–38.


70. Lee H, Park KG, Lee G, Park J, Park YG, Park YJ. Assessment of the quantitative ability of AdvanSure TB/NTM real-time PCR in respiratory specimens by comparison with phenotypic methods. Ann Lab Med. 2014; 34:51–55.


71. Choe W, Kim E, Park SY, Chae JD. Performance evaluation of anyplex plus MTB/NTM and AdvanSure TB/NTM for the detection of Mycobacterium tuberculosis and nontuberculous mycobacteria. Ann Clin Microbiol. 2015; 18:44–51.


72. Steingart KR, Flores LL, Dendukuri N, Schiller I, Laal S, Ramsay A, et al. Commercial serological tests for the diagnosis of active pulmonary and extrapulmonary tuberculosis: an updated systematic review and meta-analysis. PLoS Med. 2011; 8:e1001062.


73. Senoputra MA, Shiratori B, Hasibuan FM, Koesoemadinata RC, Apriani L, Ashino Y, et al. Diagnostic value of antibody responses to multiple antigens from Mycobacterium tuberculosis in active and latent tuberculosis. Diagn Microbiol Infect Dis. 2015; 83:278–285.


74. Fujita Y, Doi T, Maekura R, Ito M, Yano I. Differences in serological responses to specific glycopeptidolipid-core and common lipid antigens in patients with pulmonary disease due to Mycobacterium tuberculosis and Mycobacterium avium complex. J Med Microbiol. 2006; 55:189–199.


75. Steingart KR, Ramsay A, Dowdy DW, Pai M. Serological tests for the diagnosis of active tuberculosis: relevance for India. Indian J Med Res. 2012; 135:695–702.
76. World Health Organization. Tuberculosis serodiagnostic tests policy statement 2011. Geneva: World Health Organization;2013.
77. Ahmad S, Mokaddas E. Current status and future trends in the diagnosis and treatment of drug-susceptible and multidrug-resistant tuberculosis. J Infect Public Health. 2014; 7:75–91.


78. Lee SH, Choi HB, Yu SY, Chang UJ, Kim CK, Kim HJ. Detection of first-line anti-tuberculosis drug resistance mutations by allele- specific primer extension on a microsphere-based platform. Ann Lab Med. 2015; 35:487–493.


79. Coll F, McNerney R, Preston MD, Guerra-Assunção JA, Warry A, Hill-Cawthorne G, et al. Rapid determination of anti-tuberculosis drug resistance from whole-genome sequences. Genome Med. 2015; 7:51.


80. Outhred AC, Jelfs P, Suliman B, Hill-Cawthorne GA, Crawford AB, Marais BJ, et al. Added value of whole-genome sequencing for management of highly drug-resistant TB. J Antimicrob Chemother. 2015; 70:1198–1202.


81. Xu HB, Jiang RH, Sha W, Li L, Xiao HP. PCR-single-strand conformational polymorphism method for rapid detection of rifampin- resistant Mycobacterium tuberculosis: systematic review and meta-analysis. J Clin Microbiol. 2010; 48:3635–3640.


82. Catanzaro A, Rodwell TC, Catanzaro DG, Garfein RS, Jackson RL, Seifert M, et al. Performance comparison of three rapid tests for the diagnosis of drug-resistant tuberculosis. PLoS One. 2015; 10:e0136861.


83. Molina-Moya B, Lacoma A, Prat C, Diaz J, Dudnyk A, Haba L, et al. AID TB resistance line probe assay for rapid detection of resistant Mycobacterium tuberculosis in clinical samples. J Infect. 2015; 70:400–408.


84. Li Q, Dong HY, Pang Y, Xia H, Ou XC, Zhang ZY, et al. Multicenter evaluation of the molecular line probe assay for multidrug resistant mycobacterium tuberculosis detection in China. Biomed Environ Sci. 2015; 28:464–467.
85. Viveiros M, Leandro C, Rodrigues L, Almeida J, Bettencourt R, Couto I, et al. Direct application of the INNO-LiPA Rif.TB line-probe assay for rapid identification of Mycobacterium tuberculosis complex strains and detection of rifampin resistance in 360 smear-positive respiratory specimens from an area of high incidence of multidrug-resistant tuberculosis. J Clin Microbiol. 2005; 43:4880–4884.


86. Yadav RN, Singh BK, Sharma SK, Sharma R, Soneja M, Sreenivas V, et al. Comparative evaluation of GenoType MTBDRplus line probe assay with solid culture method in early diagnosis of multidrug resistant tuberculosis (MDR-TB) at a tertiary care centre in India. PLoS One. 2013; 8:e72036.


87. Raizada N, Sachdeva KS, Chauhan DS, Malhotra B, Reddy K, Dave PV, et al. A multi-site validation in India of the line probe assay for the rapid diagnosis of multi-drug resistant tuberculosis directly from sputum specimens. PLoS One. 2014; 9:e88626.


88. Kiet VS, Lan NT, An DD, Dung NH, Hoa DV, van Vinh, et al. Evaluation of the MTBDRsl test for detection of second- line-drug resistance in Mycobacterium tuberculosis. J Clin Microbiol. 2010; 48:2934–2939.


89. Molecular line probe assays for rapid screening of patients at risk of multi-drug resistant tuberculosis. World Health Organization Policy Statement. Geneva, Switzerland: World Health Organization;2008.
90. Ritter C, Lucke K, Sirgel FA, Warren RW, van Helden PD, Böttger EC, et al. Evaluation of the AID TB resistance line probe assay for rapid detection of genetic alterations associated with drug resistance in Mycobacterium tuberculosis strains. J Clin Microbiol. 2014; 52:940–946.


91. Rufai SB, Kumar P, Singh A, Prajapati S, Balooni V, Singh S. Comparison of Xpert MTB/RIF with line probe assay for detection of rifampin-monoresistant Mycobacterium tuberculosis. J Clin Microbiol. 2014; 52:1846–1852.


92. Yin X, Zheng L, Liu Q, Lin L, Hu X, Hu Y, et al. High-resolution melting curve analysis for rapid detection of rifampin resistance in Mycobacterium tuberculosis: a meta-analysis. J Clin Microbiol. 2013; 51:3294–3299.


93. Chakravorty S, Kothari H, Aladegbami B, Cho EJ, Lee JS, Roh SS, et al. Rapid, high-throughput detection of rifampin resistance and heteroresistance in Mycobacterium tuberculosis by use of sloppy molecular beacon melting temperature coding. J Clin Microbiol. 2012; 50:2194–2202.


94. Martin A, Panaiotov S, Portaels F, Hoffner S, Palomino JC, Angeby K. The nitrate reductase assay for the rapid detection of isoniazid and rifampicin resistance in Mycobacterium tuberculosis: a systematic review and meta-analysis. J Antimicrob Chemother. 2008; 62:56–64.


95. Coban AY, Deveci A, Sunter AT, Martin A. Nitrate reductase assay for rapid detection of isoniazid, rifampin, ethambutol, and streptomycin resistance in Mycobacterium tuberculosis: a systematic review and meta-analysis. J Clin Microbiol. 2014; 52:15–19.


96. Gonzalez L, Sanchez R, Murcia MI. The usefulness of the nitrate reductase assay for detecting drug-resistant Mycobacterium tuberculosis. Biomedica. 2014; 34:Suppl 1. 232–238.
97. Martin A, Portaels F, Palomino JC. Colorimetric redox-indicator methods for the rapid detection of multidrug resistance in Mycobacterium tuberculosis: a systematic review and meta-analysis. J Antimicrob Chemother. 2007; 59:175–183.


98. Coban AY, Akbal AU, Uzun M, Durupinar B. Evaluation of four colourimetric susceptibility tests for the rapid detection of multidrug- resistant Mycobacterium tuberculosis isolates. Mem Inst Oswaldo Cruz. 2015; 110:649–654.


99. Cheng X, Zhang J, Yang L, Xu X, Liu J, Yu W, et al. A new Multi-PCR-SSCP assay for simultaneous detection of isoniazid and rifampin resistance in Mycobacterium tuberculosis. J Microbiol Methods. 2007; 70:301–305.


100. Cabibbe AM, Miotto P, Moure R, Alcaide F, Feuerriegel S, Pozzi G, et al. Lab-on-chip-based platform for fast molecular diagnosis of multidrug-resistant tuberculosis. J Clin Microbiol. 2015; 53:3876–3880.


101. Pérez-Osorio AC, Boyle DS, Ingham ZK, Ostash A, Gautom RK, Colombel C, et al. Rapid identification of mycobacteria and drug-resistant Mycobacterium tuberculosis by use of a single multiplex PCR and DNA sequencing. J Clin Microbiol. 2012; 50:326–336.


102. Chia BS, Lanzas F, Rifat D, Herrera A, Kim EY, Sailer C, et al. Use of multiplex allele-specific polymerase chain reaction (MAS-PCR) to detect multidrug-resistant tuberculosis in Panama. PLoS One. 2012; 7:e40456.


103. Choi JH, Lee KW, Kang HR, Hwang YI, Jang S, Kim DG, et al. Clinical efficacy of direct DNA sequencing analysis on sputum specimens for early detection of drug-resistant Mycobacterium tuberculosis in a clinical setting. Chest. 2010; 137:393–400.


104. Engström A, Juréen P. Detection of Drug-Resistant Mycobacterium tuberculosis. Methods Mol Biol. 2015; 1315:349–362.


105. Lacoma A, Molina-Moya B, Prat C, Pimkina E, Diaz J, Dudnyk A, et al. Pyrosequencing for rapid detection of Mycobacterium tuberculosis second-line drugs and ethambutol resistance. Diagn Microbiol Infect Dis. 2015; 83:263–269.


106. Lin SY, Rodwell TC, Victor TC, Rider EC, Pham L, Catanzaro A, et al. Pyrosequencing for rapid detection of extensively drug-resistant Mycobacterium tuberculosis in clinical isolates and clinical specimens. J Clin Microbiol. 2014; 52:475–482.


107. Theron G, Peter J, Richardson M, Barnard M, Donegan S, Warren R, et al. The diagnostic accuracy of the GenoType(®) MTBDRsl assay for the detection of resistance to second-line anti- tuberculosis drugs. Cochrane Database Syst Rev. 2014; (10):CD010705.


108. Barnard M, Warren R, Gey Van, van Helden P, Bosman M, Streicher E, et al. GenoType MTBDRsl line probe assay shortens time to diagnosis of extensively drug-resistant tuberculosis in a high-throughput diagnostic laboratory. Am J Respir Crit Care Med. 2012; 186:1298–1305.


109. Lacoma A, Garcìa-Sierra N, Prat C, Maldonado J, Ruiz-Manzano J, Haba L, et al. GenoType MTBDRsl for molecular detection of second-line-drug and ethambutol resistance in Mycobacterium tuberculosis strains and clinical samples. J Clin Microbiol. 2012; 50:30–36.


110. Brossier F, Veziris N, Aubry A, Jarlier V, Sougakoff W. Detection by GenoType MTBDRsl test of complex mechanisms of resistance to second-line drugs and ethambutol in multidrug-resistant Mycobacterium tuberculosis complex isolates. J Clin Microbiol. 2010; 48:1683–1689.


111. Feng Y, Liu S, Wang Q, Wang L, Tang S, Wang J, et al. Rapid diagnosis of drug resistance to fluoroquinolones, amikacin, capreomycin, kanamycin and ethambutol using genotype MTBDRsl assay: a meta-analysis. PLoS One. 2013; 8:e55292.


112. Mahendradhata Y, Probandari A, Widjanarko B, Riono P, Mustikawati D, Tiemersma EW, et al. Embedding operational research into national disease control programme: lessons from 10 years of experience in Indonesia. Glob Health Action. 2014; 7:25412.


113. Kaul KL. Molecular detection of Mycobacterium tuberculosis: impact on patient care. Clin Chem. 2001; 47:1553–1558.

